纳米技术在木制品行业的应用:综述
摘要
以木材为基础的工业是马来西亚经济增长的主要动力之一。森林是各种木质纤维素材料的来源,具有许多未开发的潜力,可用于生产可持续和可生物降解的纳米材料,这些材料具有非常有趣的特性,可用于木材工业本身或许多不同的应用领域。木质产品部门还可以利用各种现成的纳米材料来提高现有产品的性能或从森林中创造新的增值产品。本综述重点介绍了纳米技术在木制品行业应用的最新进展。
介绍
纳米技术被定义为对 1 到 100 纳米之间物质的操纵。纳米技术是一个多学科领域,它结合了科学和技术,旨在开发具有显着功能、物理和化学特性的新材料和改进材料 [1, 2]。与更高维度的相同物质相比,这种规模的材料具有独特的特性 [3]。因此,可以在许多学科探索通过纳米技术的各种创新应用。
纳米技术不仅作为技术推动者,还可以推动实现国家经济增长。马来西亚的林产品工业在 2019 年贡献了约 225 亿令吉的出口价值 [4]。从马来西亚出口的木制品包括锯材、单板、胶合板和线条。马来西亚的木材工业有机会充分发挥纳米技术的自身优势。必须支持和关注将纳米技术应用于木材工业,特别是在马来西亚的努力,以实现现有木材产品的多样化和增值,最终推动该领域的经济增长。
不能排除森林多年来对文明发挥着重要作用的事实,因为它已成为各种产品开发和开发的木质纤维素材料的来源。事实上,木制品在社会上早已以木材、家具、纸张和许多其他功能材料的形式存在[5, 6]。纳米技术在林业部门的应用,特别是在森林产品或木质产品中的应用值得所有相关参与者和利益相关者的关注。利用纳米技术可以生产出更坚固、多功能且重量更轻的木质产品 [3]。传统的林产品,如纸浆和纸张、木材复合材料、木材涂料和木材防腐剂,可以扩展或转化为新的或增值的产品,以找到更广泛和/或更高级的应用[5]。
在这篇综述中,纳米技术在木制品行业的应用可以分为两个[2]途径:
- 1
源自森林的纳米材料
对环境的日益关注引发了对引入可持续和可生物降解的纳米材料的巨大需求。这种称为纳米纤维素的新材料可以以安全和可持续的方式从森林资源中生产。来自森林的丰富木质纤维素材料引起了人们对将纤维素转化为纳米纤维素的兴趣。纳米纤维素主要是分子水平的纤维素,它具有几个关键特征,例如高强度和刚度、高强度重量比、电磁响应和大表面积 [5, 7,8,9]。这种材料的突出特性提供了超越林产品行业本身的巨大应用潜力。在木制品领域,可作为纸浆、纸张和木材复合材料中的增强剂或木器涂料中的涂料[10,11,12]。由于其多功能性,纳米纤维素可用作电子、传感器、电池、食品、制药和化妆品等非林产品部门的组件之一(作为基材、稳定剂、电极)[5, 13]。
- 2
纳米材料在木制品中的应用
纳米材料可用于增强现有木质产品的功能。例如,在木材涂料中使用纳米材料,如纳米氧化锌或纳米氧化钛,可以增强木材的耐用性、耐火性和紫外线吸收能力,并降低吸水率 [14,15,16]。另一方面,纳米胶囊在木材防腐剂中的应用 [17, 18] 可以通过确保化学物质可以更深入地渗透到木材中来改善木材对农药的浸渍,从而减少过度浸出的问题 [19]。这提高了处理过的木材对生物降解剂的耐久性。
已经发表了许多关于纳米技术在林业和林产品中应用的评论文章 [20, 21]。 McCrank [21] 的一篇文章对纳米技术在林业领域的应用进行了总体概述,而 Moon 等人。 [20] 讨论更多关于纳米压痕。据作者所知,关于该主题的出版物非常有限,特别是涵盖了选定的木质工业部门。这篇评论论文将重点介绍纳米技术在选定的木质部门的最新进展,即纸浆和纸张、木材复合材料、木材涂料和木材耐久性。此外,纳米纤维素作为新一代纤维素在木制品工业中的一些潜在应用在能源和传感器等领域也备受关注。
应用程序
纸浆和纸张
全世界纸和纸板的年产量超过 4 亿吨 [22]。说明当前的数字化时代并未停止社区对纸制品的持续使用。然而,不断增长的需求主要是针对各种包装产品 [23]。消费者对网上购物的偏好转变和电子商务的发展可能会刺激这种增长,电子商务的发展为人们在舒适的家中购物提供了如此便利,并将产品安全地交付给目标收件人。
纸是由木质纤维素材料经过一系列的初级加工、处理、造纸、干燥和涂层制成的。造纸阶段是添加和调整纸浆和添加剂的所有混合,以适应不同的最终产品。纳米技术以纳米材料或纳米添加剂的形式应用于纸浆和纸张。例如,可以利用森林资源或更确切地说木质纤维素材料来提取纳米尺寸的构建块或称为纳米纤维素,用作增强单元以提供独特的机械强度、功能和灵活性 [5]。这种新一代纤维素是通过细胞壁分层获得纳米原纤维或通过在纳米级提取结晶纤维素来生产的。造纸中加入纳米纤维素主要是为了增加纸的强度。造纸过程中使用的其他纳米材料包括纳米二氧化硅、纳米沸石等[22]。在造纸工业中加入纳米材料或纳米添加剂有助于提高纸制品的性能。
以下部分讨论了纸浆和造纸工业中使用或可能使用的主要添加剂。
纳米纤维素作为干湿强度剂
纳米纤维素作为纸浆和造纸领域的强度添加剂已被广泛研究 [24, 25]。纳米纤维素由于其丰富性、可用性以及其有趣的特性,如可生物降解、低毒性、优异的机械和光学性能、高表面积和可再生性而引起了极大的兴趣 [5, 7]。纳米纤维素实际上是纳米级尺寸的纤维素,可以从任何木质纤维素材料中分离和制备,包括木浆、非木材植物、木材和农业残留物、细菌纤维素和被囊类动物。纤维素是一种线性化合物,具有 β-1,4 连接的葡萄糖单元,包括结晶和无定形区域。使用无机酸从纤维素链中去除无定形区域导致纳米晶纤维素的分离,而通过高剪切机械作用导致的细胞壁分层导致纤维素宽度减少,从而形成纳米原纤化纤维素。这两类纳米纤维素是纸浆和造纸领域用于产品增强的研究最多的纳米材料。在过去的十年中,许多工作都集中在从各种植物资源制备纳米原纤化纤维素 (NFC) 和纳米晶纤维素 (NCC) 上。图 1 显示了使用透射电子显微镜 (TEM) 获得的纳米晶纤维素和纳米原纤化纤维素的图像。
<图片>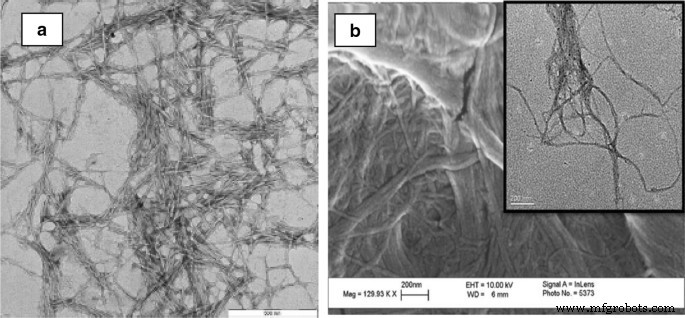
来自金合欢的NCC的TEM图像 一 来源 :Jasmani 和 Adnan [26]。经 Elsevier 和 NFC 许可从针叶浆中复制,b 来源 :赵等人。 [27]。经爱思唯尔许可转载
图>纳米纤维素的性质因制备方法和纤维素来源而异。表1显示了纳米原纤化纤维素和纳米晶纤维素的形貌和结晶度。
图>从纤维素到纳米纤维素
纳米原纤化纤维素是造纸中研究最多的纳米添加剂,因为已报道了许多工作 [3, 25]。纳米原纤化纤维素是通过对纤维进行选择性高剪切机械处理来制备的。有许多方法和方法组合来生产纳米原纤化纤维素。均质化和微流化是用于纳米原纤化纤维素制备的常用技术,包括将纤维置于高压下的小喷嘴中,重复通过,通常在 10 到 15 次之间 [32, 33]。这种类型的处理如果单独使用需要高能耗,因此使用化学物质进行预处理,例如 TEMPO(2,2,6,6-四甲基哌啶-1-氧基)介导的氧化 [34]、羧甲基化或酶 [35, 36 ] 通常需要节能。使用这种方法,由于流体流的高速和力引起的高剪切率,产生了纳米原纤化纤维素。另一种生产纳米原纤化纤维素的流行方法是通过研磨法。研磨机由静止和旋转的石盘组成。石头产生的剪切力将细胞壁纤维分解成个体化的纳米纤维。为了避免使用这种方法时由于聚集而导致宽度分布较宽 [37],作者建议在制浆和漂白过程后,原料需要保持在水中。这是为了在干燥状态下阻碍氢键网络的形成 [37, 38]。还研究了超声波处理方法来制备纳米纤维。超声处理涉及从超声波产生空化高剪切力,这可以分解纤维细胞结构 [39]。据说超声处理技术可生产分散良好且稳定的纳米纤维 [3]。除此之外,低温破碎也可用于将天然纤维转化为纳米纤维。纤维需要使用液氮冷冻,然后使用高剪切力。高剪切力对冰晶产生压力,迫使细胞壁破裂并释放微纤维[40,41,42,43]。
从天然纤维素中提取的纳米晶纤维素通常使用酸水解制备。其他涉及使用特定化学物质的方法,如 TEMPO 介导的氧化 [44] 和离子液体 [45],也已被研究用于制备纳米纤维素。纤维素酶的应用也有报道[46, 47]。
在酸水解过程中,纤维素的聚合度迅速下降,但它会稳定在某个点,称为聚合度平衡 [48]。这种行为的原因可能是由于无定形区域被酸迅速水解。当水解开始时,酸优先攻击无定形区域,因为它的体积大,并水解容易获得的糖苷键 [49]。一旦它水解了容易获得的键,在葡萄糖链的还原端和结晶区域表面以更慢的速度发生进一步的水解[50]。酸水解受酸类型、酸浓度、温度和时间等因素的影响 [49, 51, 52]。酸浓度、水解时间和温度条件的任何变化都会影响纳米晶纤维素的形态。例如,同时增加水解时间 [53] 和酸浓度 [29] 导致纳米晶纤维素缩短,而高温导致纤维素完全转化为葡萄糖 [50, 54, 55]。
在造纸中添加纳米纤维素可以使纸张具有更好的性能,因为它增加了纸张强度 [56, 57] 和密度 [58,59,60],还降低了孔隙率 [61]。添加纳米结构纤维素会产生与添加打浆纤维相似的纸张特性 [3, 25]。理论上,纸的强度可以通过加入湿强度剂和干强度剂 [24, 62]、添加功能化纤维 [63] 和打浆 [64] 来增加。增强导致纤维粘合能力[25]。布菲等人。 [25] 提出该机制可能是由于纳米纤维素充当纤维之间的连接器,导致纤维与纤维之间的粘合,从而增加了粘合面积。除此之外,这也可能是由于在光纤中创建的不同网络导致了粘合能力的增加。鉴于纳米纤维素的微米长度,它们可以作为连接相邻纤维的桥梁,从而形成更强的网络 [25]。由纤维和纳米纤维素构成的相互结合的网络增加了纸张的强度。
纳米纤维素作为纳米添加剂可改善内部粘合,从而增加干拉伸强度、降低透气性和不透明度,并提高密度 [3]。作为纳米级材料,纳米纤维素具有高表面积,因此可以更有效地形成氢键。它作为湿部添加剂添加,以提高干强度和提高留着率。常用于干强度添加剂的纳米纤维素为纳米原纤化纤维素。
将 NFC 添加到纸浆配料中有不同的策略,因为它可以与 [24, 62, 65] 直接添加或不添加助留剂 [58] 或与其他填料或长纤维混合 [66, 67] 然后添加助留剂.添加NFC后拉伸强度的增加与添加量有关。发现当将 3% NFC 添加到由打浆纸浆和阳离子淀粉组成的纸浆配料中时,观察到拉伸强度增加了 5% [24]。有趣的是,如果纸浆打得少,效果会更显着。例如,将 6% 的 NFC 添加到热机械纸浆中时,拉伸强度增加了 100% 以上。另一方面,将 NFC 添加到充分打浆的化学纸浆中时,其影响较小 [65]。因此,当将 NFC 添加到填充纸、机械纸浆和再生纸浆中时,预计会出现拉伸强度的提高。 Hii 等。 [65] 报道了这样的观察结果,即 NFC 被吸附到填料和纤维上,并将填料与纤维网络连接起来。在造纸中使用NFC的唯一主要缺点是它使排水变慢。排水在造纸中起着非常重要的作用,因为它直接关系到造纸的效率。排水时间越慢,出纸速度就越慢。因此,使用特定剂量的助留剂来提高纳米纤维在纤维表面的吸附能力非常重要,从而改善脱水[24, 68]。
NFC 具有介于干强度添加剂(如淀粉)和由打浆作用产生的细粉之间的特性,因为它们都会增加粘合面积 [3]。这是通过在纤维表面形成一层柔软而薄的层来实现的,这有助于在干燥过程中增加纤维粘合,同时填充纤维之间的空隙和孔隙,从而增加粘合面积 [3]。
纳米纤维素作为阻隔性涂层材料
纳米纤维素还可以用作包装纸的涂层材料,因为它具有良好的阻隔性能。使用纳米纤维素作为涂层元素的好处是脱水问题不再是问题,因为它是在纸张制成后添加的。可以采用不同的方法来应用纳米纤维素,包括喷涂、棒涂、施胶和辊涂。据报道,纳米纤维素特别是纳米原纤化纤维素的应用可提高氧气阻隔性和耐油性 [69]。在一个示例中,观察到透气性从 69,000 减少到 4.8,从 660 减少到 0.2 纳米 Pa -1 分别用于未漂白纸和防油纸 [70],使用棒式涂布机。 Syverud 和 Stenius [71] 在针叶木浆上应用 0% 到 8% 不等的 NFC,发现阻隔性能从 6.5 纳米显着增加到 360 纳米 Pa -1 s −1 .这是由于纳米原纤维的增加导致孔隙率降低。 Hult 等人也尝试了 NFC 和虫胶的应用。 [72] 在纸和纸板上导致透气性、氧气透过率和水蒸气透过率降低,使其成为阻隔包装的潜力。
不仅如此,纳米纤维素特别是纳米原纤化纤维素可以转化为独立的薄膜或纳米纸。该纳米纸透明且柔韧,可用作电子应用的基材[73]。
纳米材料作为性能改进的保留剂
造纸中加入助留剂,以提高功能性化学品在纸上的留着率。一些纳米材料已经在纸制品中进行了测试,例如使用纳米沸石 [74] 和纳米二氧化钛。纳米沸石在造纸工业中用作干燥剂以吸收水分,如果用于特种纸还具有去除气体排放的作用。由空隙和孔隙组成的纳米沸石的高表面积有助于该过程。与对照样品相比,在纸中添加纳米氧化钛可以形成具有更好动态弹性模量的纸[75]。
用于属性增强的纳米填料效应
在造纸工业中使用填料主要是为了降低成本,因为填料通常比纸浆本身便宜。除了作为强度添加剂进行研究外,纳米纤维素还可以用作填料。添加纳米原纤化纤维素可以减少木浆用量,增加填料用量,从而降低生产成本[76]。此外,所生产的纸张具有增强的特性,例如低孔隙率和高不透明度。据报道,添加 2-10% 的纳米原纤化纤维素作为填料会导致 50-90% 的强度增加(Future Markets Inc. 2012)。纳米粘土可用作造纸中的添加剂,以降低透气性,从而延长纸张的保质期。这对于气体和水屏障在防止食品和饮料变质方面起着重要作用的包装行业至关重要。
纳米碳酸钙用作填料以改善光散射。使用纳米结构颗粒改性沉淀碳酸钙对光散射有积极影响 [77]。沉淀的碳酸钙涂有硅酸盐和硫化锌纳米颗粒。野生等。 [78] 报道了一项类似的研究,其中纳米涂层用于实验室、中试和工厂试验。研究发现,纳米粒子涂层具有良好的印刷质量、防水性和尺寸稳定性。添加到纸张配料中的纳米氧化锌赋予纸张抗菌性能。同时,添加纳米氧化锌后,纸张的亮度和白度等光学性能以及可印刷性也得到了改善。纳米氧化钛与 β-环糊精在涂布纸中的结合也得到了研究 [79]。发现纳米材料混合物对二甲苯的降解效果优于仅涂有纳米氧化钛的纸张。
纳米材料作为性能改进的施胶剂
造纸时添加施胶剂,以提高抗水/液体渗透性,使纸张适用于印刷和书写用途。纳米二氧化硅的使用可以提高光学性能并将透印率降低 30%。发现涂有纳米二氧化硅的纸比未涂布的纸具有更好的光学密度、尺寸稳定性和印刷质量[80,81,82]。
木质复合材料
木材是大自然送给人类的礼物,因为它是一种可生物降解和可再生的材料,可用于许多应用。然而,由于白蚁攻击和其他原因,木材本身具有几个弱点,例如易变质、不灵活和不耐用。利用木纤维生产木复合材料有其自身的缺点,因为它们具有低堆积密度、低热稳定性、高吸湿性和生物降解敏感性。纳米技术已应用于许多科学领域,可用于提高许多材料的质量,包括木材和木材复合材料。
纳米纤维素作为增强材料
在基体材料中使用纳米纤维素作为增强材料的原理导致了许多研究工作正在进行中。通过添加纳米级纤维素,纳米复合材料在许多方面都具有出色的性能,这是微复合材料无法实现的[83]。这些纳米纤维素增强复合材料能够替代传统的复合材料。通过对NCC进行适当的修饰,可以开发出各种性能优异或显着改善物理、化学、生物和电子性能的功能纳米材料。纳米复合材料的性能取决于基质材料的性质、纳米纤维素的特性、纳米纤维素在基质材料中的分散性以及填料与基质材料的界面相互作用等几个因素[84]。
纳米纤维素作为各种基质材料的增强剂已被广泛研究。由用纳米纤维素增强的天然或合成聚合物组成的聚合物纳米复合材料已成为一类有前途的材料。制备这些纳米纤维素增强聚合物时,最常见的目标是提高机械性能 [85,86,87]。石油基聚合物通常分为热塑性和热固性类型。热塑性和热固性聚合物之间的区别在于保持其长链分子的键合,前者由弱范德华键保持,而后者由强共价键保持[88]。已经研究了多种热固性材料用于纳米纤维素复合材料。例如,环氧树脂由于其优异的粘合性能和固化后良好的机械性能(高模量、低蠕变和合理的高温性能)已被用于先进材料产品。然而,由于高度交联的结构,它很容易在冲击下失效[89]。通过添加功能化纳米纤维素作为增强材料,环氧基纤维素纳米复合材料的机械性能得到显着提高 [90, 91]。已经进行了一种制备具有定向结构的环氧-纤维素纳米纤维复合材料的方法[92]。该工艺结合了冰模板(或冷冻浇铸)方法,以制备高度多孔的纳米纤维素网络,然后用作浸渍生物环氧树脂的预制件。结果表明,纳米复合材料的弹性和储能模量在两个测试方向均优于纯环氧树脂,纵向强度有所提高。另一种最常用的热固性树脂是不饱和聚酯 (UP)。纳米纤维素含量高达 45 vol% 的纳米结构 UP 生物复合材料,远高于之前的任何研究,已成功加工和表征 [93]。纳米结构的纳米纤维素网络增强剂不仅大大提高了 UP 的模量和强度,而且还大大提高了延展性和韧性。图 2 显示了使用场发射扫描电子显微镜 (FE-扫描电镜)。 FE-SEM 图像清楚地表明,与 NCC 相比,乙酰化 NCC 均匀分散到 PHB 中。均匀性有助于增强材料与聚合物基体之间的强界面相互作用[94]。
<图片>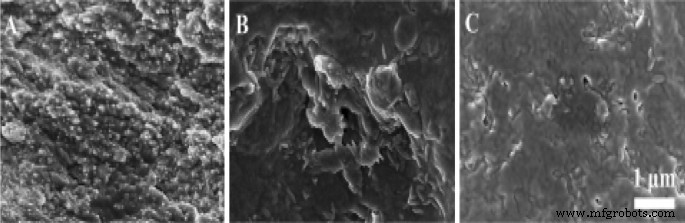
a断口形貌的FE-SEM图像 PHB/NCC-15,b PHB/乙酰化 NCC(II)-15, c PHB/乙酰化 NCC(IV)-15 纳米复合材料。 来源 :甘等人。 [94]。经ACS许可转载
图>表 2 说明了将纳米纤维素掺入热塑性聚合物中的情况,例如 PLA、PVA、淀粉、PU、PP 等。用于开发这些纳米复合材料的工艺包括溶剂交换、水分散、溶液浇铸、接枝、芯背泡沫注塑、电纺、凝固和热压,以及原位阴离子开环聚合反应等。大多数研究报告称,在纳米复合材料中添加纳米纤维素可提高纳米复合材料的机械性能(强度、刚度、抗蠕变性、弹性)、热稳定性、阻隔性能,甚至某些纳米复合材料的抗菌和抗氧化功能,具体取决于成分使用的纳米复合材料。总体研究结果表明,纳米纤维素在热塑性基质中的增强取得了积极成果,这促使并鼓励更多研究生产更绿色、更可持续的复合材料。
图>天然聚合物通常存在于自然界中并且可以被提取。天然聚合物不是热塑性塑料,只有少数例外。然而,化学和物理改性技术能够引发来自生物质资源(如纤维素、木质素和几丁质)的天然聚合物的热塑性。由于环保意识的增强和对绿色产品的需求,各种用纳米纤维素增强的天然聚合物已被用于生产生物纳米复合材料。报告在骨组织再生中使用植物来源的纳米纤维素的研究数量非常有限。其中一项研究 [113] 报道了通过合成 (TEMPO) 氧化纳米原纤维化纤维素 (TNFC) 或纳米晶纤维素 (NCC) 与羟基磷灰石 (HA) 来制备纳米复合材料。发现复合材料在外部和致密皮质骨范围内比基于 NCC 的复合材料表现出更好的压缩强度、弹性模量和断裂韧性。此外,该复合材料不会对人骨源性成骨细胞产生细胞毒性,而是提高了它们的活力,使其有望用于承重应用中的骨组织再生。
使用的其他天然生物聚合物包括海藻酸钠、纤维素和蛋白质,它们具有可生物降解、生物相容性和低毒性等特性。例如,海藻酸钠作为一种优良的生物材料已被广泛应用于组织工程、药物输送、食品包装和生物医学应用等许多领域。然而,较差的机械强度和不受控制的降解性能限制了其应用。通过开发将纳米纤维素掺入海藻酸盐基质中的纳米复合薄膜,已经进行了多次尝试来克服这些问题。发现将NFC并入藻酸盐基质中改善了其耐水性和机械性能。进一步的研究表明,通过超声波处理促进分散,与 NFC 相比,TEMPO 介导的氧化 NCC 在增强藻酸盐生物聚合物方面具有更好的效率。将纳米纤维素整合到几丁质、壳聚糖、大豆分离蛋白(SPI)和亚麻籽胶基质中的仿生协同增强策略的相似概念为构建高性能纳米复合材料开辟了新途径。
用于增强人造板性能的纳米颗粒
木质复合材料通常被描述为多种产品,其具有由粘合剂结合在一起的木质元素的组合。木质复合材料的优势之一是它们可以针对不同厚度、等级和尺寸的特定质量或性能要求进行设计。木质复合材料的制造利用了木材的天然强度特性(有时比普通木材具有更高的结构强度和稳定性)。 On the other hand, wood composites also have disadvantages such that they require more primary energy to manufacture when compared to solid lumber. Hence, wood composites are not suitable for outdoor use as they can absorb water and are more prone to humidity-induced warping than solid woods. The adhesives used release toxic formaldehyde in the finished product. Nanotechnology can be utilised to improve the quality of wood-based composites to fulfil the increasing demand for existing products and for new products to be used in new applications.
The main drawbacks of wood are its susceptibility and biodegradability by microorganisms and also dimensional instability when subjected to varied moisture content. These are mostly due to the cell wall main polymers and their high abundance of hydroxyl groups (OH) [114]. Wood is naturally hygroscopic, and moisture absorption by wood materials is directly related to the exposed surface area. The addition of inorganic nanoparticles to wood composites has been reported to enhance the composites’ anti-microbial properties. Nanoparticles of zinc oxide (ZnO) exhibit good antimicrobial activity. These nanoparticles are added into melamine-urea formaldehyde (MUF) glue before being used for particleboard production [115]. The findings show that there were increments in bioresistance of the particleboards against the Gram-positive bacterium Staphylococcus aureus , the Gram-negative bacterium Escherichia coli , the molds Aspergillus niger and Penicillium brevicompactum as well as the brown-rot fungus Coniophora puteana . Silver nanoparticles which are well-known biocide additives also exhibited similar antibacterial and anti-mold efficiency effects when applied onto the melamine-laminated surfaces of particleboards [116]. The combination of nanocopper oxide and alkane surfactant was also confirmed to improve water and termite resistances of treated plywood specimens [117]. Modified starch-based adhesive was explored as another option to increase the decay resistance of particleboard. Particleboard bonded with modified PVA/oil palm starch added with nanosilicon oxide (SiO2) and boric acid was found to be more decay resistant than particleboard bonded with their native starch [118]. The addition of nano-SiO2 and boric acid as the water repellent and antifungal agents, respectively, have prevented the microorganism's activity in the final particleboard.
The manufacture of wood composite panels can be improvised by developing methods to shorten the cure time of the resin during hot-pressing, which could speed up production or improve overall quality of the board. Heat transfer which effects the pressing time of a wood-composite panel varies with thickness, press temperature, closing rate, and mat moisture distribution. The addition of ZnO nanoparticles increased the heat transfer at the centre of the particleboard during hot-pressing causing a greater degree of resin cure and improved the physico-mechanical properties [119]. High conductive nanoparticles such as multiwalled carbon nanotubes (CNTS) and aluminium oxide (Al2O3) were also proven to enhance thermal and mechanical properties of medium density fibreboard [120]. The study also reported that although activated carbon nanoparticles did not give any significant effect to physical and mechanical properties of the board, they have more accelerated effect on the curing of urea formaldehyde (UF) and reduction in the formaldehyde emission compared to the other two nanofillers.
In fabricating the wood composites, the adhesives play exceptionally significant role which affect the composites properties which include the mechanical properties, their ability to perform in wet conditions and their effects on the environment. Urea formaldehyde, melamine urea formaldehyde and phenol formaldehyde are commonly used in the wood composites industry. The utilisation of nanoparticles has led to improvements of the properties of adhesives. Many studies have been conducted to produce nanomaterial-reinforced wood composites with enhanced physical and mechanical performance and reduced formaldehyde emission. Nanoclays have been shown to be excellent fillers and reinforcement for the resin matrix, and significantly enhancing strength, toughness and other properties. The modification of UF adhesive using nanoclay particles for plywood fabricated with three forest species from fast-growth plantations:Cordia alliodora , Gmelina arborea and Vochysia ferruginea were reported by Muñoz and Moya [121]. It was determined that nanomodification of the resin with nanoclay at 0.75% improved the moduli of rupture and elasticity of the board. The effect of using nanoclay particles in PF adhesives significantly elevated mechanical properties of the adhesive in the bondline and contributed an increase in the macro-bonding strength of plywood [122]. Interestingly, transition metal ion-modified bentonite (TMI-BNT) nanoclay was used to covert crystalline UF resins to amorphous polymers by blocking the hydrogen bonds via in situ intercalation method [123]. This resulted in 56.4% increase in the adhesion strength and 48.3% reduction in the formaldehyde emission.
Other nanoparticles used to improvise the physical and mechanical properties of wood composites include nanowollastonite (NW) [124, 125] nano-ZnO [126], nano-SiO2, nano-Al2O3 [127], nanosilver [128] and nanocellulose (NCC and NFC). The NCC was utilised as filler for the adhesive, whereas NFC was applied as a binder to the formulation of the composite boards. Recently, it has been revealed that the addition of micro- and nanofibres of cellulose have advantageous effects on the properties of resin. Based on the investigations, it was found that the addition NCC significantly improved the mechanical properties of plywood in the amount of 10%/100 g of solid resin [129]. On the other hand, the physical properties of particleboard after adding the NCC to UF adhesives showed smooth surface, insignificant difference in the density and moisture content of the panels and only high value of nanocellulose content exhibited significantly higher in thickness swelling [130]. Meanwhile, particle boards panels manufactured using NFC as the bonding materials were shown to meet the industry requirements in terms of mechanical properties for low density grades [131]. For high density particleboard, it was estimated that the increased NFC ratio and higher pressure could improve internal bond properties. Even the nail and face screw withdrawal strength was found to be increased with the increase in NFC addition ratio and panel density [132, 133].
Wood Coating
Forests are primarily or partially used for the production of wood and non-wood forest products. Non-wood forest products include bamboo, rattan, firewood, charcoal, damar, palm, etc. There is a huge demand for high quality wood, but the availability of wood from natural forest has been declining. Consequently, the search for non-wood resources as an alternative to wood has been accelerated. Due to its rapid growth property, bamboo has been developed into one of the most important non-wood forest products. Wood is a natural biologically self-assembled polymeric structure (cellulose, lignin, hemicellulose). It is one of the most versatile materials and has been used for centuries in the form of building and structures. However, wood is subjected to intense oxidative degradation processes such as photo-oxidation, chemical oxidation, thermal decomposition and photolysis reactions from the environment, including ultraviolet (UV) light, moisture, chemical pollutant and heat/cold variations [134]. Even non-wood materials like bamboo itself is a natural organic material which is rich in protein, carbohydrate and other nutrients and is prone to mildew, being eaten by moths and rotting. Hence, the final products of wood and non-wood products conventionally comprise additives which can be used as coatings for protection and aesthetic appearance improvement, preservatives for protection against fire and biological factors (fungi and insects).
Coating is a process of applying a layer to the substrate surface. Examples of common coatings applied to wood surfaces are varnishes, lacquers, and paints whose purpose can be both protective and decorative. The main components of the coatings determine their fundamental properties such as binders, pigments, solvents, fillers, and additives [135]. Each element contributes specific properties to the wood surface. The binder contributes to the adhesion of the pigment to the wood and creates a protective layer, while the pigment provides colour and form non-translucent surface layer. The solvents give necessary viscosity for coating application, and the addition of the fillers alters the colour strength and the gloss of the coating. As for the additives, they inhibit mould and decay, assist the drying process, improve the adhesion properties and control the finishing. However, there are weaknesses of coatings such as limited flexibility, strength loss, disproportionate adhesion between coating layer and substrate, inferior abrasion resistance and less durability.
Nanocoating has the capability to resolve these issues. Nanocoating is a process by which a thin layer of thickness about < 100 nm is deposited on the substrate for improving some properties or for imparting new functionality. The nanocoating can be used not only on nanomaterials but also on a bulkier material with an extremely thin layer coating without affecting the topography of the substrate surface. The application of nanocoating in wood and wood products is mainly focused on the improvement of durability, mechanical properties, fire resistance and UV absorption as well as decrease in water absorption. One approach to enhance the functionality and the end user value of nanocoating is the addition of nanoparticles [136]. These nanoparticles have very large surface-to-volume ratios due to their morphology, which allows them to interact intensively with their surroundings, and their nanosize ensures transparency is still sustained.
Nanoadditive for Durability Improvement
Nanocoatings are able to improve the durability of wood and non-wood products by utilising nanoparticles and nanodelivery systems that make the changes at the molecular level of the products. One of the aims of coatings is to prevent the growth of various microorganisms like fungi and bacteria. Nanosized particles of metal oxides, such as zinc oxide (ZnO) [137, 138], titanium oxide [138, 139] and cerium oxide (CeO2) [140] were reported to demonstrate strong antimicrobial properties. Studies have been conducted in the direct deposition of nanoparticles onto wood surfaces or direct functionalisation of wood surfaces with nanoparticles. ZnO nanoparticles were successfully fabricated on the surface of bamboo timber by a simple low-temperature wet chemical method based on sol–gel-prepared ZnO seed layers. The findings indicated that the treated bamboo timber had better resistance against Aspergillus niger V. Tiegh (A. niger) and Penicillium citrinum Thom (P. citrinum), but poor resistance against Trichoderma viride Pers. ex Fr (T. viride) [141]. Graphene also demonstrates superior ability to inhibit bacterial growth. Hence, the combination of utilising reduced graphene oxide and nano-ZnO to coat bamboo-based outdoor materials via a two-step dip-dry and hydrothermal process, resulting in the improvement of the mould resistance and antibacterial activity properties [142]. Similarly, the nanostructured ZnO using a hydrothermal process has also provided an effective protection of wood surfaces from biodeterioration [143].
Waterborne polyurethane [144] coatings (WPU) incorporated with nanocrystalline cellulose (NCC) and silver nanoparticles (AgNPs) were used to improve antibacterial property of wood board [145]. The AgNPs were known for their antimicrobial material but aggregated easily during the preparation process. Therefore, NCC was introduced to assist with the blending and dispersibility of AgNPs with WPU or other coatings. In addition, NCC was also a good reinforcing agent to improve the mechanical properties of nanocomposites. Mini emulsion polymerisation was also used to synthesise an acrylic latex coating containing AgNPs, which will limit the growth of black-stain fungi on the wood surfaces [146]. The study of the antibacterial effect of silver and zinc oxide nanoparticles in acrylic coatings applied during the treatment of commercial wooden composites such as particleboard and medium density fibreboard was conducted [147]. Ag and ZnO nanoparticles were partly more effective against the Gram-negative bacterium Escherichia coli compared to the Gram-positive bacterium Staphylococcus aureus .
Nanoadditive for Water Absorption Improvement
It is well known that wood is susceptible to water or moisture. This is due to the hydrophilic nature of the cell wall constituent polymer and its capillary-porous structure. The interaction between wood and water leads to biodegradation of wood, dimensional instability and accelerated weathering. Although there are conventional chemical modifications being used to improve the hydrophobicity of wood, the accessibility of water into wood is still not completely retarded [148]. Furthermore, the chemicals used in the treatment process are possibly hazardous. Hence, nanotechnology is used as an alternative approach for wood modification and functionalisation. The incorporation of nanoparticles into polymeric coatings is used to improve water absorption of wood surfaces. Two approaches are utilised to integrate the nanoparticles into the coatings, namely solution blending and in situ addition [149]. The first approach (solution blending) is when a solvent combines with the polymer before being dispersed onto the wood surfaces. This physical method can be applied through dipping, brushing and spraying [150].吴等人。 [151] reported that a superhydrophobic coating was constructed on the surface of poplar wood with a contact angle of up to 158.4° through the waterborne UV lacquer product (WUV) which was modified by ZnO nanoparticles and stearic acid. Compared with WUV, the water resistance of zinc stearate/waterborne UV lacquer super-hydrophobic coating (ZnSt2/WUV) was stronger, which was conducive to prepare superhydrophobic coatings in an easy and environmentally friendly. Interestingly, a water-based varnish added with TiO2 nanoparticles was used to evaluate the finishing of nine tropical wood species [152]. It was found that the incorporation of TiO2 nanoparticles decreased the values of water absorption and after a year of weathering exposure, the varnish with no added TiO2 nanoparticles degraded completely, while the modified varnish film endured. Other examples of superhydrophobic wood coatings which were successfully prepared are lignin-coated nanocrystalline cellulose (L-NCC) particles/polyvinyl alcohol (PVA) composite paint system [153], UV-light curable methacrylic-siloxane-cellulose composite coatings [154], Fe 3+ -doped SiO2/TiO2 composite film [155] and polydimethylsiloxane (PDMS)/silica hybrid coating system [156].
The second approach is the in situ addition or a chemical process which involves compound addition directly to monomers and subsequent polymerisation. The nanoparticles are synthesised in situ by chemical reactions on the wood surface such as hydrothermal methods or solgel deposition.高等人。 [157] applied a simple and effective method in preparing superhydrophobic conductive wood surface with super oil repellency using AgNPs modified by fluoroalkyl silane. The multifunctional coating could be commercialised for various applications, especially for self-cleaning and biomedical electronic devices. In another study, bamboo was treated using ZnO sol, and the ZnO nanosheet networks were grown hydrothermally onto the bamboo surface and subsequently modified with fluoroalkyl silane [158]. The successfully treated bamboo exhibited superior properties such as robust superhydrophobicity, stable repellency towards simulated acid rain, UV-resistant and fire-resistant. Similar superior properties were obtained when bamboo timber prepared by the hydrothermal deposition of anatase TiO2 nanoparticles and further modified with octadecyltrichlorosilane [159]. Superhydrophobic wood surfaces can also be prepared using approaches such as layer-by-layer [160] assembly of polyelectrolyte/TiO2 nanoparticles multi-layers and hydrophobic modified with perfluoroalkyltriethoxysilane (POTS) [161], spray coating of a waterborne perfluoroalkyl methacrylic copolymer (PMC)/TiO2 nanocomposites onto the PDMS pre-coated substrate [162] and a one-step hydrothermal process using tetrabutyltitanate (Ti(OC4H9)4, TBOT) and vinyltriethoxysilane (CH2CHSi(OC2H5)3, VTES) as a co-precursor [163]. Even a biomimetic approach as to produce a lotus-leaf-like SiO2 superhydrophobic bamboo surface based on soft lithography was successfully carried out [122].
Nanoadditive for Mechanical Properties Improvement
The inorganic particles integrated into organic polymers are commonly used in wood coatings to increase the mechanical properties. As fillers, the rigidity and hardness of the inorganic materials are combined effectively with the polymer’s processability. The inorganic particles when apply in micron size have disadvantages such as they reduce the flexibility of the material and decrease the transparency of the coating system [164]. The utilisation of the inorganic particles in nanosize increases the surface area and the ratio of the interfacial area, which subsequently influences the properties of the raw material [165]. Recent studies have investigated on using nanocellulose as a renewable reinforcement to develop a bio-based nanocomposite coating system with improved performance. Nanocellulose was surface modified due to the issue of incompatibility with the polymer matrix. The addition of TEMPO-oxidised cellulose nanofibres improved the mechanical properties of the WPU coating [144, 166]. In the case of the non-polar polymer matrix [167], nanocrystalline cellulose was modified by two methods, with acryloyl chloride or a cationic surfactant [167]. An increase in NCC loading level up to 2% increased hardness, elastic modulus, and tensile strength.
Nanosilica is another common nanoparticle that is applied for the improvement of mechanical properties. Among the advantages of using nanosilica are its high hardness and can easily be chemically modified to improve its compatibility with the polymer matrix. A recent study by Meng et al. [168] reported castor-oil-based waterborne acrylate (CWA)/SiO2 hybrid coatings with organic–inorganic covalent cross-linked network structures were prepared via solgel and thiol-ene reactions. The finding showed that beside the emulsions had good stability, the thermal and mechanical properties of the coating improved significantly at 10 wt% of SiO2. The improvement of mechanical properties with nanosilica addition was also described in other coating system such as waterborne nitrocellulose [169] and acrylate [170].
Nanoadditive for UV Absorption Improvement
The process of wood photodegradation begins directly after being exposed to solar light, and then the colour changes and progressive erosion of the wood surface occur. The UV radiation is capable of photochemically degrading the polymer structure components of wood (lignin, cellulose and hemicellulose) [171]. The photodegradation process usually results in reduced water resistance of wood and wood-based materials which lead to further biodegradation under outdoor exposure conditions. The intense damage to materials due to the UV component in solar radiation can be prevented by using light-stabilisation technologies, surface coatings or by replacing these materials with materials that are more resistant against UV radiation [172]. Nanoparticles can be utilised to improve the UV resistance for solvent, waterborne and UV coatings in order to protect the wood surfaces. Nanoparticles that contain functional coatings to achieve UV-blocking properties offer a high level of protection against UV without affecting the transparency of the surface. The small size of the nanoparticles gives a significant increase in effectiveness of blocking UV light compared to natural material due to their large surface area-to-volume ratio.
The use of UV radiation absorptive coatings serves to prevent lignin degradation from UV light. Among the nanoparticles used as UV absorbers are mainly TiO2 and ZnO. Wallenhorst et al. [173] reported a system composed of a Zn/ZnO coating and additional polyurethane sealing strongly reduced photodiscolouration of the wood surface and proved to be chemically stable. The combination of benzotriazole (BTZ) and ZnO nanoparticles was applied as the UV absorbers in acrylic-based bamboo exterior coatings [174]. Strong synergistic effects were detected in the BTZ–ZnO coatings, especially for the 2:1 ratio formulation. The coating system provided high resistance to photodegradation and effectively inhibited photodiscolouration of the bamboo substrates. Another mixture of benzotriazoles, hindered amine light stabilizers (HALS), and ZnO nanoparticles in thick-film waterborne acrylic coating also gave the most positive effect in UV protective surface modification when applied to oak wood [175]. The mixture of benzotriazoles, HALS and both TiO2 and ZnO nanoparticles was confirmed as one of the most effective treatments for colour stabilisation of wood due to UV and VIS spectrums. It was reported that wood specimens coated with rutile TiO2 and a mixture of methyltrimethoxysilane and hexadecyltrimethoxysilane showed superior weathering performance and improved resistance to surface colour change and weight loss [176]. The TiO2 coating also was found to apparently enhance the colour stability of wood during UV light irradiation without water spray. However, the adjacent wood surface degraded because of the photocatalytic activity of TiO2 [177].
Nanoadditive for Fire Retardancy Improvement
The flammability of wood and non-wood products has restricted utilisation, with fire safety being a major concern for the various applications. To overcome the inherent deficiencies and use of wood and non-wood in a safe manner, the flame retardant properties need serious consideration. Nanoparticles have recently been used to produce the nanocomposites for the improvement in fire retardant properties. The utilisation of nanoparticles, either alone or in combination with conventional fire retardants, serves to reduce the ignitability of wood. The nanosize and high surface area of nanomaterials make them more effective at low concentrations than other conventional compounds which are an enormous advantage industrially and economically. The surface modification is necessary for nanoparticles to achieve better compatible and homogeneous dispersion. The TiO2 coated wood was found to be capable in reducing the flammability of the wood and the spreading of the flame, as compared to the uncoated sample [178]. The ZnO–TiO2-layered double-nanostructures had been synthesised on a bamboo substrate [179]. The findings showed that the oxygen index increased from 25.6 to 30.2% after being covered with a ZnO–TiO2 coating, which revealed a significant enhancement of its flame retardant property. Layered double hydroxides [180] can absorb a large amount of heat, dilute the concentration of flammable gas, and absorb harmful acid gases during the decomposition process; therefore, it is an excellent flame retardant.姚等人。 [180] applied nanomagnesium aluminium layered double hydroxide (Mg–Al LDH) to bamboo in an in situ one-step process and found that the total heat release and total smoke production were reduced by 33.3% and 88.9%, respectively, compared to those of samples without Mg–Al LDH.王等人。 [181] introduced zinc-aluminum layered double hydroxide (Zn–Al LDH) nanostructures to wood and found that the peak heat release rate (PHRR) and total smoke production were reduced by 55% and 47%, respectively, compared to those of the pristine wood [181]. Nanostructured carbon materials such as graphene was also proven to have a great potential to be used as an effective fire retardant in wood and wood-composite materials for surface protection against fire [182].
Wood Durability
Wood is such a versatile material that finds its use in various fields like construction, furniture and artwork [183,184,185]. Wood is applied as a construction material due to its high strength-to-weight ratio, eco-friendly characteristic, aesthetic appearance and biodegradability feature. Unfortunately, wood is very sensitive to biological attacks, especially by decay fungi and insects [186, 187]. Wood also gets affected by exposure to UV-radiation, fire and moisture [188]. Moisture can cause wood warping, cracking and dimensional instability. The wood degradation can cause aesthetical and internal structural damages. The degradation of wood leads to immeasurable losses each year. Besides, the growth of decay fungi on wood structural will trigger health problem to human including allergies, respiratory symptoms and asthma especially after prolonged indoor exposures [189]. Therefore, the associated issues related to wood cannot be simply overlooked. Preservation of wood using chemical is the effective way to protect and prolong the service life of wood.
Over the last decades, many chemical preservatives have been developed and used to protect wood against biodegradation agents [188, 190]. Unfortunately, most of these chemical preservatives may pose serious effects to human, living organisms and environment due to their accumulation in soil and ecosystems [191]. Chromated copper arsenate (CCA) is one of the chemical preservatives widely used since the middle 1930s and effectively protects wood against decaying fungi, termite and insect borer. However, CCA was shown to be toxic to human and environment [192,193,194]. A similar issue is also faced by another chemical, i.e. pentachlorophenol (PCP). It was considered to be hazardous to human health; thus, its production and uses were banned in many countries [195].
In response to these issues, a new series of chemical preservatives claimed as safe and less-polluting are being introduced. These chemical preservatives can be obtained from plant extract or produced synthetically and have plant bioactive compound properties. For instance, pyrethroids, derived from pyrethrum flower (Chrysanthemum cinerariifolium ), have a potent insecticidal activity and can now be produced synthetically. Unfortunately, these chemicals were found easily degradable by light and temperature, and have narrow efficiency spectrum [196]. Other limitation of organic pesticides is that most of them dissolve in organic solvents and cannot be formulated as water-based wood preservatives. Therefore, smart and intelligent organic pesticides delivery system is crucial. Through a smart delivery system, biocides can be delivered in a controlled and targeted manner. This will reduce hazards to human and environment [17].
Wood treatment via nanotechnology method can improve wood durability against biodegradation agents and weather [14, 197, 198]. The main advantage of applying nanoproducts to wood is its unique ability to penetrate deeply in wood structure [14, 199], thus improving durability properties that result in a long life time service. On the other hand, complete penetration with uniform distribution of nanoproducts can also be achieved [200]. To date, many ready-to-use nanoproducts (nanopreservatives) for wood protection are available in the market, while some of them are still in the research and development stage. Generally, nanoproducts used to protect wood can be classified into two types, namely nanocapsules and nanomaterials. Nanocapsule refers to the pesticides embedded in a polymeric nanocarrier, while nanomaterial is nanosized metals which can be directly impregnated into wood [201].
Biocide Enhancement Property via Nanoencapsulation
Encapsulation of pesticides into polymeric nanocarriers is one of the promising nanotechnology techniques in improving the impregnation of wood with pesticides. This technique is designed to increase the solubility of poorly water-soluble pesticides and to release pesticides in a slow manner [202]. Encapsulation allows those low solubility pesticides to disperse easily in solid polymeric nanoparticles. The polymer can then be suspended in water and applied to wood via conventional water-based treatments [149]. Encapsulation is also able to protect the hydrophilic active ingredient from excessive leaching [19]. Due to the small diameter of capsule, it can be easily incorporated and penetrated into the cell wall of the wood. This accordingly improves the durability of treated wood against biodegradation agents. Not only this technology can deliver pesticides safely, it can also prolong the pesticide lifespan, resulting in extended protection for the wood [203].
Encapsulation of pesticides is a bottom-up approach. It can be carried out through several techniques such as nanoprecipitation [17, 204,205,206], emulsion-diffusion [207] and double emulsification [208] (Table 3). The materials used as polymeric nanocarriers can be derived from natural polymer, synthetic polymer or combination of both [209]. For instance, natural polymer includes cellulose, starch, alginates, silica and halloysite. The synthetic polymer usually used as polymeric nanocarriers includes poly(vinyl acetate), poly(methyl methacrylate), poly(lactic acid), etc. (Table 3).
图>A previous study by Liu et al. [18, 216] successfully encapsulated tebuconazole and chlorothalonil into polyvinylpyridine and polyvinylpyridine-co-styrene using the impregnation method. The particle size of capsules obtained was between 100 and 250 nm. They impregnated a suspension of the capsules into sapwood of southern yellow pine and birch using conventional pressure treatments. The treated wood was then exposed to brown-rot (Gloeophyllum trabeum ) and white-rot (Trametes versicolour ) fungi for 55 days. The results showed great resistance against both decay fungi.
Salma et al. [17] used the nanoprecipitation technique to encapsulate tebuconazole. The polymer capsules containing tebuconazole were prepared from amphiphilic copolymers of gelatine grafted with methyl methacrylate with the size diameters ranging from 200 to 400 nm or 10 to 100 nm depending on core/polymer shell mass ratio. The encapsulated tebuconazole was reported to be able to preserve wood against a brown rot fungus. On the other hand, the formulation system developed by Salma et al. [17] is flexible and can be easily modified using copolymerisation of other acrylic monomers like hydroxyethyl methacrylate. This indicates that tebuconazole release rate can be tailor-made. However, the disadvantage of this nanocapsules is that they are prone to aggregation, which reduces the delivery efficiency of the nanocapsules into the wood.
Can et al. [217] successfully encapsulated nano silver into polystyrene-soybean co-polymer. In their study, the Scots pine was impregnated with the capsules and tested against white-rot fungi (Trametes versicolor )。 The finding obtained from the study indicates that the soybean oil, polystyrene and nanosilver played important roles in the synergistic effect of increasing the decay resistance of Scots pine.
Impregnation of Metallic Nanoparticles for Durability Enhancement
Metallic nanoparticles have been used to protect wood against biodegradation agents and weathering since decades ago [197, 218, 219]. Nanoparticles offer better characteristics than their bulk form, mainly because of their reduced size that leads to high specific surface area-to-volume ratio, uniform size distribution and good stability. Due to its very small size, nanoparticles can penetrate deeply and uniformly into the wood pores leading to a protection of wood [197, 220]. In addition, dispersion stability is improved because of the size and also by addition of surfactant [221]. Dispersion stability coupled with small particle size may greatly improve the following aspects:(1) preservative penetration, (2) treatability of wood, (3) stability of finishes and coating products, (4) low viscosity, and (5) non-leachability [222, 223]. Besides, it can enhance compatibility with binders thereby being able to increase the affinity with wood polymers [197, 224].
Metallic nanoparticle can be prepared by altering the particulate size of metal via chemical reactions, mechanical treatment, heating or refluxing. To date, a lot of nanoparticles have been used for wood protection. Metallic nanoparticles mainly copper, silver, boron and zinc exhibited a good performance against white-rot and brown-rot but less efficient against mould [219]. Titanium dioxide (TiO2) is another nanoparticle that has a good potential to be used as wood preservative. The potential of TiO2 is related to its antibacterial and antifungal [14, 225] and UV-resistant properties [225, 226]. However, the study on wood treated with TiO2 is still under preliminary investigation [14, 225]. Table 4 lists the works on the utilisation of TiO2 for wood preservation. The distribution of TiO2 in wood was studied by Mohammadnia-Afrouzi et al. (Fig. 3).
图>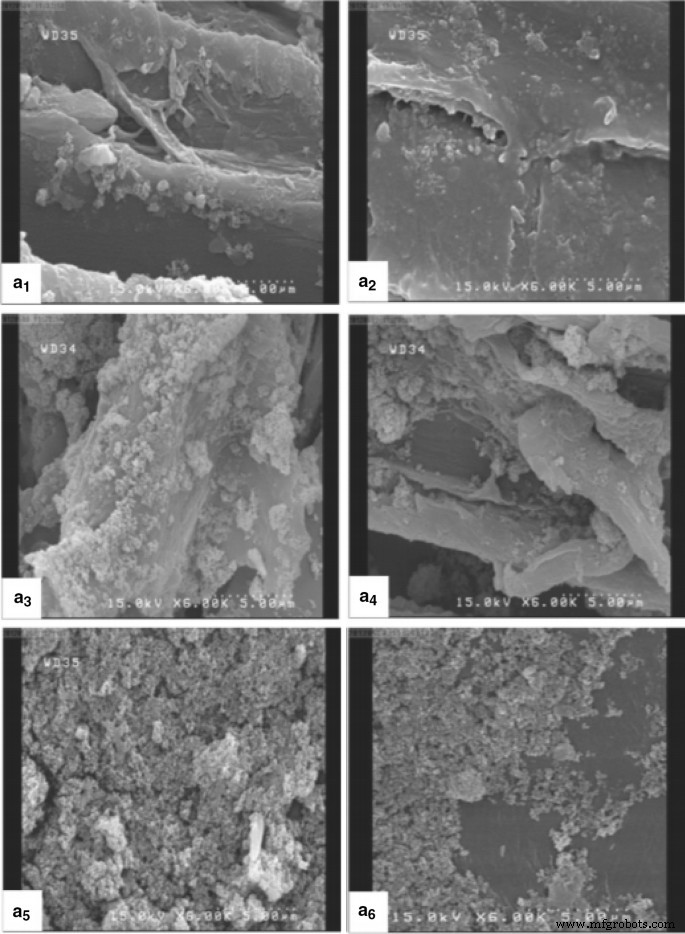
SEM images taken from the tangential section of nano-TiO2 wood treated samples:the 0% moisture content (MC)/0.5% concentration (a1), the 25% MC/0.5% concentration (A2), the 0% MC/1% concentration (A3), the 25% MC/1% concentration (A4), the 0% MC/1.5% concentration (A5) and (25% MC/1.5% concentration. Source :Afrouzi et al. [227]
图>Copper is an essential biocide for wood protection. However, copper alone fails to protect wood against copper-tolerant wood destroying fungi. Copper nanoparticles is a new generation of wood preservative-based copper. The use of copper nanoparticles instead of conventional copper shows improved durability of wood against decay fungi [230]. This shows that copper nanoparticles can be used to protect wood without the presence of chromium and arsenic [230].
Cristea et al. [15] studied the effects of the addition of ZnO nanoparticles and silver nanoparticles into exterior wood coatings. The purpose of the study is to improve the durability and wood protection through UV shielding. Besides providing an efficient protection against UV, the mechanical properties of wood such as hardness, adhesion strength, the abrasion resistance and the barrier effect for water vapor diffusion were slightly improved.
The mixture of ZnO nanoparticles with silver nanoparticles was able to protect wood from weathering problem such as UV rays [231]. They impregnated sapwood of cottonwood using three different concentrations of mixture by full-cell process. The samples were then exposed to natural weathering. The colour changes of treated wood samples were measured using spectrophotometer. The wood treated with ZnO nanoparticles alone was used as a control. The finding indicates that wood treated with the mixture of ZnO nanoparticle and silver nanoparticles has the lowest colour changes compared to the samples treated with each metallic nanoparticles.
In another study, Mantanis et al. [197] treated black pine wood with ZnO, zinc borate and copper oxide nanoparticles under vacuum. They used acrylic emulsion to force the metallic nanoparticles into the wood structure to avoid leaching. The durability of treated wood against mould decay fungi and subterranean termites was evaluated. Results showed that wood treated with zinc borate slightly inhibited the mold, while the other metallic nanoparticles did not exhibit mould. A similar finding was reported by Terzi et al. [232] that ZnO nanoparticles did not exhibit the mould growth on wood specimens. However, all metallic nanoparticles significantly inhibited white-rot fungi and termite.
Another study shows that metallic nanoparticles are able to improve fire retardant properties of wood. Francĕs et al. [16] studied the effect of SiO2, TiO2 and ZnO2 infiltrated into pine veneers. They reported that veneer treated using 3 wt% of SiO2 was most effective to improve the fire retardant behaviour.
Despite the remarkable advantages of nanotechnology in the wood preservation sector as discussed above, the fundamental understanding on synthesis, processing and characterisation of nanocapsules and metallic nanoparticles for wood protection still needs to be improved. The most interesting characteristics need to be considered during the design and development of nanocapsules or metallic nanoparticles for wood protection are as listed in Table 5.
图>Potential of Nanocellulose-Based Material in Energy Sector
Energy is an important resource that has a strong correlation between economic growth and development [233]. Today, the main energy sources are from fossil fuels and hydroelectric sources, which are very harmful to environment because they can cause climate change and global warning as well as ozone layer depletion, pollution, greenhouse gases emission and ecological destruction [234,235,236]. About 80% of carbon dioxide (CO2) emissions in the world are from energy sector and technology advancement is required to develop sustainable renewable energy resources to reduce the CO2 emissions as well as to overcome the global warming impact on life and health in line with the needs of accelerating technology development [234, 237].
In order to minimise the environmental effects, a sustainable and low-cost energy efficient carbon-based material has been explored as a potential to replace some conventional materials in the fabrication of energy devices. One of the natural carbon-based materials is cellulose which is the most promising natural polymer with many usages, including energy [237].
Nanotechnology is one of the advanced technologies that have the potential and prospect to fulfil the demand to create clean and green energy. Developing this new material in nanoscale enables new application and its interaction with current energy technology that would revolutionise the energy field from usage to supply, conversion to storage and transmission to distribution [238]. By adapting this nanotechnology, it will have high impact on the development of clean and green energy and benefits the environment and natural resources [239].
According to Serrano et al. [234] and Hut et al. [235], most promising application of nanotechnology for energy conversion is mainly focused on solar energy, conductive materials, solar hydrogen, fuel cells, batteries, power generation and energy devices. Understanding the structural and morphological properties of nanomaterial is essential to obtain the proficiency and sustainability for many applications. The greatest application of nanotechnology in energy generation is solar energy using photovoltaic (PV) cells which focuses on harnessing efficiency [233]. Consumption of energy generated from this solar cell using natural resources will reduce the usage of fossil fuel and decrease the pollution towards creating environmentally friendly and green energy [239]. In addition, the development of nano devices using solar cell could improve the existing materials efficiency as well as reducing manufacturing cost that might increase the economic growth [236].
Nanotechnology has been used in various applications to improve the environment, to solve humanity problems and to produce more efficient and cost-effective energy, such as generating less pollution during products manufacturing, producing solar and fuel cells at a competitive cost, hydrogen production, cleaning up organic chemicals polluting groundwater and overcoming the problem of energy sufficiency, climate change and diseases as well as to reduce the dependency on non-renewable energy sources [233, 235, 240].
Nanocellulose-Based Material for Solar Energy
Solar energy is available in various parts of the world and can be captured from the sun with 15,000 times more energy yearly. This energy source can be used in different ways:photovoltaic (PV) technology, solar thermal systems, artificial photosynthesis, passive solar technologies and biomass technology, which are used to produce electricity, steam or biofuels [234, 235].
In future, nanotechnology might contribute to develop an effective and low-cost system for production, storage, and transporting of energy [235]. According to Serrano et al. [234] and Hut et al. [235], current photovoltaic (PV) market is based on silicon wafer-based solar cells (first generation) and thin film layers of semiconductor materials (second generation). Current drawback of using solar cells is the cost of manufacturing mainly on the high cost of conventional PV cells with poor energy absorption efficiency (less than 40%) [233].
Nanocellulose shows a good potential to be used in the solar energy system due to its renewability, biodegradability, biocompatibility, broad modification capacity, adaptability and versatile morphology [241, 242]. Low cost, flexible and porous substrate of cellulose could be used to produce solar cells. Nanofibrillated cellulose (NFC) with size as low as 4 nm could become the excellent candidate for production of ultrathin paper for use in solar cell component to store the energy [235]. Klochko et al. [243] used nanocellulose from biomass for the development of biodegradable eco-friendly flexible thin film as a thermoelectric material. The thin film was used to convert low-grade waste heat from sun radiation into electricity at near-room temperature.
Nanocellulose-Based Conductive Materials
Conductive materials allow the flow of electrical current which is needed in the fabrication of energy devices. There are many types of conductive materials such as conductive polymers and conductive carbon materials (e.g. carbon nanotubes, graphene, and carbon black) and metallic particle with different levels of conductivity. These conductive materials can be combined with nanocellulose to form novel composites. The process of production conductive nanocellulose is shown in Fig. 2. There are two major strategies involved in nanocellulose based conductive hybrids fabrication process; one is coating of conductive materials layer on the surface of nanocellulose substrates, and another one is mixing the conductive materials inside the nanocellulose substrate to make composite [237]. Conductive polymers are an alternative to metallic materials because of their good electrochemical performance, light in weight and low cost. One of the most promising conductive polymers is polyaniline (PANI) because of its simple route of synthesis, controllable conductivity and high specific capacitance [244].
Nanocellulose-based conductive materials are developed for supercapacitors and energy storage device applications using various types of method such as in situ polymerisation, doping, coating, inkjet printing and in situ depositing [68, 244,245,246]. Modification of the existing supercapacitor by adding nanocomponents has increased its ability to store large amount of energy with longer time of supply [238].
Besides that, nanocellulose-based composite membrane electrodes can be developed via in situ polymerisation of nanocellulose using conductive components via a simple filtration unit (Fig. 3). A well-mixed conductive materials/nanocellulose composite membrane is left on the filter after the liquid has passed through the filter and air-dried composite membrane can be peeled off from the filter membrane for further use as supercapacitors [244].
Nanocellulose for Energy Storage
The potential application of nanocellulose for energy storage application has gained much attention recently. This is due to its nanoscale dimension, high surface area-to-volume ratio, and rich with hydroxyl group, which make their surface chemistry easily modifiable for composite processing. The most important aspect in energy storage is to develop nanocellulose with conductivity and flexibility properties. It can be achieved by adding conducting polymers such as polyaniline and polypyrrole. For example, the nanocellulose/polyaniline composite film is widely used as paper based sensors, flexible electrode, and conducting adhesive [68, 247,248,249]. Razaq et al. [250] manufactured electrodes from the composite of nanocellulose/polypyrrole and carbon filament for paper based energy storage devices.王等人。 [248] reported that their devices which developed using composite of nanocellulose/polypyrrole provide high charge and discharge rate capabilities, high cell capacitances, and cycling performance.
Nanocellulose-Based Materials for Lithium and Vanadium Battery
High demand on flexible portable electronic devices recently such as smart phone, electrical vehicles, laptops, and even the grid energy storage causes increasing demand on rechargeable lithium-ion battery (LIBs) [251] and supercapacitors [252]. LIBs are one of the most ideal energy storage candidates for electronic devices, due to their high energy density, moderate power density and cycle stability. In LIBs, electrolyte is important for lithium-ion (Li + ) transfer between anode and cathode. Organic liquid electrolyte is used for LIBs system, but it can pose tremendous safety concern due to the high toxicity and flammability. Solid-state electrolyte has become of interest because it demonstrates obvious advantages of low flammability and low toxicity [253, 254]. According to Janek et al. [255], solid-state electrolyte is classified as solid polymer electrolyte (SPE) and inorganic solid electrolyte (ISE). However, SPE offers the advantages of easy processing and flexibility [255].
秦等人。 [256] developed SPE by incorporating polyethylene oxide (PEO) with nanofibrillated aerogel and bis(trifluoromethanesulphonyl)imide lithium salt (LiTFSI). The results showed that the ionic conductivity properties of SPE were significantly enhanced due to the negatively charged nanofibrillated cellulose. The results also proved that the fabricated SPE is electrochemically stable, mechanically robust and thermally stable as well as flexible, expected for use in flexible electronic devices.
Nair el at. [257] fabricated nanocellulose-laden composite polymer electrolyte for high performing lithium-sulphur batteries using a thermally induced polymerisation method. The composite polymer electrolyte demonstrates excellent ionic conductivity, thermal stability up to more than 200 °C and stable interface towards lithium. The electrolyte also has stable cycling profiles which are attributed to significant reduction of the migration of polysulphide towards anode by entrapment of nanocellulose in the polymer matrix.
Another study was carried out by Zhang et al. [258] on robust proton exchange membrane developed using sulphonated poly(ether sulphone) reinforced by core–shell nanocellulose for vanadium redox flow batteries (VRFBs). It was found that with the incorporation of silica–encapsulated nanocellulose, the proton exchange membrane exhibits outstanding mechanical strength of 54.5 MPa and high energy efficiency above 82% at 100 mA cm −2 , which is stable during 200 charge–discharge cycles. Proton exchange membrane is one of the key components in VRFBs. It functions as the separator to avoid vanadium ions crossover. It also acts as proton conductors that contribute to high voltage efficiency in VRFBs [259].
Nanocellulose-Based Materials for Flexible Supercapacitor
Supercapacitor or known as electrochemical double-layer capacitor or ultracapacitor is another versatile energy storage system that has gained the attention of researchers worldwide [260]. With the high power density, long life cycle, simple principles, low maintenance, portability, stable performance and fast charge/discharge rate make supercapacitors capable of filling the gap between batteries and conventional capacitors [260]. These properties offer a promising approach to meet the growing power demands.
Electrode is a very important component in supercapacitor. It requires good electrochemical performance and flexibility especially for preparing a high-performance flexible supercapacitor. Him et al. [261] and Zhe et al. [262] found that graphene and nanocellulose are excellent flexible electrode materials for supercapacitors. Nanocellulose has been used as a substrate material because of its good biodegradability, mechanical strength, flexibility, and chemical reactivity. The porous structure and hydrophilicity of nanocellulose can facilitate the attachment of other materials for example graphene in their fibrous network structure [263]. At the same time, the abundance of hydroxyl groups on the nanocellulose surface enables the interaction of nanocellulose with other polymer to form strong composites [264].
Khosrozadeh et al. [265] developed an electrode for supercapacitor using nanocellulose-based polyaniline/graphene/silver nanowire composite and after applying it for 2400 cycles, at a current density of 1.6 A/g, the supercapacitor showed a power density, energy density and capacitance of 108%, 98% and 84%, respectively. This shows that the electrode has an excellent cyclic stability and good mechanical flexibility. On the other hand, Ma et al. [266] developed an electrode using bacteria cellulose/polypyrrole coated with graphene. The prepared electrode has a good mechanical flexibility in which it can bend at any angle. The area capacitance and energy density can reach 790 mF cm −2 and 0.11 mWh cm −2 , respectively, when assembled into symmetric supercapacitors. The nanocellulose-graphene electrode can be fabricated using chemical cross-linking or physical cross-linking method [267, 268]. Nanocellulose as an electrode component of supercapacitor also plays the role of internal electrolyte reservoir. This is because nanocellulose can provide an effective way for ion transport. The high pore structure and hydrophilic properties of nanocellulose make it easier to transport electrolyte ion [261].
Nanocellulose-Based Paper for Electronic Devices
Nanocellulose-based paper is a green substrate that can be used for electronic and optoelectronic devices. Currently, commercial paper has relatively rough surface and weak mechanical properties which can be quite problematic for electronic device fabrication [269]. Most of the electronic device’s fabrication use non-biodegradable and non-recyclable component such as plastics, glasses and silicones as substrates [270]. Formation of nanocellulose-based paper from NFC via simple filtration method can produce mechanically strong and low coefficient thermal expansion paper as an alternative to commercial paper [6, 269]. According to Li and Lee [270], transparent nanocellulose-based paper for electronic devices has been designed and being applied for electrochromic, touch sensor, solar cells, transistors, organic light-emitting diodes (OLEDs), gravure printing proofer and radio-frequency identification (RFID).
One of the applications of transparent nanocellulose paper is for the production of flexible electronics materials through printing circuit directly on the surface of substrates via coating or thermal deposition techniques. Firstly, the fabrication of flexible electronics was built on special silicon wafer that allows the silicon nanomembranes (Si NMs) to be released and then transferred to NFC substrate with an adhesive layer, and the device was completed by photoresist patterning and dry etching steps as illustrated in Fig. 4 [271].
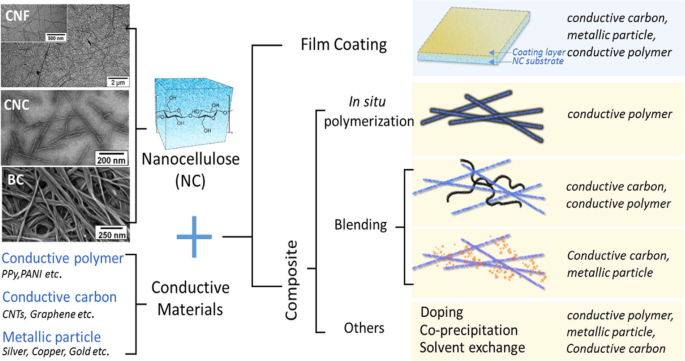
Schematic illustration of the generalised fabrication routes to nanocellulose-based conductive hybrid. Source :Du et al. [237]. Reproduced with permission of Elsevier
图>Moreover, NFC also is the main substrates for organic light-emitting diodes (OLEDs) (Fig. 5). Okahisa et al. [272] reported that the OLEDs device was fabricated on wood-based nano fibrillated cellulose (NFC) composite.
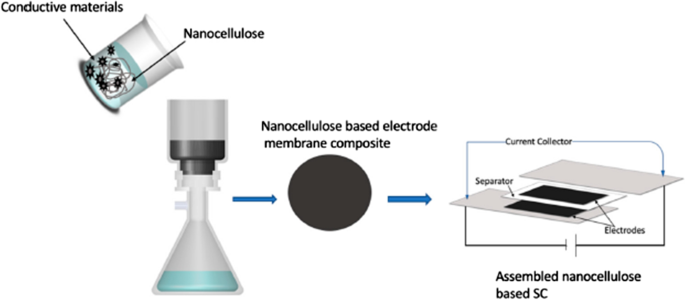
Filtration procedure to fabricate nanocellulose-based composite membrane [supercapacitor (SC)]. Source :Hsu and Zhong [244]
图>Potential of Nanocellulose-Based Composite for Development of Sensor
Nanocellulose has been widely used to develop novel sensors and improve the sensitivity of sensors. In food industry for example, sensors have become an important tool to protect humans from health hazards and risks caused by the food contaminants. Sensors can help to quickly identify mycotoxins, pathogens, heavy metals, pesticides, metal ions, and so on in food. In addition, sensor technology can overcome the complicated, laborious, and time consuming process using expensive instrument that usually requires well-trained personnel [273, 274]. Sensor also provides rapid and sensitive food safety detection.
In the last decade, plenty of sensors (electrochemical sensors, biosensors and chemical sensors) have been successfully developed as alternative or as complementary detection tools for the rapid and sensitive detection [275,276,277]. However, conventional sensors were developed using plastics, petrochemical-based products and inorganic material which lead to environmental problems. Moreover, issues such as green gas emission, toxicity and sustainability of the materials are becoming increasing. Therefore, the demand for sustainable sensor devices has increased rapidly in recent years.
Nanocelluloses from plants and bacteria have shown promising potentials due to their excellent physical, thermal, mechanical, optical and physical properties, which are important for fabricating high-performance sensor devices. These properties make nanocellulose more preferred as biomaterials since it can enhance the selectivity and sensitivity of sensors for the detection of analytes. On the other hand, the adhesion properties of nanocellulose prevents the leaching problems of immobilised reagents. Thus a remarkable improvement can be obtained for the long-time stability of the sensor. Besides, the hydroxyl –OH groups on nanocellulose can be modified for the incorporation of binding sites for the selective adsorption of different analyte species [278]. The combination of those characteristics makes the nanostructured nanocellulose fibres an ideal building block for conjugation with other functional materials [248, 279, 280] (Table 6).
图>Development of Biosensors
金等人。 [286] immobilised enzymes candida rigosa lipase into the different cellulose nanocrystals. The findings indicate candida rigisa lipase absorbed on nanocellulose was relatively higher compared to that of microcellulose. This can be related to the high surface area on nanocellulose and thus increase the ionic interaction between nanocellulose anionic group and candida rigisa lipase. In this study, they found that the half-life of the candida rigisa lipase immobilised in nanocellulose increased 27 times higher compared to in free form. Besides, the stability of enzyme also increased (Fig. 6).
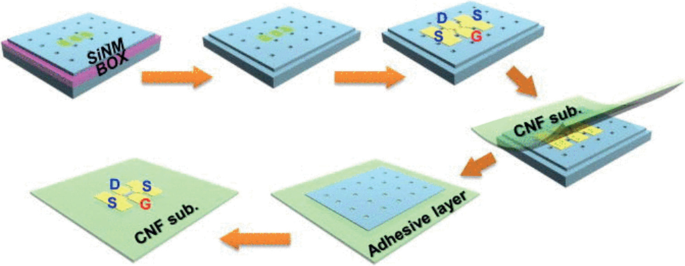
Flexible electronics production process on NFC substrate. Source :Sabo et al. [271]
图>Another group of researchers, Edwards et al. [287] studied the kinetic profiles of tri- and tetrapeptides substrate of elastase for the fabrication of elastase biosensor whereby known as human neutrophil elastase (HNE) and porcine pancreatic elastase (PPE). To develop HNE and PPE, immobilised tri- and tetrapeptides were used into cotton cellulose nanocrystals. They found that 2 mg of tripeptide conjugated cotton cellulose nanocrystals in 1 h was able to detect 0.03 U mL −1 PPE, while 0.2 mL tetrapeptide conjugated cotton cellulose nanocrystals over 15 min could detect 0.05 U mL −1 HNE activity. Incani et al. [288] fabricated a biosensor by immobilising glucose oxidase (GOx) enzyme in a nanocellulose/polyethyleneimine (PEI)/gold nanparticles (AuNPs) nanocomposites. The AuNPs were adsorbed on the cationic PEI and nanocellulose. The Fourier Transform Infrared (FTIR) spectra confirmed that GOx was successfully immobilised on the polymer composites (Fig. 7).
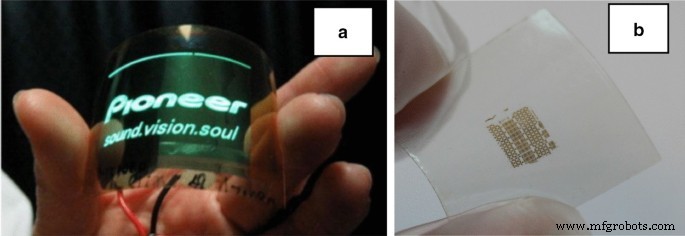
Flexible display (OLEDs) on NFC substrate, a Source :Okahisa et al. [272]. Reproduced with permission of Elsevier. Flexible electronics on NFC substrate, b Source :Sabo et al. [271]
图>Abd Manan et al. [289] successfully developed biosensor based on nanocrystalline cellulose (NCC)/cadmium sulphide (CdS) quantum dots (QDs) nanocomposites for phenol determination. They modified the NCC with cationic surfactant of cetyltrimethylammonium bromide (CTAB) and further decorated with 3-mercaptopropionic acid (MPA) capped CdS QDs as a scaffold for immobilisation of tyrosinase enzyme (Tyr). The TEM images of NCC and CTAB-NCC (Fig. 8a, b) indicates that agglomerated whiskers-like structure of NCC was not affected by modification, while the MPA QDs are of spherical shape (Fig. 8c). The FESEM images of CTAB-NCC nanostructured film (Fig. 8d) exhibited a homogenous, uniform and dense fibrous structures aggregated, while for CTAB-NCC/QDs nanocomposites film (Fig. 8e), the CdS QDs were appeared as like tiny white dots. EDX analysis shows the presence of respective elemental of carbon (C), oxygen (O), sulphur (S) and cadmium (Cd), indicating the CdS QDs was successfully attached into the CTAB-NCC film. The test finding against phenol shows the biosensor exhibits good linearity towards phenol in the concentration range of 5–40 µM (R2 = 0.9904) with sensitivity and limit of detection of 0.078 µA/µM and 0.082 µM, respectively.
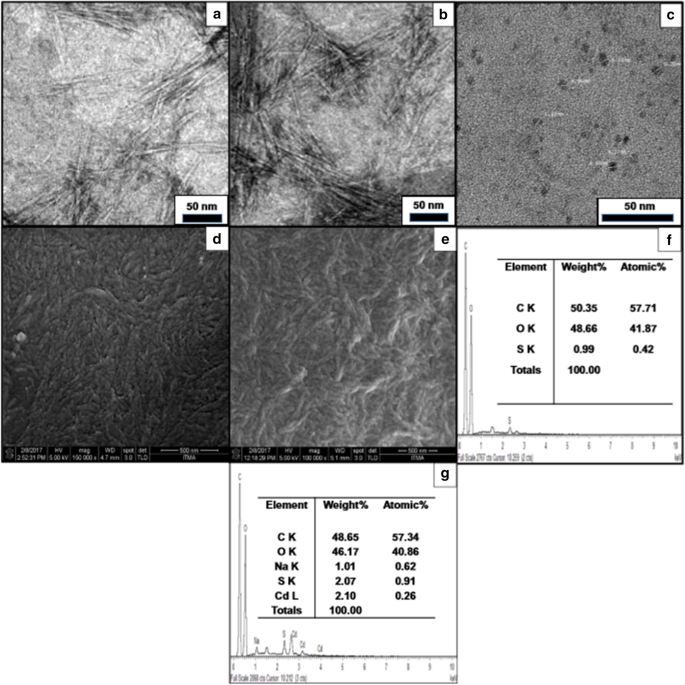
The TEM images for a NCC, b CTAB-NCC, c MPA-QDs, the FESEM micrograph of d CTAB-NCC nanostructures film, e CTAB-NCC/QDs nanocomposites film, f EDX analysis of CTAB-NCC nanostructured film, g CTAB-NCC/QDs nanocomposite film (Source :Abd Manan et al. [289]). Reproduced with permission of Elsevier
图>In the food industry, sensors are used to monitor the quality or freshness of food.莫拉迪等人。 [290] developed a pH sensing indicator based on bacterial cellulose nanofibres for monitoring freshness of fish. They used carrot anthocyanins as an indicator. The fabricated sensor displayed wide colour differences from red to gray over the 2–11 pH range. The colour changes were distinguishable whereby deep carmine colour indicates fresh fish, charm pink colour indicates fish is best to eat immediately, and spoiled fish is indicated by jelly bean blue and khaki colours.
Development of Chemical Sensors
Nanocellulose hydrogels structure was previously used to immobilise sulphur and nitrogen co-doped graphene quantum dots as a low-cost sensor for detecting the laccase enzyme [291]. Laccase is the multicopper oxidase family of enzyme. It is involved in the monoelectronic oxidation of several aromatic compounds and aliphatic amines. Therefore, it is usually used in decolouration and coloration products [292, 293]. Therefore, monitoring of laccase activity in commercial products is of great interest. From this study, the authors found the sulphur and nitrogen co-doped graphene quantum dots were self-organised via electrostatic interactions.
Faham et al. [294] described the development of a nanocellulose-based colorimetric assay kit for smartphone sensing of iron, Fe(II) and iron-chelating deferoxamine drug (DFO) in biofluids. They embed curcumin in a transparent bacterial cellulose nanopaper, as a colorimetric assay kit. The assay kit was then used for monitoring of iron and deferoxamine as iron-chelating drug in biological fluid such as urine, blood, saliva and serum. The assay kit can be easily coupled with smartphone technology for colorimetric monitoring of Fe(II) and DFO.
Development of Electrochemical Sensors
Burrs et al. [295] demonstrated the electrochemical biosensor-based glucose for the detection of pathogenic bacteria. The conductive paper used for the development of electrochemical sensor was prepared using graphene-nanocellulose composites. Platinum was electrodeposited on graphene-cellulose composites using pulsed sonoelectrodeposition [296]. Then the sensor was fabricated by functionalising the nanoplatinum with glucose oxidase (GOx ) entrapped in a chitosan hydrogel on the conductive paper. They found that the sensor is extremely efficient for use in electrochemical biosensing with a low detection limit for glucose or pathogenic bacteria.
Ortolani et al. [297] developed an electrochemical sensing device using nanocellulose and single-walled carbon nanohorns (SWCNH) for guanine and adenine determination. Both nanocellulose and SWCNH have large surface area, good conductivity, high porosity and chemical stability. The prepared sensor showed highly sensitive and high electrocatalytic activity towards simultaneous determination of guanine and adenine. The sensor also presented a lower limit detection. Another study conducted by Shalauddin et al. [298] used hybrid nanocellulose/functionalised multi-walled carbon nanotubes (f -MWCNTs for development of electrochemical sensing. The sensor for determination of diclofenac sodium in pharmaceutical drugs and biological fluids samples were used. The presence of –OH groups in the nanocellulose was reported to provide more binding sites for different analytes which ensures an axial modulus rearrangement and incorporation of f -MWCNTs.
Toxicity of Nanomaterial
Although nanomaterial offers many good potential to various industrial sectors, its potential hazard to human and environment could not simply be overlooked [299]. The safety or toxicity issue needs to be addressed by conducting a complete study from various angles. Nanotoxicity or the study of nanomaterials’ toxicity can be classified into several areas namely oxidative stress, genotoxicity, and ecotoxicity. In humans, toxicity can occur through pulmonary, oral and dermal routes. Nanomaterials can affect humans mainly during extraction or production, handling, usage and disposal. Inorganic nanoparticles (NPs) such as ZnO and nickel oxide (NiO) are believed to be more toxic compared to organic nano materials such as nanocellulose. Digested organic NPs are less likely to induce toxicity since the by-products of digestion by stomach acid are simple sugars, although in the case of nanocellulose, the lack of enzyme in human gut capable of degrading cellulose results in the cellulose moving through the gut fairly quickly. A 90-day subchronic toxicity study on Sprague Dawley rats conducted by Ong et al. [300] indicated that no systemic toxicity attributable to 4% dietary consumption of fibrillated cellulose was observed. In the same study, a higher concentration of 30% of nanofibrillated cellulose for 72 weeks also indicated no adverse effects. When present in the air, nanoparticles can also have harmful effects to human health [301]. Multi-walled carbon nanotubes (MWCNT) are possibly one of the most studied NPs for toxicity effects. In the study, Poulsen et al. [302] found that both thick and long as well as thin and short MWCNT induced genotoxic potential and long lasting inflammation and can lead to cardiovascular disease. In contrast, a study on inhalation NCC toxicity in albino rats was conducted and showed no undesirable effects were observed at a maximum concentration of 0.26 mg/L for a period of four (4) hours [303]. At cellular level, cytotoxicity on NCC on different cell types has been studied with most studies concluded that NCC to be non-toxic to a variety of mammalian cells at concentrations below 0.25 mg/L, but can exhibit cytotoxic effects at concentrations of 0.5 mg/L and above [304,305,306,307,308,309]. Primary nanogenotoxicity refers to the possibility of nanoparticles or their by-products to damage DNA directly or indirectly. Secondary genotoxicity is caused by immune system interaction to disturbances cause by nanoparticles. A study by Akerlund et al. [310] indicated that inorganic NPs of Ni and NiO-induced cytokines inflammations which led to secondary genotoxicity in human lung epithelial cells. However, they require further investigation to understand the factors causing this secondary genotoxicity and whether such results are caused by other nanoparticles as well.
NanoTiO2 in wood coatings has been reported to have low toxicity to terrestrial organisms, and no bioaccumulation was observed [311]. Another study conducted on cerium oxide (CeO2) nanoparticle or ceria showed that no acute toxicity was observed for Daphnia magna and Thamnocephalus platyurus and D. rerio embryos at test concentrations of 1000, 5000, and 200 mg/L, respectively. However, the same study indicated that a significant chronic toxicity on P. subcapitata was observed with 10% effect concentrations (EC10s) between 2.6 and 5.4 mg/L [312]. Pulido-Reyes et al. [313] explained that the redox cycling between Ce 3+ and Ce 4+ is a unique chemistry of nanoceria which depends on the prevailing environmental conditions. They concluded that in most cerium oxide NP studies, the amount of surface Ce 3+ correlates with toxicity and this can be overcome by blocking the Ce 3+ sites with anions such as phosphates (PO4 3− ) which will reduce reactive oxygen species levels and overcoming oxidative stress. During and after disposal, there is a likelihood that NPs can interact with the environment, whether in soil or aquatic environment. The oxidative stress or carbon-based nanoparticle-induced toxicity is primarily of concern as this condition will affect the oxygen balance in the environment.王等人。 [314] showed that NCCs’ activated oxidative stress in aquatic organisms (Scenedesmus obliquus algae, planktonic crustacean Daphnia magna and freshwater fish larva Danio rerio) at concentrations above 0.1 mg/L. The NCC form, morphology and concentration are believed to be the contributing factors, thus suggesting evaluation of nanocellulose ecotoxicological impacts when used at large scales. One method of reducing the undesired effect to the environment is by providing a mechanism to capture, retain or recycle the NPs during processing. Magnetic NPs (e.g. magnetite) [315] or surface modification can be used for this purpose. Almost all toxicity studies on nanoparticles recommend more research to be conducted on finding suitable analytical techniques and protocols in detecting organic NPs within biological matrices as well as mechanistic and environmental investigations to understand potential NPs risks on the ecosystem [301, 302, 310, 316].
Conclusions
The potential of nanotechnology in wood-based products sector is enormous. Nanotechnology offers the opportunity to change the landscape of wood-based products industry locally and globally. Forest itself via forest plantation can provide sustainable sources towards the creation of new generation of cellulose called nanocellulose that could offer myriads of application in various industrial fields. Besides, nanocellulose is less expensive than carbon-based material (e.g. carbon nanotube, graphene), environmentally friendly and may improve the recyclability and performance of countless products. For instance, nanocellulose can be used to improve the performance of paper and reduce the use of pulp in papermaking, thus resulting in cost saving. Nanocellulose can also be made into nanopaper or thin film for use in paper packaging or other sector such as electronics which offers alternative to non-renewable plastic. Nanocellulose used in wood composite can lead to the development of advanced composites which can be tailored to specific uses. Together with their strength properties and affordability makes them a viable solution to reduce the need for solid wood. Industrial sectors other than wood-based products could also benefit from the use of nanocellulose such as in energy device and sensors. Various nanocellulose-based products for energy storage and energy harvester applications as well as sensor have been developed at laboratory scale. In view of this interesting development, Malaysia has embarked on the production of nanocellulose from various tropical lignocellulosic materials. Unfortunately, the application of nanocellulose at commercial scale in Malaysia is still non-existent. Thus, it requires collective, concert and intensive efforts from various players that include stakeholders, industry and researchers to promote the use of nanocellulose and ensure that nanocellulose could enter the next level of application and commercialisation stage. The use of nanosized material other than nanocellulose has already benefited and also given advantage to wood-based products area which includes pulp and paper, wood composite, wood coating and wood preservative.
数据和材料的可用性
All data and materials are available without restriction.
缩写
- AgNPs:
-
银纳米粒子
- Al2O3:
-
Aluminium oxide
- AuNPs:
-
Gold nanparticles
- BTZ:
-
Benzotriazole
- C:
-
Carbon
- CCA:
-
Chromated copper arsenate
- Cd:
-
Cadmium
- CdS:
-
Cadmium sulphide
- CeO2:
-
Cerium oxide
- CH2CHSi(OC2H5)3:
-
Vinyltriethoxysilane
- CMC:
-
羧甲基纤维素
- CNT:
-
碳纳米管
- CO2:
-
Carbon dioxide
- CTAB:
-
十六烷基三甲基溴化铵
- CTAB-NCC:
-
Cetyltrimethylammonium bromide-nanocrystalline cellulose
- CWA:
-
Castor-oil-based waterborne acrylate (CWA)
- DFO:
-
Deferoxamine drug
- FESEM:
-
场发射扫描电子显微镜
- f -MWCNTs:
-
Functionalised multi-walled carbon nanotubes
- FTIR:
-
Fourier transform Infrared
- GOx:
-
葡萄糖氧化酶
- HALS:
-
Hindered amine light stabilizers
- HNE:
-
Human neutrophil elastase
- ISE:
-
Inorganic solid electrolyte
- LDH:
-
Layered double hydroxide
- LIB:
-
Lithium-ion battery
- LiTFSI:
-
Bis(trifluoromethanesulphonyl)imide lithium salt
- L-NCC:
-
Lignin-coated nanocrystalline cellulose (L-NCC)
- MC:
-
Moisture content
- Mg–Al LDH:
-
Magnesium–aluminium layered double hydroxide
- MPA:
-
3-Mercaptopropionic acid
- MUF:
-
Melamine-urea formaldehyde
- MWCNT:
-
Multi-walled carbon nanotube
- NCC:
-
Nanocrystalline cellulose
- NFC:
-
Nanofibrillated cellulose
- Ni:
-
镍
- NiO:
-
Nickel oxide
- NP:
-
纳米粒子
- 西北:
-
Nanowollastonite
- O:
-
氧气
- OH:
-
Hydroxyl
- OLEDs:
-
Organic light-emitting diodes
- PA:
-
Polyamide
- PCL:
-
Polycaprolactone
- PCP:
-
Pentachlorophenol
- PDMS:
-
聚二甲基硅氧烷
- PE:
-
Polyethylene
- PEG:
-
Poly(ethylene glycol)
- PEO:
-
聚环氧乙烷
- PHB:
-
Poly(3-hydroxybutyrate-co-4-hydroxybutyrate)
- PHRR:
-
Peak heat release rate
- PLA:
-
Polylactic acid
- PMC:
-
Perfluoroalkyl methacrylic copolymer (PMC)
- PMMA:
-
聚(甲基丙烯酸甲酯)
- PO4 3− :
-
Phosphates
- POTS:
-
Perfluoroalkyltriethoxysilane
- PP:
-
聚丙烯
- PPE:
-
Porcine pancreatic elastase
- PPG:
-
Propylene glycol
- 附注:
-
聚苯乙烯
- PSU:
-
Polysulphone
- PU:
-
聚氨酯
- PV:
-
Photovoltaic
- 量子点:
-
量子点
- RFID:
-
Radio-frequency identification
- S:
-
Sulphur
- SC:
-
Supercapacitor
- Si NMs:
-
Silicon nanomembranes
- SiO2:
-
Silicon oxide
- SPE:
-
Solid polymer electrolyte
- SPI:
-
Soy protein isolate
- SWCNH:
-
Single-walled carbon nanohorns
- TBOT:
-
Tetrabutyltitanate
- TEM:
-
透射电子显微镜
- TEMPO:
-
2,2,6,6-Tetramethylpiperidin-1-oxyl
- Ti(OC4H9)4:
-
Tetrabutyltitanate
- 二氧化钛:
-
二氧化钛
- TMI-BNT:
-
Transition metal ion-modified bentonite
- TNFC:
-
TEMPO-oxidised nanofibrillated cellulose
- Tyr:
-
Tyrosinase
- UF:
-
Urea formaldehyde
- UP:
-
Unsaturated polyester
- 紫外线:
-
紫外线
- VRFBs:
-
Vanadium redox flow batteries
- VTES:
-
Vinyltriethoxysilane
- WPU:
-
Waterborne polyurethane coating
- WUV:
-
Waterborne
- Zn-Al LDH:
-
Zinc-aluminium layered double hydroxide
- 氧化锌:
-
氧化锌
- ZnSt2:
-
Zinc stearate
- ZnSt2/WUV:
-
Zinc stearate/waterborne UV
纳米材料