纳米结构缓释局部麻醉剂的进展
摘要
缓释局部麻醉剂 (LA) 因其在改善镇痛和减少 LA 不良事件方面的有前景的作用而受到越来越多的关注。纳米结构的载体,如脂质体和聚合物囊泡,最能满足缓释/缓释的需求,几十年来一直用于药物递送,并显示出令人满意的缓释效果。基于成熟的脂质体技术,首个获批的载有布比卡因的 LA 脂质体 EXPAREL 以缓释形式获得成功。与此同时,聚合物囊泡比具有互补特征的脂质体取得了进步,这激发了混合载体的出现。本文总结了近年来纳米结构缓释LAs的研究成果,其中脂质体和聚合物是主流系统。此外,通过持续优化,药物输送系统具有超越简单运输的特性,例如特异性和响应性。在不久的将来,我们可能会实现靶向递送和控释特性,以满足各种镇痛需求。
介绍
自1996年以来,疼痛因其对身心健康的重要性而被视为“第五生命体征”[1]。为了应对传统疼痛管理中发生的阿片类药物危机,引入了多模式镇痛的概念。局部麻醉剂 (LA) 是多模式方案中最常用和最安全的镇痛剂之一 [2, 3]。然而,有限的持续时间(少于 24 小时)和潜在的毒性(心脏和中枢神经功能障碍)限制了其应用,并增加了平衡副作用和长期镇痛的紧迫性 [4,5,6]。尽管使用带泵的一次性导管来延长 LA 的持续时间,但在手术过程中导管移位、感染和创伤的风险确实存在。此外,导管放置既费力又费时[5]。缓释 LA 弥补了上述缺点。它们能够通过单次给药(通常在没有全身麻醉的情况下注射,需要微创技术和特殊工具)连续释放安全剂量,以确保最小的全身毒性。同时,可以延长痛觉阻滞的持续时间。
与宏观尺度的药物递送系统 (DDS) 相比,纳米尺度的 DDS 与纳米结构的生物环境更相容,这有利于其细胞渗透、更好的生物利用度和更长的保留时间 [4]。随着制造技术的进步,纳米级 DDS 在缓释方面取得了显着的成功,表现出更高的负载效率、更好的生物相容性(可接受的局部炎症,如肌肉毒性和神经毒性)和生物降解性(与负载化合物和确保完全磨损)[7,8,9]。此外,可调节和差异化的释放曲线(灵活的持续时间、调节的镇痛强度和通过特定修改的受控靶向释放)旨在满足不同的需求 [7, 8]。此外,目前可供选择的材料种类繁多,大大降低了成本,扩大了纳米级DDS的应用[7]。
然而,纳米级 DDS 仍面临着因高表面积体积比而突然释放、货架上脂质体稳定性差以及合成聚合物不合格的异物反应等缺点[4],所有这些都吸引了越来越多的努力。进一步优化纳米级 DDS。在这里,我们总结了纳米结构缓释 LA 的几种最先进的配方。最常用的形态是纳米颗粒,而纳米结构的凝胶、niosome、薄膜和棒也可以使用 [8, 10, 11]。在这里,提供并比较了合成技术、释放曲线、镇痛效果和安全质量,以帮助未来的设计和开发。
局部麻醉剂如何工作?
镇痛机制和理化性质
周围神经是疼痛传递过程中感知疼痛刺激的第一站 [12]。从一开始就考虑抑制疼痛,停止下游反应和更难以控制的神经可塑性的适应不良变化是合理的[13]。因此,LA 成为一个完美的选择。 LAs 通过与电压门控 Na 通道的细胞内结构域结合,抑制 Na + 的流入作用于周围神经 ,导致去极化阻滞[14](图1a,b)。
<图片>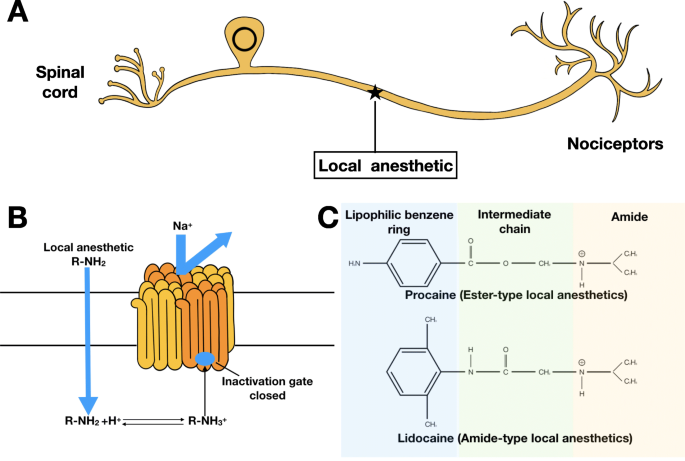
LA 的功能和结构特性。 一 , b 演示 LA 如何与神经元上的电压门控钠通道相互作用。 c 酯和酰胺LAs的典型结构
图>LAs 由三个化学基团构成:亲水性氨基(主要是叔胺)、亲脂性苯环和可以是酰胺或酯的接头,决定了 LAs 的分类(图 1c)。酰胺类 LA 是最常用的,包括布比卡因、罗哌卡因、利多卡因和甲哌卡因。酯类 LA 包括氯普鲁卡因、普鲁卡因和丁卡因 [14, 15]。药代动力学如起效速度、效力和持续时间在很大程度上取决于其理化特性。通过神经元膜的渗透性是发病的决定性因素,它受带电和不带电 LA 之间的平衡影响。随着 pKa 接近细胞外 pH,更多的不带电荷的 LA 形成并扩散到神经元中以发挥作用。 LA 的效力,也称为镇痛功效,是亲脂性的结果,可以通过分配系数进行量化。持续时间是蛋白质结合亲和力的代表,随着游离 LA 的代谢,它也会产生一个储库。总体而言,酯类 LA 因其生理上相似的 pKa 显示出相对较快的起效,但由于更容易水解和体内蛋白质亲和力较差,因此持续时间较短,而布比卡因因其长链而成为持续时间最长的 LA [14] , 16](表 1)。
图>全身毒性
决定作用的特性也与全身毒性有关。尽管存在血脑屏障 (BBB),但 LA 仍容易以适当的分子量、pKa 和良好的亲脂性进入中枢神经系统 (CNS)。具有低分子量、高脂溶性和适当 pKa 的 LA,如利多卡因和普鲁卡因,显示出脊髓-脑液浓度与血浆药物浓度的快速平行变化 [19]。由于LA-Nav通道结合是非排他性的,当LAs意外泄漏到心血管系统时,会攻击心脏和大脑等具有大量血液灌注和电压门控通道(Na、K、Ca)活性高的器官最好是由 LA 导致器官功能障碍 [20, 21]。除了抑制易损器官的兴奋传导外,线粒体功能障碍和细胞凋亡引起的能量消耗也有贡献[15, 22]。
因此,总体毒性是由细胞外药物浓度、细胞膜通透性和诱导的毒性反应引起的,这可以解释季衍生物 QX-314 与利多卡因相比更严重的 CNS 毒性,尽管 QX-314 穿透 BBB 和细胞膜较慢。 23]。与更常见但相对更容易控制的中枢神经表现(抽搐和癫痫发作)相比,心脏功能障碍,如传导障碍、心律失常和收缩功能障碍,可导致致命的结果 [6, 15]。为了延长LAs的镇痛作用,同时防止不良事件的发生,缓释LAs的开发已投入大量精力。
缓释 LA 的脂质体制剂
关于脂质体的一般概念
脂质体是一种以磷脂为基础的纳米级脂质囊泡[10]。脂质体技术长期以来一直用于药物递送以治疗癌症、感染和眼病等疾病。脂质具有通过酯键或醚键连接的亲水头和疏水尾[24]。脂质体是通过以双层形式聚集脂质单元而产生的,从而产生具有亲水性头部的水性鞘和核心,在层内屏蔽疏水性尾部。从简单的超声处理到更复杂的干法探测技术,基于超声处理、乳化、干喷和流动聚焦的不同组合开发了越来越多的创新方法,以生产具有改进特性的脂质体(图 2)[24,25, 26,27,28]。薄膜水化是最常用的生产技术之一,而微流体技术有望扩大规模[29]。药物可以通过不同的方式被截留,包括被动加载、主动加载、患者使用前加载和双乳(图 3)。最后,根据药物的亲水性,药物被携带在水核中或脂质层之间(图 4a)。这种慷慨的兼容性使脂质体能够胜任各种药物的输送[24]。
<图片>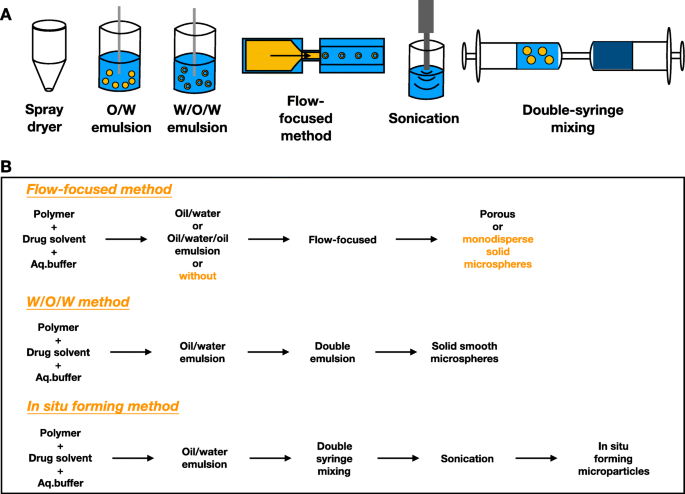
生产脂质体的经典技术。 一 脂质体的基本生产技术。 b 生产流程演示
图> <图片>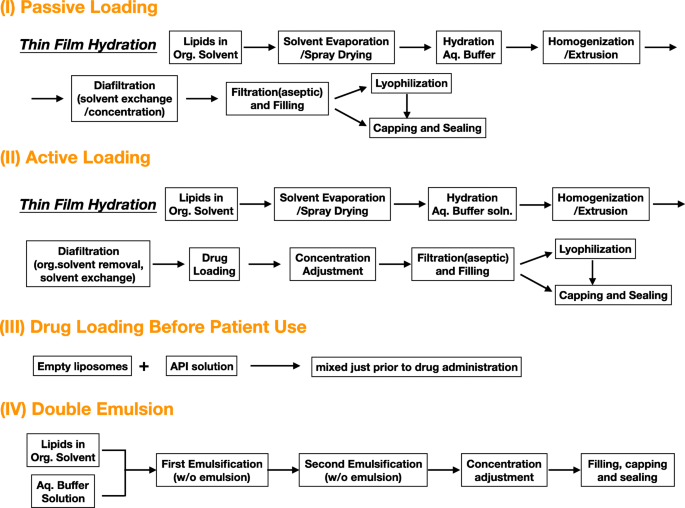
多种载药途径
图> <图片>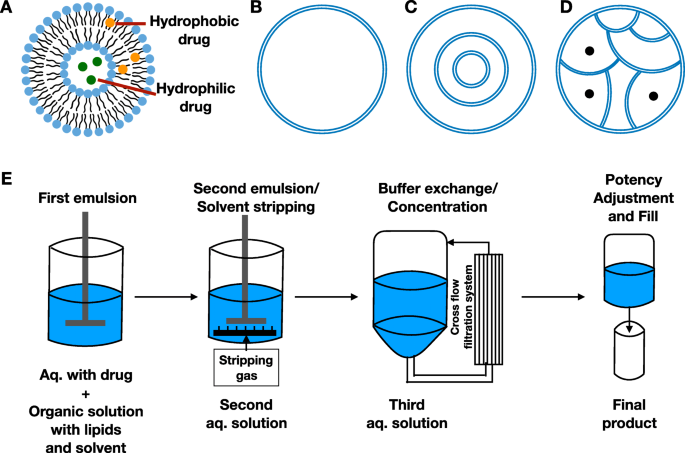
不同脂质体的结构特征。 一 脂质体中的药物分布。 b 单层脂质体。 c 多层脂质体。 d 多囊脂质体。 e DepoFoam 技术演示
图>在构建稳定有效的控释系统时,应考虑几个因素。脂质组成是设计脂质体的基本和关键因素。首先,应确保稳定的悬浮系统,这意味着不会发生聚集和融合。组合是第一个属性。表面电荷和静电力可以帮助脂质体相互排斥以避免聚集[30]。然而,悬浮环境可以中和表面电荷以诱导聚集。 1,2-Dipalmitoyl-sn-glycero-3-phosphate (DPPA) 和 1,2-distearoyl-sn-glycero-3-phospho-(1'-rac-glycerol) (DSPG) 是属于磷脂酰丝氨酸的两种带负电荷的脂质,当遇到钙和镁阳离子时往往会聚集 [31]。因此,含有较高比例带负电荷脂质的脂质体在添加二价阳离子后更容易聚集,这有助于确定脂质体悬浮液中的脂质比例和阳离子浓度。对于确定的成分,粒度是聚集的另一个属性。尽管有净电荷,但较大的颗粒更有可能聚集 [30]。除了离子成分,另一个环境因素是温度。低温会使脂质体靠得更近,从而导致聚集。建议储存温度略高于转变温度 [30, 32]。避免聚集的另一种策略是通过用聚乙二醇 (PEG) 包被来保护脂质体免于相互作用。这种亲水性分子可以作为外壳,不仅可以防止聚集,还可以拓宽表面改性的选择 [33]。在此想法的基础上,已经进行了改进以优化保护,例如添加胰岛素和葡聚糖以及聚乙二醇化双亲分子 [34, 35]。融合需要更多的吸引力,与聚集相比,这种吸引力不太常见,因为当静电力不存在时,水合排斥力存在[31]。而这种形式的排斥力可以通过增加磷脂酰胆碱的比例来增强[30, 31]。
在控释方面,降低不饱和脂质尾部的比例,增加长链脂质和醚接头可以促进脂质体的稳定性和缓释。插入胆固醇并用分子(如 PEG)进行修饰也可以稳定脂质体,如本综述后面所述,脂质体可归类为混合体。这些修饰降低了脂质体的流动性和生物降解性。表面电荷和粒度是另外两个考虑因素。中性电荷和较小尺寸的脂质体清除较慢 [24, 36]。辛醇/缓冲液分配系数是活性物质的特征,表明脂质体进入生理环境时药物的分布,这也决定了释放模式。辛醇/缓冲液分配系数高的布比卡因等药物容易释放[16, 36]。
组分不仅影响脂质体的理化特性,而且影响生理特性,例如组织特异性。当脂质体进入血液循环时,巨噬细胞的吞噬作用是主要的清除途径。然而,巨噬细胞在网状内皮系统 (RES) 中的不同识别由于不同的调理过程而发生,其中脂质成分、粒径和表面电荷是重要的决定因素 [37, 38]。例如,主要由胆固醇决定的补体系统的激活被肝脏汇管区周围的库普弗细胞识别,而饱和磷脂增强了脾脏和骨髓中巨噬细胞的识别[39]。较大直径的颗粒 (~ 100 nm) 优先被库普弗细胞清除 [37, 38]。此外,带负电荷的脂质体被库普弗细胞上的清道夫受体选择性识别,而中性脂质体更惰性 [38]。吞噬作用发生迅速,而细胞内过程缓慢,从数小时到数月不等,导致在 RES 中的保留时间延长 [37, 38]。这种保留可以帮助靶向 RES,同时阻碍系统性分配。已经制定了几种策略。细丝束的形态可以限制脂质体和受体之间结合位点的可及性。巨噬细胞不太喜欢软脂质体。通过聚乙二醇化和 CD47 修饰的隐形策略也可以钝化脂质体 [40]。额外脂质体使巨噬细胞饱和(RES 阻断)是延长载药脂质体循环的替代方法 [41]。氯膦酸盐可以更积极地清除巨噬细胞。
从巨噬细胞清除后,脂质体表现出良好的生物相容性和生物分布。对于血管多、血流灌注大、血管孔大的组织,脂质体有很大的进入倾向。肿瘤组织表现出上述所有特性。由于血管形成和淋巴引流异常,脂质体很容易进入并在肿瘤组织中停留更长时间,这被称为增强渗透性和滞留性 (EPR) [42, 43]。此外,具有更大尺寸和正电荷的脂质体可以克服肿瘤中心的高间质液压力并彻底穿透它[42]。除了血液运输的被动靶向和结构优势外,表面修饰的主动靶向也被广泛用于优化选择性,例如用于肿瘤靶向的表皮生长因子受体(EGFR)-配体和血管内皮生长因子(VEGF)-配体,以及转铁蛋白配体和鞘磷脂用于 BBB 渗透 [42, 44, 45]。
多泡脂质体
不同包装的脂质囊泡也有助于延长药物释放。通常,脂质体是同心的,其中脂质膜以多层或单层方式堆积(图 4b、c)[16]。因此,内部脂质膜的塌陷将导致药物蓄积并在外层破裂后迅速释放[46]。在这种情况下,无法实现持续释放。多泡脂质体是非同心的,其中囊泡从外部彼此紧密堆积(图 4d)。双乳液,也称为 DepoFoam 技术,用于生产多泡脂质体(图 4e)。关于多泡结构,应选择至少一种中性脂质(甘油三酯)和一种两亲脂质(磷脂)。甘油三酯的百分比在一定范围内与载药效率有关[46, 47]。它通过最外层囊泡的逐渐降解实现更长的释放。多泡结构在内部囊泡的重排过程中保持不变,内部囊泡被外部囊泡与外部环境隔离以避免突然释放 [46, 48]。多囊泡脂质体在释放持续时间方面表现出极大的优势,与单层和多层脂质体通过血管内途径释放药物相比,通过非血管给药可以在数天到数周内释放药物[46, 49]。
附加优势
与其他封装载体相比,脂质体在递送 LA 时可能具有额外的优势。众所周知,脂质乳剂疗法在治疗 LAs 全身毒性方面已显示出疗效,并已被纳入 LAs 毒性复苏指南[50]。潜在机制包括从心肌细胞的 Nav 通道中释放 LA,将 LA 分配和重新分配到储存(脂肪酸)、解毒(肝脏)和排泄器官(肾脏)、提供额外能量和增加 Ca 通道以增强心输出量 [51, 52]。尽管假设脂质体通过侵蚀和降解释放布比卡因,但同时发生通过脂质膜的扩散,这可以保持脂质体的完整性 [16, 53, 54]。有一项研究发现,即使药物在体外几乎完全释放后,大部分脂质体仍能保留其多囊泡结构,这表明扩散可能占主导地位 [46, 55]。此外,超声诱导的孔形成有助于保持脂质体的大小和结构不变[56]。因此,可以假设布比卡因在脂质体的保护下可能表现出降低的脂质体形式的毒性。需要进一步的研究和设计制造来确认和最大化这种好处。
由于良好的生物相容性和亲脂性,脂质体已被用于大脑定向药物递送 [57, 58]。有几种机制通过 BBB 介导渗透。受体或吸收介导的胞吞作用是穿越 BBB 的主要途径 [45]。吸收在很大程度上取决于脂质体和带负电荷的细胞膜之间的静电吸引力 [45]。当脂质体足够小时,例如包含亲脂性药物的单层脂质体,可能会发生被动扩散。还设计了修饰以加强配体-受体结合,以促进大脑导向的药物递送 [57]。因此,负净电荷和缺乏BBB特异性配体等逆向设计有助于防止大脑穿透并增强安全性。
EXPAREL-First 脂质体 LA 批准
EXPAREL (Pacira Pharmaceuticals, Parsippany, New Jersey, USA) 是唯一获得美国食品和药物管理局 (FDA) 批准的缓释脂质体 LA。脂质体悬浮液是通过 DepoFoam 技术与属于中性脂质的三辛酸和两亲性脂质,包括 1,2-dipalmitoyl-sn-glycero-3-phospho-rac-(1-glycerol)、1,2-dirucoylphosphatidylcholine 和胆固醇 [47, 49]。 2011年获批的伤口浸润疗法和2018年获批的肌间沟臂丛神经阻滞,在有限的临床情况下被合法使用,可达到长达72小时的缓释和镇痛效果[5]。
与常规镇痛方案,如游离 LA 注射、疼痛泵、硬膜外镇痛和患者自控镇痛 (PCA) 相比,EAPAREL 在通过伤口浸润使用时已证明其镇痛效力非劣效。除了标签上的适应症外,EXPAREL 在其他镇痛途径中也显示出有前景的作用,例如肋间神经阻滞、腹横肌平面 (TAP) 阻滞和卫星神经节阻滞(表 2)。然而,有研究显示 EXPAREL 在伤口浸润方面的镇痛效力是负面的。这些研究在不同疼痛强度的手术中有所不同,在疼痛评估的时间点上也有所不同,这些时间点相对较早,如12小时。骨科手术通常会导致患者剧烈疼痛,因此使用 EXPAREL 的单伤口浸润可能不足以控制疼痛。与普通布比卡因相比,EXPAREL 在初始时间可能不会释放足够的布比卡因,并且不能与游离 LA 共同给药以达到更好的镇痛效果(表 3)。因此,EXPAREL的镇痛效果可能取决于手术类型、疼痛强度和预期的镇痛作用。
图> 图>尽管在镇痛方面存在争议,但与导管插入、神经阻滞和硬膜外镇痛相比,EXPAREL 的给药途径更简单、更安全 [62, 75, 76]。在动物模型中,EXPAREL 在神经周围/神经内或蛛网膜下腔注射后不会引起神经毒性(神经元浓度降低或脱髓鞘)[77,78,79]。但与常规盐酸布比卡因相比,EXPAREL 诱导注射部位周围的局部炎症更高,但与生理盐水相似 [78]。总体而言,EXPAREL 显示出与游离布比卡因相似的毒性 [80,81,82,83]。与硬膜外镇痛 [63, 84] 和连续泵 [60] 相比,使用 EXPAREL 的医疗成本进一步降低,但成本高于普通 LAs 注射 [85,86,87,88]。因此,EXPAREL的使用需要综合考虑,包括不同手术对镇痛预期的不同要求和成本效益。
注意事项
由于脂质层的流动性和重排,EXPAREL 在应用上有明显的限制。由于布比卡因在脂质体中的替代,布比卡因在额外使用游离 LA 的情况下会快速释放,尤其是在 20 分钟内[89]。在这种情况下,局部麻醉全身毒性(LAST)的发生率将急剧增加。利多卡因尤其对 DepoFoam 表现出更强的亲和力,在 20 分钟内与 EXPAREL 共同给药时,会导致利多卡因和布比卡因的全身暴露量更大。然而,爆发释放的风险不仅是由于替换,而且是由于所使用的 LA 和血管收缩剂的血管效应以及稀释/混合效应。因此,超过 20 分钟的时间可确保 EXPAREL 和游离 LA 的安全水平 [90]。至于盐酸布比卡因,允许与 EXPAREL 的混合物比例低于 1:2,以确保安全和持续释放 [5, 49]。由于镇痛是多学科合作,因此应谨慎管理 EXPAREL。此外,所有脂质体产品都存在储存问题,被描述为“货架上”不稳定。脂质在长期储存后会降解为有害的代谢物。过多的溶血脂和其他脂质碎片会与红细胞结合,导致致命的溶血。用壳聚糖或海藻酸盐包被可以通过稳定脂质体并将保存期延长至2年来克服这个问题[24, 36]。
更多灵感
还从其他方面努力提高布比卡因脂质体的镇痛效果。克里斯托弗韦尔登等。通过将特定尺寸减小到十倍,增加细胞-脂质体布比卡因相互作用,延长其区域保留时间,与 Bier 阻滞中的常规脂质体布比卡因相比,实现了更长的麻醉和相似的安全质量 [91]。 Changyou Zhan 和他的同事结合金纳米棒和脂质体来实现光触发麻醉。这种光反应系统最大限度地减少了所需的单剂量近红外光,并表现出令人满意的安全性。更重要的是,它实现了按需和重复的区域麻醉,这将使疼痛管理更加个性化和精确[92]。或者,加强 LAs 与脂质的相互作用,有证据表明 LAs 与不同的脂质具有不同的亲和力 [93],并用海藻酸盐包裹脂质体以增强间充质基质细胞的抗炎反应 [94],可能进一步改善控释并满足不同的临床需求。
缓释 LA 的聚合物配方
高分子DDS基础知识
聚合物是由数千个重复单元(单体)组成的大分子。与范德华力和氢键起重要作用的脂质体不同,聚合物由共价键形成,不仅在货架上提供更好的稳定性,而且在与游离 LA 共同给药时也提供更好的稳定性。大量生物相容性和可生物降解的聚合物已用于制造缓释材料,不仅是天然的,而且是合成的。考虑到酰胺和酯 LAs 的不同化学性质,可以使用相应的加载方法来优化加载效率(静电相互作用、共价共轭和封装)[8]。此外,当需要注射、敷料或薄膜时,纳米颗粒、纳米胶囊、纳米凝胶、纳米薄膜和纳米纤维等灵活的形态扩展了聚合物 DDS 在实践中的应用 [7, 8]。此外,结构修改使聚合物 DDS 的释放曲线可调节,例如围手术期疼痛的天数、慢性疼痛的数周以及与第二种药物共同给药以提高 LA 的疗效 [7]。
其中最常用的合成聚合物是聚酯。该类别包括聚(l-丙交酯)、聚(乙醇酸)、聚(乳酸-共-乙醇酸)和聚(ε-己内酯)[95]。代谢物通常是二氧化碳和水等小分子,可以安全回收或排泄[96]。另一方面,生产技术已经从传统的双/单乳液、沉淀和喷雾干燥等技术发展为微流体平台、挤出和非润湿模板(PRINT)中的颗粒复制(图5a-g)。还开发了静电纺丝等新技术,以实现配方和交付的灵活性(图 5h)[95, 96]。
<图片>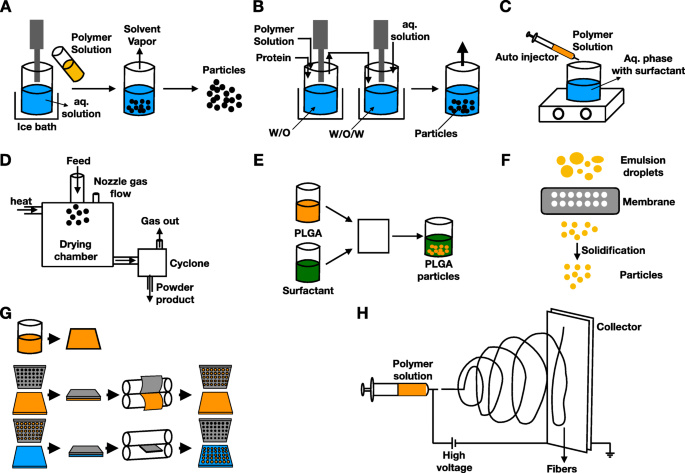
聚合物囊泡的经典技术。 一 单一乳液。 b 双乳液。 c 纳米沉淀。 d 喷雾干燥。 e 微流体。 f 挤出乳化。 g 非润湿模板中的粒子复制 (PRINT)。 h 静电纺丝
图>还有另一种生产方法,自组装,尚未广泛用于药物输送,但可能会启发 DDS 的未来发展方向。自组装与其他生产程序不同,它在水性环境中自发组装成两亲聚合物-试剂共聚物的胶束。因此,它表现出简单、高效和良好的水分散性 [97, 98]。共聚物可以通过多种反应形成,如一锅多组分反应(仲酰胺与甲醛连接的活性氢化合物之间的甘露醇反应)[97, 99]、超分子反应(主客体相互作用,如环糊精和金刚烷) [100, 101]、酯化和开环反应 [102,103,104]、硫醇-炔点击反应 [105] 和共聚物的后改性 [106]。尽管该技术最近主要用于荧光聚合物粒子以帮助防止荧光猝灭并实现聚集诱导发射,但不同的反应和相互作用使得不同的疏水性药物可以找到响应性聚合物(尤其是有机聚合物)以自组装成胶束 [107, 108]。例如,酰胺 LA 中的仲酰胺可用作通过甘露醇反应与聚合物反应的活性部分。或者,药物可以被吸收到共聚物中以实现同步成像和治疗 [101]。由于疏水性药物被包裹在胶束的核心中,它可以为药物功效提供合适的内部环境,例如LAs的碱性环境。同时,良好的细胞摄取行为可能会增强靶向细胞内结构域(如 LA)的药物 [97, 100, 105]。此外,反应通常无催化剂/简单(如微波和超声)[109, 110],溶剂经济,实验条件温和,省时,这使其在未来能够大规模生产[97, 105] ].
聚合 DDS 通过多种途径释放活性剂。当水开始流入聚合物时,对流会产生渗透力将药物泵出。然而,通过在水性环境中形成的孔进行扩散是主要方式。它有助于亲水性药物(例如离子化 LA)泄漏。相反,疏水性药物可以直接扩散通过。 At the final stage of a typical tri-phase release profile, erosion of polymer dominates [111, 112].
Like liposomes, polymersomes also face the challenge of RES clearance. Morphology modification, stealth strategy, RES blockade, and macrophage depletion can be applied as well [40, 113,114,115]. Furthermore, the EPR effect facilitate tumor-target while auxiliary surface modification can help tissue specificity [43, 116]. Polymersomes do not induce significant systemic inflammation while some of natural polymers such as hyaluronan and laminin, and synthetic polymers such as negatively charged poly(lactic-co-glycolic acid) and polyurethane can reduce systemic inflammatory reaction [117,118,119]. Moreover, N -(2-hydroxypropyl) methacrylamide and polystyrene are reported to reduce neurotoxicity [120, 121].
Diverse Morphology and Applications
Particle is a common form of polymeric DDS. Polymeric particles are generally divided into two categories:nanosphere and nanocapsule. Nanosphere is an evenly disperse system, while nanocapsule is an embedding drug in polymeric cavity [95]. The earliest researches built nanoparticles with poor entrapment efficiency (< 60%) and release duration (< 30 h) [122, 123]. With finesses of technology, the drug entrapment efficiency reaches nearly 90% [124,125,126], the highest result is 93.3% [127]. In-vitro release can reach a maximal duration of 35 days [128]. There is another available form termed nanofiber, which is produced by electrospinning. Electrospinning technique has been widely applied in drug delivery, but limited usage in LAs. There is one research only using electrospinning technique to fabricate bupivacaine-loaded suture. It gained satisfactory results on extended release (over 12 days), while significant analgesia appeared from day 1 to day 9 in rat skin wound model [129]. Mostly in polymersomes, drugs are entrapped physically; in polymer-drug conjugate, drug is bonded to polymer covalently to enhance drug loading capacity and prolong release furthermore [130]. Apart from solid forms, amorphous forms such as gel show more versatility and malleability. A 60-day release was seen in the work of Daryl Sivakumaran and his colleagues (Table 4) [134]. Rapid burst release over 60% of LAs was observed in the work of Haibo Qu and his colleagues (Table 4) [135].
图>In order to make the release intelligent, responsive materials are used in situations where structural changes, volume-phase transitions, or sol-gel transitions are needed [137]. Inflammatory reaction is a major and initial process when trauma happens. Along with it is the changes of pH and temperature. Thus, pH and temperature are stimuli used commonly in LAs delivery. In the work of Todd Hoare and his colleagues, thermal aggregation can help control regional accumulation of thermal-responsive nanogel. However, larger precipitates after aggregation induced more severe local inflammation (Table 5) [139]. Teresa Alejo and his colleagues used sequential heat pulse to trigger the collapse of nanoparticle, fastening profound release in a spatiotemporal way (Table 5) [138]. A pH-responsive polymer (methacrylicacid–ethyl acrylate) was used by Jeremy P.K. Tan to achieve decreased release of procaine chloride with decrease of pH (Table 5) [140].
图>Despite the advantages of being responsive to stimuli, pH and temperature-responsive polymers do not act precisely enough to localize therapeutic agents. Moreover, acidic environment and high temperature may bring harm. Therefore, stimuli which are able to modulate therapeutic agents precisely without harm are wanted. Although there is no application so far in LAs delivery, ultrasound-responsive nanoparticles exhibiting harmless property have shown precise localization in tumor targeting and imaging [141, 142]. However, ultrasound signal can be influenced by air attenuation and bone structure. Magnetic responsive nanoparticles exhibit broader application such as in respiratory system which is filled with air and central nervous system covered with cranial bone [143]. Furthermore, magnetic responsive nanoparticles can help spatially and temporally localize actives without restriction by tissues depth [143]. Generally, there are three mechanisms underlying magnetic response, which are magnetic deformation, magnetic guidance, and magnetic-induced hyperthermia [144, 145]. Aginate-based ferrogel undergoes pore formation with magnetic stimulation [146]. In contrast, chitosan-based nanoparticle undergoes pore formation with the help of heat produced by magnetic field [147, 148]. In order to decrease thermal damage to surrounding tissues, Wei Chen and his colleagues produced a core-shell structure to embed heat-producing core and prevent thermal damage [143]. The guidance function can help the nanoparticle migrate long distance and penetrate biological barrier [149]. Combination of aforementioned mechanisms can create synergistic outcomes [150].
Polymeric carriers endow LAs extended-release, but unexpected burst release does exist. Long-term degradation may induce foreign body response. Versatility of materials and mature techniques are advantages of polymeric carriers. Acidic metabolites, however, may hamper the effect of LAs. Although responsive polymers make delivery more intelligent, they do not solve the aforementioned problems above completely. Therefore, further improvements are needed.
Lipid-Polymer Hybrid Carriers of Local Anesthetic
Liposomal and polymeric nanocarriers both have drawbacks such as low solubility, poor stability, undesired drug leakage. and diffusion [151,152,153]. Conventional liposomes are easily cleared out by enzymatic degradation and macrophage engulfing. Therefore, surface modification with inert and hydrophilic coats, such as PEG, ganglioside GM1, and phosphatidylinositol, have been used for protection against degradation by enzymes and macrophages. Furthermore, pegylation can enhance the negative charge of liposome and reinforce its attraction to cationic actives. In the work of Brett A. Howell and his colleagues, higher concentration and slower release of bupivacaine in human serum was observed in pegylated liposomes compared to conventional liposomes [154]. Alternatively, apolar cavity of biologically active compounds formed by cyclodextrins (a polymeric peptide), also known as an inclusion, in multi-vesicular liposomes can help achieve longer release. Inspired by various pioneer attempts to overcome drawbacks of liposome and polymeric nanoparticles, lipid-polymer hybrid nanoparticles come to the stage of controlled release [151, 155].
There are three systems of hybrid nanoparticles:lipid-core polymer-shell, polymer-core lipid-shell, and the polymer-lipid matrix. Lipid-core polymer-shell system using a polymeric coat improves the stability of liposome and delays clearance, while maintaining optimal biocompatibility of lipid core. The amphiphilic properties of some polymers can reduce surface tension of nanoparticles and decrease particle size to achieve better biocompatibility. Additionally, responsive polymers can enable liposomes more flexible behavior in various physical environment [152, 156]. Poly (acrylic acid) (Chol-PAA) is a pH-sensitive polymer, which tends to form globular structure from random coil in low pH. In poly (acrylic acid) (Chol-PAA)-caged liposome, the polymeric coat shrinks when environmental pH decreases, compressing core liposome to release significantly when liposome collapses [152]. Marina Sokolsky-Papkov and her colleagues used hybrid polymer (DL-lactic acid and castor oil) to formulate bupivacaine-loaded nanoparticles. This poly (fatty-ester) achieved relatively longer in-vitro release (beyond 1 week) and sensory blockade (> 72 h) compared to previous research [157]. Further research confirmed its release profile and extended its analgesic efficacy among thermal pain, mechanical pain, and rearing assessment [158]. Another research study explored the difference between oil nucleus on stability, release profile, and analgesia property, and found that a higher entrapment efficacy was observed compared to polymeric DDS [159].
Polymer-core lipid-shell system is commonly used in intravenous administration of drugs, especially anticancer drugs [151, 155]. The two components together help inhibit water infiltration and slow hydrolysis down, and as a result prevent drug diffusion and burst leakage. Lipid shell also enhances biocompatibility of nanoparticle with similarity to cell membrane [160]; this is promising in achieving simultaneous co-delivery of multiple drugs [151, 152, 160]. On the other hand, polymeric core can facilitate mechanical stability, shape control, and size distribution. Additionally, it can increase drug entrapment efficacy. In the work of Jianguo Wang and his colleagues, nanoparticle showed a slower release speed, smaller particle diameter, and higher drug loading compared to traditional liposomes [161]. The work by Pengju Ma at el. showed that hybrid bupivacaine nanoparticle had better stability, analgesic efficacy, and cytotoxicity [162].
The third system is a polymer-lipid matrix which can be subdivided into polymerized liposomes and nano-in-micro type. As their names suggest, polymerized liposome is a covalently bound liposome which demonstrates better stability and modulated release as mentioned above with basic knowledge on polymeric DDS [154], while the nano-in-micro system gathers nanoparticles into a matrix to achieve controlled release as shown in the work of Khanal Manakamana and his colleagues [163]. Moreover, hybrid nanoparticle was used in transdermal route showing better skin permeation than free bupivacaine in three studies by Yaocun Yue and Aimei Li at el., which expands its clinical application of analgesia [161, 164, 165].
Future Perspectives
Nano-structured systems have been utilized in extended-release for drug delivery over decades. Tremendous breakthroughs have been seen in controlled release of LAs to achieve longer duration of analgesic and better safety profile, bringing in the successful approval of EXPAREL. At the same time, various modifications and combinations of nano-structured systems have broaden the horizon of LAs. With the guidance of physical or chemical stimuli, targeting LAs to treat the specific sources of pain may not be a difficult process anymore. Additionally, with the help of external and internal stimulus, we may modulate the release profile of LAs even after administration. Physiochemical properties of actives, rather than carriers alone, are also taken into account to formulate new and flexible systems. In the future, we may see precisely designed extended-release LAs to meet different demands of analgesic intensity, duration, and target sites in distinct surgeries, to make analgesia more individualized.
结论
LAs are key components in multimodal analgesia. Short duration and adverse side effects limit its application, which induces the emergence of extended-release LAs. Nano-structured DDSs show better biocompatibility and biodegradation compared to micro-structured DDSs due to similar size with physiological environment. Among various nanocarriers, liposomes achieve the first success in super-long-lasting LAs, which can release bupivacaine for 72 h in vivo. Liposomes also potentiate the safety of LAs with the protection of emulsion. The instability of liposome, however, hinders its storage and co-administration profile with additional free LAs. Compared to liposome, polymersome has a more advantageous profile with better stability and prolonged release. Moreover, the electrospinning technique and the stimuli-responsive property endow polymersomes with more flexibility in morphology and release behavior. Besides the optimization of materials and manufacturing processes, combination of nanocarriers is an alternative way to improve drawbacks and boost strengths. This is where hybrid nanocarriers come to the stage:hybrids not only improve the release profile but also broaden administration routes, such as the transdermal route. With the ever-emerging versatility of nanocarriers, extended-release may become more specific and controllable in the future to satisfy various analgesic demands.
数据和材料的可用性
不适用。
缩写
- BBB:
-
Blood-brain barrier
- CNS:
-
Central nervous system
- DDSs:
-
Drug delivery systems
- DPPA:
-
1,2-Dipalmitoyl-sn-glycero-3-phosphate
- DSPG:
-
1,2-Distearoyl-sn-glycero-3-phospho-(1'-rac-glycerol)
- EGFR:
-
Epidermal growth factor receptor
- EPR:
-
增强渗透性和滞留性
- FDA:
-
American Food and Drug Administration
- LAs:
-
Local anesthetics
- LAST:
-
Local anesthesia systemic toxicity
- PCA:
-
Patient controlled analgesia
- PEG:
-
Polyethylene glycol
- PRINT:
-
Particle replication in nonwetting template
- RES:
-
Reticuloendothelial system
- TAP:
-
Transversus abdominis plane
- VEGF:
-
Vascular endothelial growth factor
纳米材料