用于监测人类健康信息的柔性和可穿戴电子设备的迷你评论
摘要
在过去的几十年中,可穿戴电子产品在医疗保健领域的应用潜力引起了人们的极大兴趣。基于亲肤柔软弹性材料的柔性可穿戴设备可紧贴人体皮肤表面,提取并分析腕脉搏、体温、血糖等一系列重要健康信息,帮助人体健康。病人保持身体健康。在这里,我们概述了用于监测人体健康信息的最常见的可穿戴电子设备类型,包括力传感器、温度传感器、生理生化传感器和多功能传感器。审查了它们的一般工作原理和结构创新。然后,我们讨论了使可穿戴传感器更适用于现实生活的两个功能模块——自供电模块和信号处理模块。还提出了开发可穿戴电子产品以监测人体健康信息的挑战和未来研究方向。
介绍
20世纪50年代以来,硅基半导体技术的兴起极大地推动了信息技术产业的发展,使人们的生活发生了翻天覆地的变化。然而,随着世界信息化进程的加快和物联网(IoT)的发展,传统的高杨氏模量硅基电子产品正面临着新的挑战。在过去的几十年里,柔性和可穿戴电子产品引起了越来越多的兴趣,并成为科学界的热门话题。与刚性硅基电子器件相比,柔性电子器件具有许多独特的优越特性,如高柔韧性、超轻重量和保形性,使柔性可穿戴电子器件得到更广泛的应用。
特别是,人们对用于定期和连续监测人类健康信息的柔性和可穿戴医疗设备越来越感兴趣。正在发明新设备以尽可能舒适地持续监测生命体征。这些可穿戴医疗电子设备只需将它们贴在人体表面,即可无创实时测量心率、脉搏、体温、血糖等各种健康指标。实时监测生命体征,可以在个人身体健康指标异常时提醒用户和医疗保健提供者进一步就医,避免错过最佳治疗时间的情况。此外,柔性电子可以随意变形并以极高的灵敏度检测各种信号,因此可用于人造电子皮肤、运动检测、远程医疗和家庭保健。毫无疑问,下一代柔性可穿戴电子产品将引领人类生活方式的一场革命。
过去几年,可穿戴电子产品的生产和开发投入了大量精力,在新材料、新工艺和传感机制方面取得了令人振奋的进步。如图 1 所示,这篇综述主要关注用于监测人类健康信息的可穿戴电子设备的开发,并通过引用一些成功的例子来讨论它们的一般工作原理。在第 2 节中,我们介绍了用于测量由血液运动和人类活动引起的体表微应变的力传感器。特别是那些微结构应力或压力传感器具有超高灵敏度,可用于检测脉搏[1, 2]、声音[3]和人体运动[4]。在第 3 节中,回顾了用于检测和映射皮肤温度的温度传感器。对于温度传感器,我们专注于一些解决方案,以增强可拉伸性和从温度效应中解耦应变干扰。除了物理信号,人体的正常活动也会产生生物信号。用于监测生理生物标志物的生理生化传感器在第 4 节中描述。在第 5 节中,我们描述了一些集成多个敏感元件以执行同时多通道信号检测的多功能传感器。为了真正实现可穿戴电子设备的独立运行,需要一些实用的功能模块,如自供电组件和数据处理模块,在第 6 节中进行了简要回顾。 最后,我们总结了用于监控人体的柔性可穿戴电子设备的发展近年来的健康信息,展望了柔性可穿戴电子产品在人体健康信息监测中的应用前景。
<图片>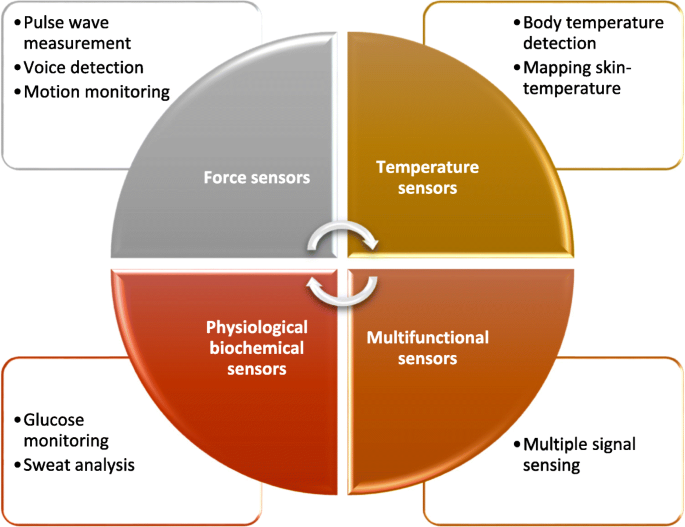
用于监测人体健康信息的可穿戴电子产品的最新发展可视化总结
图>柔性力传感器
力传感器是一种传感装置,可以检测张力、压力、扭矩、应力、应变等机械力的值,并将其转换为电信号。人体有规律的生理活动所产生的各种生理刺激中包含着许多重要的健康信息,例如心率、肌肉运动、呼吸频率、血压等。传统的力传感器大多采用金属和半导体材料制成,体积庞大且笨重,而且由于其便携性和灵活性受到极大限制,不适用于监测人体生命体征的可穿戴电子产品。与传统的力传感器相比,使用塑料和弹性体基板的柔性力传感器具有一系列优势,例如更好的生物相容性、拉伸性、透明性、耐磨性和连续检测能力。下面我们将讨论柔性力传感器可分为电阻式传感器、电容式传感器和压电式传感器。
电阻力传感器
电阻式传感器是将外界刺激引起的敏感材料的电阻变化转换为电信号输出的传感器。柔性电阻力传感器的活性材料通常是通过掺入导电填料形成的弹性体复合材料,例如石墨烯 [5, 6]、碳纳米管 (CNT) [7,8,9,10, 11]、金属薄膜、纳米线、粒子 [12,13,14] 和导电聚合物 [15] 合成弹性体(例如,PDMS、PU、SEBS)。传感器的电阻变化主要由以下三个因素引起:(1)敏感元件几何形状的变化[15],(2)纳米颗粒或纳米线之间间隙的变化[5,6,7,8, 9,10,13,14] 和 (3) 不同材料层之间接触电阻的变化 [12, 11]。压阻式传感器由于功耗低、制造工艺简单、应用广泛而受到广泛关注[16]。
利用具有微结构表面的基板提供了一种制造高灵敏度压阻力传感器的有效方法。如图 2a、b 所示,Choong 等人。 [15] 通过使用微锥聚二甲基硅氧烷 (PDMS) 阵列来提高传感器的压力灵敏度,报告了一种灵活的压阻传感器。这项工作证明,使用微金字塔基底可以最大限度地提高由压力或拉伸引起的导电电极的几何变化,显着提高灵敏度(图 2c)。从图 2d 可以看出,传感器对压力具有良好的线性响应。然而,微金字塔结构的制造基于硅模具,其制造工艺复杂且成本高[1, 3]。王等人。 [1] 使用一条精致的丝巾作为模具来制造微图案的 PDMS 基板。在他们的工作中,将独立的单壁碳纳米管 (SWCNTs) 超薄膜转移到微图案表面上,并通过面对面放置两层 SWCNTs/PDMS 薄膜来构建传感器。以蚕丝为模板制备的具有表面微结构的传感器具有灵敏度高、响应时间快、稳定性好、检测限超低、语音识别和脉冲实时检测等优异的传感性能。此外,Su 等人。 [17] 报道了使用含羞草叶具有不规则微区图案的 PDMS 薄膜。魏等人。 [18] 使用磨砂玻璃基板生产微圆顶结构的 PDMS 薄膜。这些努力为制造具有微结构的大面积薄膜基板提供了简单且低成本的方法,并在提高压阻传感器的灵敏度方面取得了良好的效果。固有的微结构柔性材料,例如纸 [4]、纺织品 [19]、植物和植物衍生的生物材料 [20, 21],已经引起了广泛的兴趣以用作基材。陶等人。 [4] 报道了用于检测人类活动的石墨烯/纸基压力传感器。他们将薄纸与氧化石墨烯 (GO) 溶液混合以获得 GO 纸。在干燥炉中加热几个小时后,GO 纸被还原,得到 rGO/纸导电复合材料。纸基传感器在 0-20 kPa 压力范围内的灵敏度随薄纸的层数而变化。八层传感器的最大灵敏度为 17.2 kPa -1 在 0-2 kPa 的范围内。石墨烯/纸基压力传感器在监测手腕脉搏、呼吸、说话和运动状态方面表现出巨大的潜力。此外,杨等人。 [19] 通过在聚酯织物基材上将 GO 片热还原为石墨烯片,制备了一种可穿戴应变传感器。交织结构的织物基底赋予传感器一些特殊的响应特性,包括超高的负电阻应变系数和独特的方向灵敏度。所制备的纺织品应变传感器可以与服装完美结合,实时监测人体脉搏、嘴巴运动、面部表情等运动。
<图片>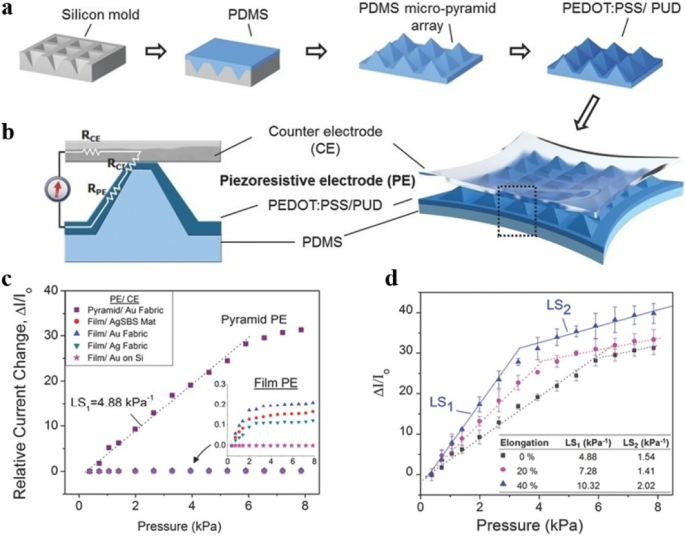
一 微锥PDMS阵列的制作过程。 b 微金字塔结构传感器在外力作用下的传感原理示意图。 c 与非结构化传感器相比,金字塔传感器的灵敏度得到提高。 d 微金字塔传感器在拉伸时的线性压力响应。经参考许可改编。 10. 版权所有 2014 John Wiley and Sons
图>将具有多孔结构的导电材料嵌入弹性体基质中以构建二维或三维导电网络是另一种在电阻力传感器中实现高灵敏度的方法 [7, 22, 23, 19, 24]。外力引起的变形会改变导电材料的空间分布密度,从而改变传感器的电阻。如图 3a 所示,Wang 等人。 [7]通过将向日葵花粉(SFP)微胶囊与多壁碳纳米管(MWCNT)结合制备空心球导电复合材料,然后将它们添加到PDMS中制备MWCNT / PDMS复合膜。通过将这种 MWCNT/PDMS 复合膜夹在两个导电电极之间来制造电子皮肤装置。如图 3b-d 所示,与平面传感器相比,这种由基于花粉的微胶囊引入的空心球结构使传感器具有更高的灵敏度、更快的弛豫时间和非常高的稳定性。当传感器附着在人的手指或人的喉咙上时,它可以同时动态检测压力和应变。李等人。 [23] 介绍了一种通过高温热解过程将薄纸转化为复写纸 (CP) 来构建多孔导电网络的简单方法。图 3e 是碳纸的 SEM 图像。通过简单的真空灌注工艺成功制造了由碳纸和PDMS树脂组成的高灵敏度应变传感器。多孔结构使传感器对施加的应变具有超高灵敏度,几乎比传统金属传感器高一个数量级。如图 3f、3 所示,CP/PDMS 传感器分别通过与腰带和手套的集成,演示了对成人呼吸和人手手势的监测。李等人。 [22]通过使用静电纺丝工艺制造了具有多孔结构的压敏纳米纤维。导电纳米材料(碳纳米管和石墨烯)均匀分散在纳米纤维内部以提高传感能力。由于纳米多孔结构,使用这些复合纳米纤维制成的电阻式压力传感器对压力诱导变形具有很高的灵敏度,并且对三维结构具有良好的顺应性。
<图片>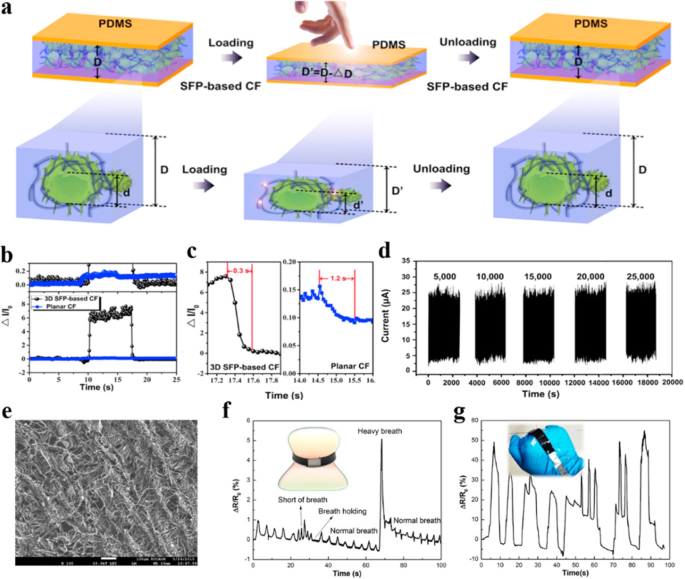
一 向日葵花粉微胶囊加压诱导的空心球结构传感器机理示意图。 b 基于 SFP 的复合膜 (CF) 和平面 CF 在 600Pa 压力下的瞬态响应。 c 两个相关传感器的松弛时间。 d 基于 SFP 的 CF 在 80 Pa 下的稳定性测试。经参考文献许可改编。 3. 版权所有 2017 爱思唯尔。 e 转化后的碳纸的 SEM 图像。 f , g 监测呼吸 (f ) 和手势 (g ) CP/PDMS 传感器的成人。经参考许可改编。 63. 版权所有 2017 美国化学会
图>电容力传感器
电容式传感器可以通过电容的变化来响应指示外力的变化。电容器通常由夹在两个导电板之间的介电层组成。用于计算电容的公式为\( C=\frac{\varepsilon_0{\varepsilon}_rA}{d} \),其中ε0是真空介电常数,εr是电介质的相对介电常数,A 是两块导电板的有效重叠面积,d 是两个导电板之间的间距。柔性电容式力传感器的电极通常使用碳纳米管 [25]、银纳米线 [26、3] 和导电离子材料 [27]。 PDMS、SEBS和Ecoflex等低模量弹性材料是很好的介电层候选材料。
微结构化电极或介电层可以显着增强电容式传感器的传感能力 [3, 2, 28]。如图 4a-d 所示,Quan 等人。 [3] 使用磨砂表面玻璃作为模板制备微结构 PDMS 薄膜作为柔性电容传感器的电极基板。他们将带有微结构电极的传感器与没有微结构电极的传感器进行了比较。图 4e-g 中的结果表明,具有微结构的传感器具有更高的灵敏度、更低的检测限和更快的响应时间。康等人。 [28] 开发了一种基于海绵状多孔介电层的高性能电容式压力传感器。海绵状多孔结构是通过将 PDMS 涂覆在堆叠有聚合物微珠的硅基板上,然后溶解聚合物微珠来实现的。然后将多孔 PDMS 薄膜转移到 ITO 薄膜电极上,从而产生具有超高灵敏度和高稳定性的电容式传感器。多孔 PDMS 压力传感器的灵敏度比基于裸 PDMS 薄膜的传感器高八倍以上。微结构电容传感器性能更好的原因可归结于以下两点。一方面,构造弹性体电极基底或介电层提高了器件的可压缩性。另一方面,微架构以有序的方式在电容器的导电板之间添加空气空隙,这使得介电常数在压力下发生变化。当外力作用在传感器上引起变形时,介质层中空隙的总体积减少,空气/弹性体混合介质层的介电常数增加,从而导致电容式传感器的电容值上升由两个因素:板间距的减小和介电常数的增加。此外,Pang 等人。 [2] 开发了一种具有金字塔形 PDMS 介电层和微毛结构界面的高灵敏度压力传感器,如图 5a、b 所示。图5c-f对比了四种不同界面几何形状传感器的桡动脉测试结果,表明微毛界面可以明显提高电容式压力传感器的信噪比。
<图片>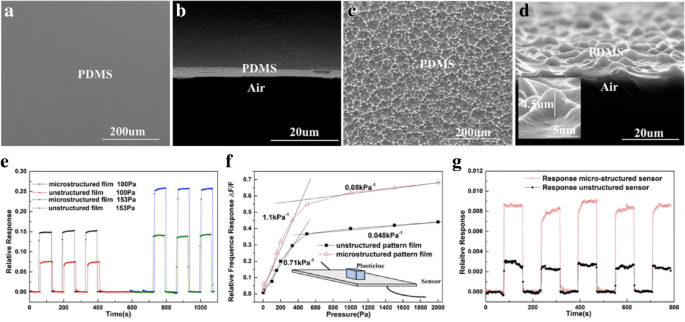
一 –d 俯视图的 SEM 图像 (a ) 和侧视图 (b ) 的非结构化 PDMS 薄膜,俯视图的 SEM 图像 (c ) 和侧视图 (d ) 的微结构 PDMS 薄膜。 e 不同结构传感器的相对响应比较。 f 两个结构化传感器的灵敏度测试。 g 两个结构化传感器在 1 Pa 压力下的响应。经参考许可改编。 18. 版权所有 2017 Elsevier
图> <图片>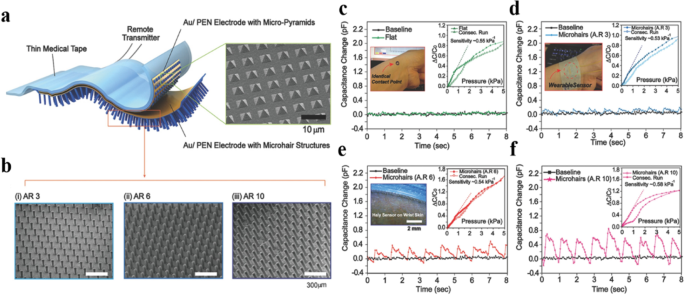
一 微毛结构传感器示意图。 b 具有不同纵横比的微毛结构的SEM图像。 c –f 使用具有不同界面几何形状的四种设备进行桡动脉测试:c 平坦的表面,纵横比为 d 的微毛结构 3、e 6、f 10
图>为了提高灵敏度,与有机场效应晶体管(OFET)集成也是电容式传感器广泛研究的项目。在 OFET 器件中,源漏电流直接取决于栅极介电电容。施瓦茨等人。 [29] 报道了使用微结构 PDMS 膜作为介电层和新型共轭聚合物聚异靛二噻吩-硅氧烷 (PiI2T-Si) [30] 作为半导体的高灵敏度 OFET E-skin 设备。集成微结构 PDMS 电介质的 OFET 器件实现了超高灵敏度 (8.4 kPa − 1) 在低压状态 <8 kPa 以及快速响应时间 (<10 ms)。这些优越的性能表明,这种装置在腕部脉搏波的高保真测量中很有前景。
与电阻式传感器相比,电容式传感器通常具有更高的灵敏度和更低的检测限。然而,它们的线性响应差以及对寄生电容和边缘电容的敏感性在实际应用中可能是一个挑战。
压电力传感器
压电效应是指机械刺激使某些各向异性晶体材料变形,引起内部偶极子极化,导致晶体两个相对表面之间存在电位差的现象。由于压电材料的独特特性,具有快速响应时间的压电传感器能够有效地测量高频动态信号,在自供电设备中很有前景。
柔性传感器中常用的压电材料包括 P(VDF-TrFE) [31, 32]、ZnO [33]、PbTiO3 [34] 和 PZT [35, 36] 等。 P(VDF-TrFE) 是其中一种柔性压电传感器最受青睐的材料,因为它具有柔韧性、制造工艺简单、稳定性好、压电系数大等优点。佩萨诺等人。 [31] 报道了一种基于通过静电纺丝制备的对齐 P(VDF-TrFE) 纤维阵列的柔性压电传感器。这种简单的压力传感器即使在极小的压力范围(约 0.1 Pa)下也表现出出色的传感性能。结果表明在人体运动检测和机器人电子产品方面具有非凡的应用潜力。尽管无机材料缺乏柔韧性,但许多纳米级无机材料和聚合物-陶瓷纳米复合材料(如 ZnO 纳米线 [33]、PZT 纳米带 [35] 和纳米片 [36],以及 P(VDF-TrFE)/BaTiO3 纳米复合材料 [4] ) 可以表现出一定程度的灵活性。申等人。 [33] 使用填充到 PDMS 中的锂 (Li) 掺杂的 ZnO NW 作为传感元件。 Li掺杂的ZnO NW-PDMS复合材料的压电输出电压是外加力和频率的函数。所制造的设备能够提供人体运动的瞬时信息,这对于电子皮肤设备在人体活动监测中的应用具有重要意义。压电传感器对于动态物理刺激的检测特别有用,但在静态信号的测量中表现不佳。这是因为压电材料产生的电压信号只会在施加或撤回压力的瞬间出现。为了解决这个问题,陈等人。 [34] 报道了一种基于 PbTiO3 纳米线 (PTNW)/石墨烯异质结构的用于静态测量的柔性压电压力传感器。在该器件中,由 PTNW 中的应变引起的极化电荷充当石墨烯中的带电杂质并影响其载流子迁移率。其工作机制是 PTNW 中的极化电荷增加了石墨烯中载流子的散射,从而导致载流子迁移率降低。基于上述机制,如图 6 所示,这种异质结构传感器比本征 CVD 生长的石墨烯压力传感器具有更高的灵敏度 [37, 38],并且能够测量静态机械信号。
<图片>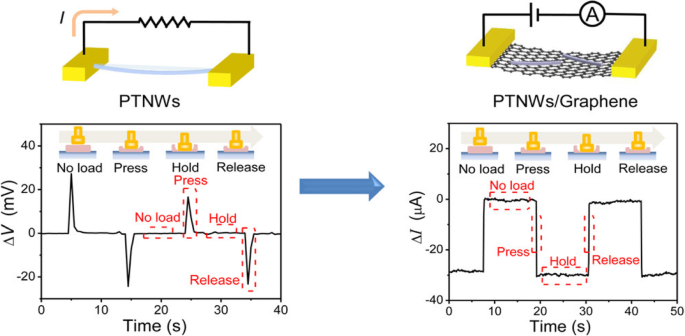
基于 PTNW 的压力传感器(左)和 PTNW/石墨烯晶体管在压力脉冲下的压力响应。经参考许可改编。 25. 版权所有 2017 美国化学会
图>灵活的温度传感器
温度检测是传感设备的重要组成部分。体温可以大量反映人的身体状况。健康人的体核温度相对恒定,一般在36.2~37.2℃之间。它与环境无关,而外壳温度会受到物理条件和环境温度的影响。体温的异常变化通常表明健康状况不佳。例如,体温升高是发烧或感染的症状,而体温下降可能表示贫血。为了实时检测电子皮肤设备的温度,人们开发了多种类型的柔性温度传感器。
电阻温度传感器
通过敏感材料的电阻变化来检测温度是类肤电子设备中最常用的温度测量方法。电阻温度系数(TCR)是电阻温度传感器灵敏度的重要指标。定义为温度每变化1℃时电阻的相对变化。已经报道了使用纯金属元素(Pt、Au、Cu)[39,40,41,42]、金属氧化物颗粒 [43]、碳纳米管 (CNT) 聚合物复合材料 [8, 9] 和石墨烯的各种电阻温度传感器[44, 45] 作为敏感材料。
金属由于其对温度的敏感性,长期以来一直用于温度检测。检测机制可以解释为,温度的升高增强了晶格的热振动,导致电子波的散射加剧,从而增加了电阻率。传统的基于金属的温度传感器提供有限的拉伸性或弯曲性。结构工程,如皱纹屈曲、直列马蹄形结构和刚性岛设计[39、41、46],已被证明是克服这些局限性的有效方法。如图 7a、b 所示,Yu 等人。 [39] 开发了一种基于弹性基板上的波纹薄膜传感元件的可拉伸温度传感器。该传感器是通过在预拉伸的 30% 柔性基板上溅射沉积 Cr/Au 薄膜 (5 nm/20 nm) 来制造的。如图 7c、d 所示,通过释放预应变形成的周期性波浪几何形状允许设备在性能不变的情况下拉伸高达 30% 的机械应变。韦伯等人。 [41] 报道了一种超薄、柔顺的皮肤状温度传感器阵列,使用薄(50 nm)、窄(20 μm)、蛇形金薄膜,通过微光刻技术制备。当采用先进的建模和分析技术实施时,可伸缩电子系统能够以毫开尔文精度对壳温度进行无创映射。
<图片>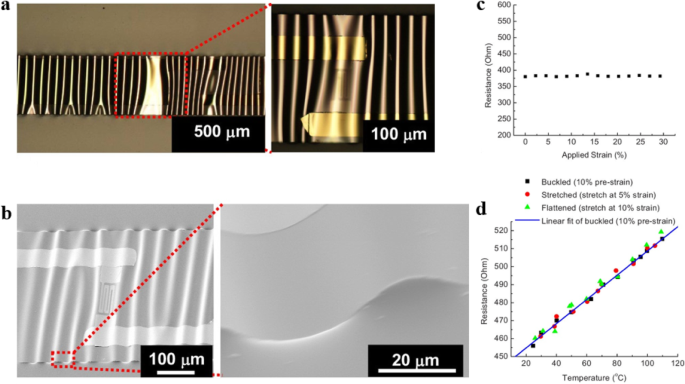
一 具有周期性波浪图案的可拉伸传感器的示意图。 b 可拉伸温度传感器的 SEM。 c 当传感器应变从 2.25% 持续拉伸到 30% 时,传感器电阻值的变化。 d 应变为 0%、5% 和 10% 的可拉伸传感器的电阻与温度之间的关系。经参考许可改编。 29. 版权所有 2009 AIP Publishing
图>上述工作有效地提高了金属基温度传感器的灵活性,但这些设备中使用的结构工程方法将可拉伸性限制在 25-30%。为了进一步打破柔性温度传感器的拉伸极限,需要使用固有的可拉伸材料。 Harada 及其同事 [8, 9] 推出了基于聚(3,4-乙撑二氧噻吩)-聚(苯乙烯磺酸盐)(PEDOT:PSS)-CNT 复合薄膜的柔性温度传感器,该薄膜通过印刷工艺制备。 PEDOT:PSS-CNT 混合温度传感器在 CNT 糊料和 PEDOT:PSS 溶液的不同复合比例下的灵敏度为 0.25 至 0.63%/°C,优于金属基温度传感器 [39,40,41, 42]。如图 8a、b 所示,Yan 等人。 [45]通过使用光刻过滤方法开发了一种可拉伸的基于石墨烯的热敏电阻,以制备具有微孔结构的石墨烯检测通道。该器件显示出高达 50% 的高固有拉伸性,并且其 TCR 可以通过机械应变进行有效调整,如图 8c、d 所示。然而,应变依赖性对于可穿戴传感并不理想,因为拉伸或扭曲传感器会改变热敏电阻的电阻。在传感器变形的情况下,不可能从单个数字信号中读取应变和温度值。在用固有可拉伸材料制备的热敏电阻中避免应变效应对温度传感的影响仍然是一个挑战。为了同时获得高拉伸性和应变适应性,朱等人。 [47]通过设计差分电路报道了一种基于具有应变抑制能力的CNT晶体管的温度传感器(电路图如图8e,f所示)。将具有超分子聚合物分选 SWCNT 图案化为半导体通道的单个可拉伸薄膜晶体管制造为温度检测装置。密集的未分类 SWCNT 网络和非极性 SEBS 薄膜分别用作源漏电极和栅电极以及栅电介质。主要机制可归因于半导体 SWCNT 网络中电荷传输的温度依赖性 [48]。通过采用静态差分电路配置,应变引起的阈值电压偏移被抵消,如图 8g、h 所示。只要在两个支路之间匹配,就可以抑制差分输出电压(VOD)。
<图片>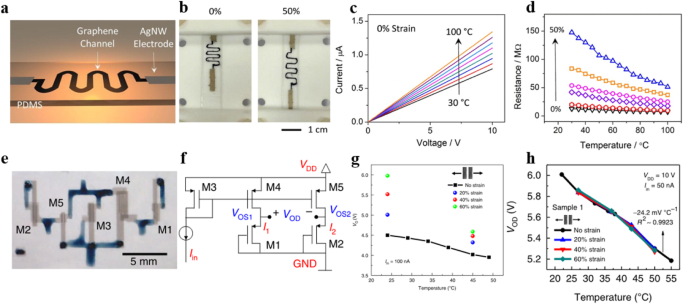
一 石墨烯热敏电阻的可拉伸示意图。 b 石墨烯热敏电阻在 0% 和 50% 应变下的图像。 c 电阻随温度变化。 d 电阻随温度在 0-50% 应变范围内的变化。经参考许可改编。 35. 版权所有 2015 美国化学学会。 e 由五个 TFT 组成的可拉伸温度传感电路的光学显微照片。 f 静态差分传感方法的电路原理图。 g 单个TFT的温度传感性能。 h 可拉伸静态差分电路传感装置的温度传感性能。经参考许可改编。 39. 版权所有 2018 Springer Nature
图>值得一提的是,这种TFT结构的器件已经被其他研究人员证明可以显着提高温度传感器的灵敏度。 Trung 等人。 [44] 为可穿戴电子设备制造了可拉伸电阻和门控温度传感器,并比较了两种传感器之间的性能差异。感温层是一种复合导电材料,通过将温度响应 R-GO 纳米片插入弹性体 PU 基质中形成。根据他们的测试结果,门控器件的温度灵敏度(每摄氏度 1.34%)高于电阻器件(每摄氏度 0.9%)。
Pyroelectric Temperature Sensors
A variation of temperature will change the remnant polarization of pyroelectric materials thus generating opposite bound charges on both surfaces of the crystal. Materials that have been found to exhibit pyroelectricity include different ceramics (PZT, LiTaO3, LiNbO3) and polymer (PVDF, P(VDF-TrFE)) [49,50,51,52,53]. A lot of pyroelectric devices have been fabricated on rigid substrate and widely used in missile detection, fire alarm, and other fields. Nevertheless, flexible pyroelectric devices still need to be explored. In particular, P(VDF-TrFE) is ideal for temperature sensing applications in flexible electronics. Tien et al. [51] directly used a highly crystalline β-phase P(VDF-TrFE) material with extremely large remnant polarization as gate insulator in an OTFT structure for temperature sensing. The remnant polarization inside the P(VDF-TrFE) can change with temperature, causing a change in the density the holes accumulated at the interface between the semiconductor channel and P(VDF-TrFE). Therefore, the source-drain current increases as the increase of temperature. The linear response of the device in a certain temperature range and its simple fabrication process suggest its potential application in flexible temperature sensors. However, for (P(VDF-TrFE)), the pyroelectric effect is indistinguishable from the piezoelectric effect, which means that mechanical deformation will interfere with temperature detection. To decouple strain-induced interference from temperature effect, Tien et al. [54] developed flexible pyroelectric OFET devices with piezo- and pyroelectric nanocomposite gate dielectrics formed by a mixture of (P(VDF-TrFE)) and BaTiO3 nanoparticles as well as piezo- and thermoresistive organic semiconductor channel(pentacene). The fabricated devices can extract effects from the target sensing signals successfully while the flexible sensor is under multiple stimuli because the two chosen materials were able to respond to strain and temperature in a disproportionate manner simultaneously. This approach is able to distinguish the temperature effects from strain for flexible pyroelectric sensors.
Flexible Physiological Biochemical Sensors
In order to understand all aspects of human health, various physiological biochemical sensors have been developed for analysis of vital biochemical signs, such as blood glucose [55, 56, 57, 58] and body fluids (sweat, interstitial fluids, saliva, and tears) [59, 60, 61]. Flexible biochemical sensors typically adopt chemical methods to detect the composition and amount of a biological substance. The chemical reaction between the sensing material and the target detection substance changes the electrical properties of the sensor, therefore the physiological health information can be obtained by analyzing the electrical parameters of the sensor.
Continuous measurement of glucose is vital to maintain the health and quality of life of diabetics. Commercially available products for glucose detection are performed by invasive lancet approaches that requires sampling the patient’s blood, leading to pain to the patient. New electronics fabrication techniques on flexible substrates have been developed to enable noninvasive wearable glucose monitoring.陈等人。 [55] developed a skin-like biosensor for noninvasive blood glucose monitoring via electrochemical channels. The detection mechanism and structure of this sensor are shown in Fig. 9a, b. A paper battery was attached to the skin to produce subcutaneous electrochemical twin channels (ETCs), through which more intravascular blood glucose was expelled from the blood vessel and transported to the skin surface. The outward-transported glucose thus can be measured easily by a glucose oxidase (GOx) immobilization layer. The experimental test results are shown in Fig. 9c, d. As can be seen from the figure, the monitoring results of the biosensor are in good agreement with the results of the commercial glucometer. Besides glucose monitoring, sweat analysis can be important in facilitating insight into an individual’s heath state. For example, sweat glucose is metabolically related to blood glucose and low electrolyte levels in sweat may be a sign of dehydration.高等人。 [61] presented a highly integrated wearable sensing system for multiplexed in situ sweat analysis. As shown in Fig. 9e, f, the sensing system composed of four different sensing elements for simultaneous and selective screening of a panel of biomarkers in sweat—sodium (Na+), potassium (K+), sweat glucose, and sweat lactate. They also exploited a flexible printed circuit board (FPCB) to realize the conditioning, processing, and wireless transmission of critical signals. According to the test results in Fig. 9, it can be seen that the wearable system can be used to measure the detailed sweat characteristics of a human subject and to evaluate the physiological state of the object in real time.
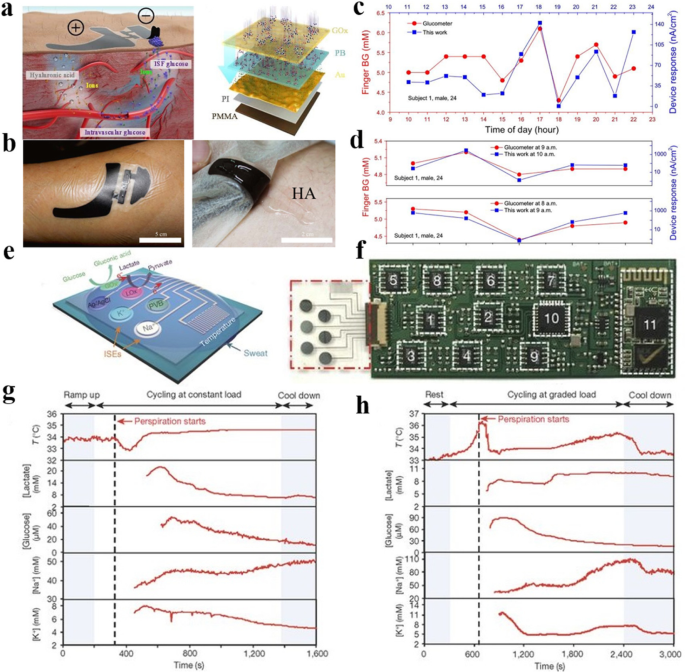
一 Schematic of the ETCs (left) and the biosensor multilayers (right). b A biosensor attached to skin surface for glucose monitoring. c Results of glucose monitoring in one day by a glucometer and a biosensor. d Results of glucose monitoring in 5 days by a glucometer and a biosensor.经参考许可改编。 48. Copyright 2017 American Association for the Advancement of Science. e Schematic of the sensor system for multiplexed sweat analysis. f Photograph of a flexible integrated sensing device. g The result of sweat analysis by wearing the sensor on the forehead of the subject. h The result of sweat analysis by wearing the sensor on the forehead of another subject.经参考许可改编。 54. Copyright 2016 Springer Nature
图>Multifunctional Sensors
Integrating multifunctional sensing components into one device is an important advance in wearable electronics. Future wearable electronics should enable to integrate the function of detecting multiple signals such as strain, pressure, temperature, humidity, gas [8, 9, 62, 63], and so on into a single device to provide more comprehensive human health and environmental information. Laminating multiple layers of thin film e-skin device with different sensing functions together is the major method to prepare multifunctional sensors. Harada et al. [8] fabricated a triaxial tactile sensor and temperature sensor array to simultaneously detect the tactile forces, slip forces, and temperature by using a printing manufacturing technique. Four strain sensors printed by a screen printer were designed with a PDMS fingerprint for a pixel, as shown in Fig. 10a, b. Three-axis force directions can be detected by characterizing the strain distribution at the four integrated force sensors with a finite element method (FEM). Figure 10c shows the measurement results of the multifunctional sensor when touching a fingerprint-like structure with a human finger. The integrated strain/temperature sensing array for e-skin application show good performance in imitating human skin.何等人。 [62] developed a multimodal all graphene e-skin sensor matrix. Three different sensors—humidity, thermal, and pressure sensors—were included in this matrix. Sprayed graphene oxide (GO) and reduced graphene oxide (rGO) were used as active sensing materials for the humidity and temperature sensors, respectively. Whereas the top PDMS substrate sandwiched between two CVD-graphene electrodes acted as the capacitive strain sensor, as displayed in Fig. 10d, e. The three sensors were integrated into a single unit through a simple lamination process. As can be seen from the test results in Fig. 10f–h, each sensor is sensitive to its associated external stimulus, but not affected by the other two stimuli. These results indicate that the E-skin device offers unique opportunities for healthcare applications in the future.
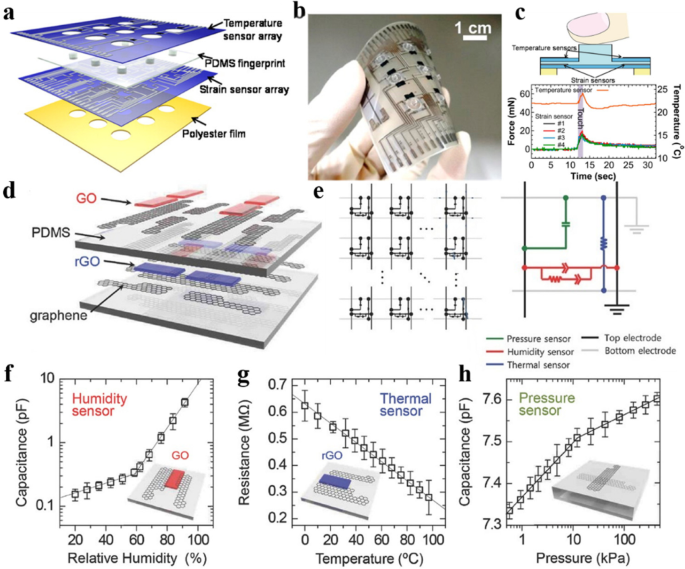
一 Schematic for the structure of multilayer sensor. b Picture of a 3 × 3 sensor array. c schematic and measurement results of the multifunctional sensor when touching a fingerprint-like structure with a human finger.经参考许可改编。 4. Copyright 2014 American Chemical Society. d Schematic diagram of the multimodal e-skin sensor. e Circuit diagram of the sensor matrix. f Performance of the humidity sensor based on GO. g Performance of the temperature sensor based on rGO. h Performance of the pressure sensor based on PDMS.经参考许可改编。 55. Copyright 2016 John Wiley and Sons
图>Functional Modules of Wearable Electronics
In order to develop highly integrated wearable system for applications in health monitoring, physical state assessment, and telemedicine, researchers have tried various manufacturing processes and device structures to combine different functions together. Self-powered modules working continuously without external power sources should be an integral part of future wearable electronics. In addition, for real-life application of wearable electronics in monitoring critical health information, a wireless digital system for processing and transmitting signals over long distances is necessary.
To realize independent operation of wearable sensors, nanogenerators based on piezoelectric, pyroelectric, and triboelectric effects have been developed to incorporate into wearable systems [64,65,66,67]. Nanogenerators are able to harvest mechanical energy or thermal energy from human activities to power wearable devices. Zi et al. [64] developed a tribo-, pyro-, and piezoelectric hybrid cell that is composed of a sliding mode triboelectric nanogenerator (TENG) and a pyroelectric-piezoelectric nanogenerator (PPENG) for self-powered sensing. The structure and working principle of the hybrid cell are shown in Fig. 11a–d. The TENG, fabricated with a piece of aluminum foil as the sliding layer and a piece of polytetrafluoroethylene (PTFE) film deposited on Cu electrode as the static layer, is able to harvest the sliding mechanical energy. The PPENG was fabricated by depositing a piece of PVDF with Cu electrodes on both sides to harvest the thermal energy generated by friction and the mechanical energy generated by the normal force. As can be seen from Fig. 11e–j, the hybrid cell is demonstrated as an efficient power source that can drive the LED with extended lighting time, and a versatile self-powered sensor for detecting both the subtle temperature alteration and strain on the surface of human skin. Nevertheless, the rapid development of flexible electronics places higher demands on corresponding power devices, which should be comparably flexible or stretchable.浦等人。 [65] reported a soft skin-like triboelectric nanogenerator that achieves ultrahigh stretchability (maximum stretch up to 12.6 or strain of 1160%) and high degree of transparency (96.2%) by using PDMS or LED as the elastomer electrification layer and PAAm-LiCl hydrogel as the electrode. This skin-like generator is capable of outputting an open circuit voltage of up to 145 V and an instantaneous power density of 35 mW m −2 through harvesting biomechanical energy. Meanwhile, the TENG-based electronic skin can serve as a tactile sensor to sense pressure and achieved a sensitivity of 0.013 kPa −1 . The development of self-powered, wearable platforms has opened up opportunities for many potential applications including soft robots, smart artificial e-skins, wearable electronics, etc. However, there are still limitations of flexible energy harvesting devices because the power generation of nanogenerators that have been reported so far cannot meet the needs of practical applications.
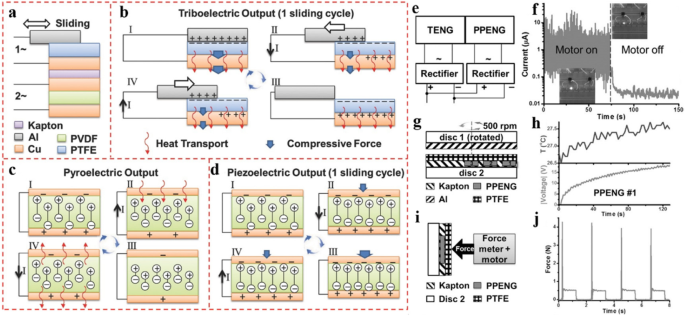
一 –d The structure and working principle of the tribo-, pyro-, and piezoelectric hybrid cell. e The circuit that hybridizes TENG and PPENG outputs. f The LED was lighted by the hybridized output current. g The schematic diagram of the structure used to demonstrate the temperature sensing. h The voltage and the temperature variation of the PPENG. 我 The schematic diagram of the measurement setup. j A force of approximately 0.5 N applied to the surface.经参考许可改编。 56. Copyright 2015 John Wiley and Sons
图>The integration of flexible sensors with information processing system is the next frontier for wearable electronics. Current research on flexible electronics mainly focused on the fabrication and optimization of sensing elements, while the research on flexible electronic circuits for information processing is relatively limited. The delivery and processing of human health information collected by the sensor still needs to be done by a computer. Wireless transmission of sensor data that has been reported so far is principally realized by combining a flexible sensor with a rigid silicon-based digital circuit technology. Pang et al. [2] built a custom wireless measurement system based on an XBee Series 2 radio module integrating to a programmed Arduino microcontroller. This system allows the sensor data to be wirelessly transmitted to a computer but is relatively bulky and not portable.高等人。 [61] devise a multiplexed sensing system that integrated the functions of signal conditioning, processing, and wireless transmission by merging commercially available technologies of consolidating integrated circuits on a flexible printed circuit board (FPCB), with flexible sensor technologies fabricated on elastic substrates. The introduction of FPCB technology bridges the technological gap between signal conditioning, processing, and wireless transmission in wearable sensors to some extent, but the flexibility and comfort of the system still do not meet the requirement of next-generation wearable electronics. Realizing skin electronics rely on the development of intrinsically stretchable circuits [68].
Conclusions and Outlook
In past several years, the rapid development of wearable electronics attracts extensive attention. Researchers have made many fruitful attempts and achieved good results in developing wearable electronics with high sensitivity, flexibility, and stability. This review analyzed recent research strategy and advancements in wearable electronics for human health detection from the aspects of force sensors, temperature sensors, physiological biochemical sensors, multi-functional sensor, and other functional modules applied in flexible electronics. The successful fabrication of flexible sensing devices with high sensitivity, low-cost, portability, and long-term stability indicates that flexible and wearable electronics will definitely become the mainstream in the field of medical care in the future. However, there are certain challenges remaining for practical applications of current wearable sensors in real life.
- 1.
Wearable electronic devices should be able to clearly identify the deformations caused by pulse, muscle movements, and external contact. While most of the flexible force sensors that have been reported so far cannot accurately identify the source and direction of external forces.
- 2.
In terms of temperature sensors, it is still difficult to achieve high stretchability, sensitivity, and strain adaptability simultaneously. Improving the sensing performance and eliminating the influence of the elastic deformation of the sensor on temperature detection remain important research topics.
- 3.
The detection accuracy of flexible physiological biochemical sensors is insufficient compared to traditional medical devices. Besides, most of the valuable physiological health information needs to be extracted from internal secretions. More biophilic implantable materials should be taken into consideration for the development of biochemical sensors to extract information from blood and muscles.
- 4.
Multifunctional sensors should be able to simultaneously detect pressure, stress, temperature, and other different signals such as humidity and gas atmosphere and avoid crosstalk between them. The realization of multifunctional sensors requires further development of new materials, nanotechnology, and device structure design.
- 5.
Processing the data in situ and transmitting them in real time are also essential parts of future wearable electronics. It is quite challenging to integrate multiple functional modules into a complete wearable system so that it can fully meet the requirements of practical applications.
数据和材料的可用性
不适用。
缩写
- Au:
-
Aurum
- 铜:
-
Cuprum
- CVD:
-
化学气相沉积
- LED:
-
发光二极管
- 西北:
-
纳米线
- OFET:
-
Organic field-effect transistor
- P(VDF-TrFE):
-
Poly(vinylidenefluoride-tirfluoroethylene)
- PAAm:
-
Polyacrylamide
- PbTiO3 :
-
Lead titanate
- PDMS:
-
Polydimethylsiloxane
- Pt:
-
Platinum
- PU:
-
聚氨酯
- PZT:
-
Lead zirconate titanate
- SEBS:
-
Styrene-ethylene-butylene-styrene block copolymer
- VHB:
-
Very high bond
- 氧化锌:
-
氧化锌
纳米材料