用于合成和生物医学应用的荧光纳米材料的进展和挑战
摘要
随着纳米技术的飞速发展,近二十年来新型荧光纳米材料(FNMs)层出不穷。纳米尺度赋予 FNM 独特的光学特性,这在其在生物成像和荧光相关检测中的应用中起着至关重要的作用。然而,由于荧光纳米材料的低选择性和低光致发光效率在一定程度上阻碍了它们在成像和检测中的应用,科学家们仍在寻找合成具有更好性能的新型 FNM。在这篇综述中,总结了多种荧光纳米粒子,包括半导体量子点、碳点、碳纳米粒子、碳纳米管、石墨烯基纳米材料、贵金属纳米粒子、二氧化硅纳米粒子、磷光体和有机框架。我们重点介绍了近年来 FNM 合成及其在生物医学领域应用的最新进展。此外,还回顾和讨论了 FNM 合成和应用的主要理论、方法和局限性。此外,还系统地总结了合成和生物医学应用中的挑战。还介绍了FNMs在临床应用中的未来方向和前景。
介绍
传统的有机染料由于其固有的缺陷,如细胞毒性和生物相容性差,在生物医学中的应用一直面临一些困难[1]。然而,荧光纳米材料的出现在替代传统有机染料方面显示出巨大的潜力。科学家们在荧光纳米材料的研究上投入了大量的时间和精力,相关的合成和应用成果令人鼓舞。
荧光纳米材料的形状、尺寸和结构决定了它们的物理和化学性质,这对其性能有着巨大的影响。因此,荧光纳米材料的可控合成成为研究的热点。合成的最佳实验条件有助于荧光纳米材料的最合适的尺寸、形态和稳定性。近年来,人们通过改进合成方法来提高荧光纳米材料的生物相容性[2]。过去,金属离子通常掺杂碳点 (CD) 或量子点 (QD) 以对荧光纳米材料的表面进行功能化。然而,无效的荧光和潜在的毒性对其在生物成像和生物标记中的应用构成了威胁 [3]。考虑到这些问题,左等人。报道了一种高效的 CDs 基因传递系统。掺杂氟的 CDs 是通过溶剂热法合成的,用于基因传递的正电荷位点可以由支化聚乙烯亚胺 (b-PEI) [4] 提供。可以预见,新的表面改性方法将成为未来的研究热点。
已经做出了许多努力来探索荧光纳米材料在生物医学应用中的潜力,包括生物成像、生物检测和一些治疗方法,如图 1 所示。可靠的荧光应用取决于它们的物理和化学特性 [5]。因此,提高其毒性、亲水性和生物相容性等性能的研究工作已成为实现荧光纳米材料在生物医学领域广泛应用的重要组成部分。随着癌症等一些疾病的发病率不断增加,患者对具有更高准确性和依从性的新型诊断和治疗策略的需求不断增加[6]。目前,金属或非金属离子掺杂和荧光纳米材料的表面改性仍然是提高荧光纳米材料的PL效率和生物相容性的主导技术[7],相应的研究为荧光纳米材料的生物医学应用开辟了新的视野。
<图片>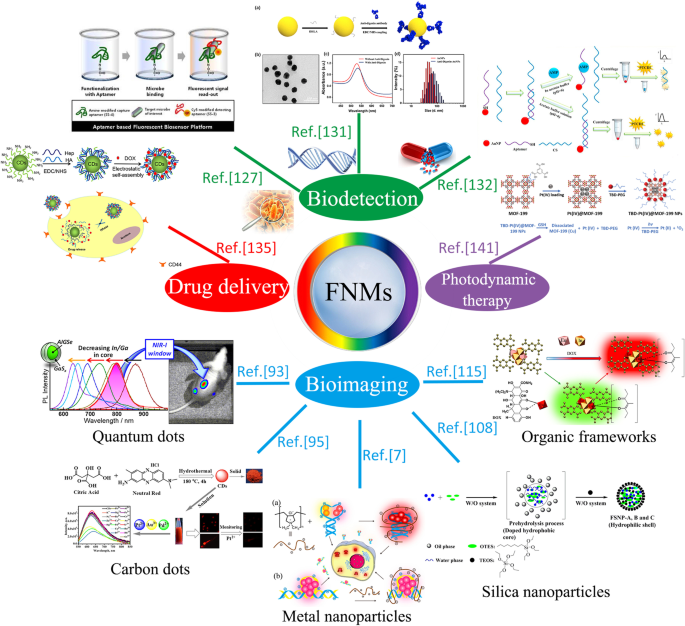
荧光纳米材料生物医学应用概述
图>考虑到荧光纳米材料在生物医学领域的巨大潜力,本综述重点介绍了最新进展和改进。科学家们致力于荧光纳米材料表面的功能化及其在生物医学应用中的性能。只有设计合理的合成策略,荧光材料才能具有高PL效率和良好的生物相容性,这对其在生物医学领域的应用至关重要。本文对荧光材料的合成与应用的综述,希望能对读者了解当前荧光纳米材料的总体发展趋势有所帮助。
荧光纳米材料的合成
量子点(半导体晶体)
量子点(QDs)由于其广泛的吸收和对称的光致发光光谱、高量子产率、高抗光漂白性、高摩尔消光系数和大的有效斯托克斯位移而成为过去几十年的研究热点[8]。在量子点的形成机制方面,当电荷载流子(电子和空穴)被势垒限制在某些区域时,半导体表现出剧烈的量子尺寸效应,导致吸收光谱和荧光光谱发生偏移。小区域小于电荷载流子的德布罗意波长,或者等效地,纳米晶体直径小于主体材料中激子玻尔半径的两倍[9]。当电荷载流子被三个空间维度的势垒限制时,就会形成 QD,主要由 II-VI(CdSe、ZnS)、III-V(GaAs、InP)或 IV-VI(PbS, PbSe).
1982 年首次报道了 QD 的合成 [10, 11]。半导体的纳米晶体和微晶体生长在玻璃基质中。随着荧光材料的发展,量子点的制备方法多种多样,如直接吸附法、接头辅助吸附法、原位法以及与以往制备方法相结合的方法。现有方法的组合包括制备的半导体与QD前驱体的组合和先前制备的QD与半导体前驱体的组合,其中分别制备半导体或QD[12]。
在对量子点的合成进行了一系列的研究后,大量的研究人员报道了量子点的荧光性质研究。巴文迪等人。通过引入半导体前体如硫化镉 (CdS)、硒化镉 (CdSe) 或碲化镉 (CdTe) 合成了具有窄尺寸分布的 QD,以研究 QD 的尺寸相关光学特性 [13]。此后,CdSe 成为量子点最常见的化学成分,并利用各种表面改性 [14,15,16] 或保护性无机壳 [13, 17] 来提供胶体稳定性。
碳点
碳点 (CD) 是纳米碳家族中的新兴纳米材料,其尺寸小于 10 nm,于 2004 年首次在通过电泳纯化单壁碳纳米管 (SWCNT) 中获得 [18]。值得注意的是,CDs以其在水中的高溶解度、低细胞毒性、高光稳定性、激发依赖的多色发射、更好的表面修饰灵活性、优异的细胞渗透性和更好的生物相容性等优点逐渐取代了半导体量子点[19, 20]。通常,CDs主要包括碳量子点(CQDs)和石墨烯量子点(GQDs)。大量尺寸可调的CDs的合成方法大致可以分为两大类:化学方法和物理方法[21]。
化学合成方法
化学合成方法是制备碳点最常用的方法,因为所得 CDs 具有优异的性能,例如优异的水溶性、化学惰性、低毒性、易于功能化和抗光漂白。一般来说,化学合成方法包括电化学合成[22、23]、酸性氧化[24、25]、水热碳化[26]、微波辅助/超声波处理[27、28、29]、溶液化学法[30]、支持合成[31]等
在众多的合成方法中,电化学合成在过去几十年中被反复报道过。赵的团队报道了一种通过电氧化合成制备低细胞毒性 CDs 的新方法,其中 CDs 是通过在 NaH2PO4 水溶液中用 Pt 线对电极对饱和甘汞电极氧化石墨柱电极来制备的 [22]。然后将上清液通过离心过滤装置进行超滤,分别获得具有蓝色和黄色荧光的 CD。 Qu 等人最近报道了另一种直接的电化学方法,通过在磷酸盐缓冲溶液中电化学氧化石墨烯电极,获得 3-5 nm 均匀尺寸的 GQD [23]。这些颗粒的光致发光(PL)颜色为绿色。
毛等人。 2007年完成了CDs的燃烧氧化合成,将烛烟灰与氧化剂混合,然后回流、离心和透析净化CDs。制备的 CDs 光致发光光谱具有广泛的颜色范围,发射峰值波长范围从 415(紫色)到 615 nm(橙红色)。然后,将获得的 CD 进一步进行聚丙烯酰胺凝胶电泳,以分离具有不同光学特性的 CD。酸性氧化也被广泛用于制备稳定的纳米材料,如碳点。碳纳米管/石墨酸处理并回流后,所得3-4nm的CD呈棕褐色透明液体,在紫外光下发出亮黄色荧光,在盐水中非常稳定。这使得具有长波(黄色/橙色/红色)荧光的 CD 具有更好的穿透性。 CD溶液可在室温下长期保存,不会产生导致荧光损失的沉淀物[25]。
微波/超声合成在合成过程中逐渐成为主要的辅助合成技术[32]。直径为 3-5 nm 的荧光 CD 由 Xiao 的团队通过一种经济、快速和绿色的微波辅助方法合成 [33]。这种一步法最显着的特点是,CDs 的形成和功能化是通过首次从离子液体中提取的微波热解同时完成的 [34]。反应过程发生在微波炉中,使用廉价的离子液体作为碳源,随着反应时间的进行,溶液从无色变为深棕色 [35]。唐等人。以葡萄糖或活性炭为碳源,采用超声波法合成单分散水溶性CDs。它们发出明亮多彩的荧光 [28]。同样,瓦内萨·罗梅罗等人。在蔬菜中的碳水化合物光化学氧化后获得高荧光氮(N)和硫(S)共掺杂碳点(CD)。 N和S的共掺杂增加了CDs表面活性位点的数量,从而增强了其发光性能[36]。掺氮碳量子点 (NCQD),一种荧光探针,已成功应用于多西环素的测定 [37]。帕塔克等人。还制备了与氮和硫共掺杂的碳点(NSCDs),其由硫脲和三乙酸乙二胺缓冲液通过微波水热法合成。由于多色荧光法,NSCDs被用于对各种病原菌和人口腔上皮细胞进行成像[38]。
考虑到上述合成方法大多需要强酸,实验步骤繁琐,并需要与其他化合物进一步修饰,以提高CDs的水溶性,增强其光致发光性能,一些研究团队利用碳水化合物光致发光的水热碳化,如壳聚糖、葡萄糖、柠檬酸等,以避免复杂且耗时的纯化和功能化过程 [39]。杨等人。描述了一种通过壳聚糖在温和温度下水热碳化制备高度氨基功能化荧光 CD 的一步合成方法,量子产率 (QY) 为 7.8%。该方法既不需要强酸溶剂,也不需要表面钝化剂。此外,CDs 表面的官能团提高了它们的水溶性并降低了它们的潜在生物毒性[26]。多掺杂碳点 (MCD) 具有明亮且颜色可调的发射,通过一锅法合成,无需任何进一步的表面钝化。合成的 MCDs 掺杂了丰富的生物元素(O、N、P),因此显示出强烈的荧光发射和激发波长依赖性、良好的水溶性、高光学稳定性以及离子稳定性。 MCDs不仅可以选择性灵敏地检测Fe 3+ 在 15.9 nm 蓝光检测下,还可测量细胞内 Fe 3+ 通过多色荧光成像[40].
对于溶液化学方法,在过去的几十年中,芳基的氧化缩合已成功应用于 GQD 的制备。 Li 的团队采用增溶策略制备了具有所需尺寸和结构的稳定胶体 GQD。这种方法实现了 CD 的尺寸可调性和窄尺寸分布,而没有任何不切实际的尺寸分离过程 [30]。在支持的合成过程中,一些研究团队已经利用它完成了纳米CDs等单分散纳米材料的合成。朱课题组采用介孔二氧化硅(MS)球作为纳米反应器,柠檬酸作为碳前驱体,通过浸渍法制备了尺寸为 1.5-2.5 nm 的亲水性 CD。具有 23% 高光致发光效率的 CD 能够发出强烈的蓝色发光并呈现出优异的转换发光特性 [31]。 Yan 等人 制备了亮黄色发光碳点 (Y-CD)。通过溶剂热法,以无水柠檬酸为碳源,2,3-吩嗪二胺为氮源。具有丰富羧基的 Y-CD 显示出可观的荧光量子产率 (24%)、188 nm 斯托克斯位移、高灵敏度和出色的稳定性 [41]。 CDs的合成方法和性能如表1所示。
图>物理合成方法
一般来说,物理合成方法主要有电弧放电、激光烧蚀/钝化、等离子处理等。 Xu 和同事用 HNO3 氧化电弧放电烟灰,然后通过凝胶电泳将悬浮液分离成 SWCNT。他们最终分离出了高荧光碳点纳米粒子的快速移动带 [18]。 Li等人以纳米碳材料为前驱体,以环境友好型溶剂为液体介质制备了CDs。通过温和的激光烧蚀方法 [44]。此外,Gokus 及其同事证明了使用氧等离子体可以将强荧光诱导到单层石墨烯中 [45]。
碳纳米粒子
荧光碳纳米粒子具有降低的细胞毒性、抗光漂白性和增加的生物相容性,在生物成像和其他生物医学应用中越来越受到关注。与典型的碳点尺寸在 1-6 nm 之间相比,碳纳米颗粒的尺寸超过 20 nm,省去了分离、纯化和收集的麻烦[46]。碳纳米粒子的合成方法与碳点类似,包括水热碳化、微波处理、化学烧蚀法和激光烧蚀法。这些方法各有优势,但不能有效控制纳米粒子的尺寸。电化学碳化是一种单步法,可以控制碳纳米粒子的尺寸和发光性能。不幸的是,这种方法可用的基板很少。目前已报道了一些新的有趣的方法,如五氧化二磷燃烧法[47]。
近年来,适用于生物医学应用的碳纳米粒子是通过改进的方法合成的。桑图等人。通过间苯二酚的受控碳化解决了高质量红色荧光碳纳米粒子的合成 [48]。这种方法涉及与脱水相关的氧化苯酚偶联,以形成红色荧光碳纳米颗粒。阿纳拉等人。使用改进的水热法合成了量子产率为 6.08% 的荧光碳纳米粒子。与需要长达数小时的长时间热处理的传统方法相比,该方法将反应时间缩短至不到30分钟,实现了荧光碳纳米粒子的快速合成[46]。
碳纳米管
一维 (1D) 碳纳米管凭借其优异的电子和光学特性在生物医学领域引起了极大的关注。碳纳米管根据圆柱形石墨烯层数可分为单壁碳纳米管(SWCNTs)和多壁碳纳米管(MWCNTs)。 SWCNT 由单层石墨烯片卷成圆柱体组成,而 MWCNTs 由几层同心石墨烯片组成。碳纳米管的外径在 100 nm 以下,但它们的长度可以达到几毫米,这导致非常高的纵横比和大表面积 [49]。此外,碳纳米管中碳原子的独特排列在纳米管外形成了丰富的 π 电子共轭 [50]。此外,碳纳米管在近红外区具有很强的吸收和荧光[51]。所有这些特性都有助于与生物分子的有效相互作用,这使得碳纳米管成为生物医学应用的理想候选者。
合成方法对碳纳米管的直径、长度、结构、手性和质量有很大影响,同时需要考虑该方法是否适合大规模生产。常用的方法包括电弧放电 [52]、激光烧蚀 [53] 和化学气相沉积 [54]。最重要的是,需要对碳纳米管进行功能化以提高它们的溶解度并防止它们在溶剂和生物介质中聚集。共价功能化会给碳纳米管的结构带来缺陷,导致它们的 NIR 荧光显着降低甚至完全丧失。用聚合物等两亲分子进行非共价官能化将保留碳纳米管的结构和荧光特性,但会降低碳纳米管的 QY。为了克服这些障碍,最近报道了合成和功能化碳纳米管的新方法。李等人。据报道,作为还原剂的二硫苏糖醇的加入首次可以增强 SWCNT 的荧光 QY,从而产生具有与 QD 相当的亮度的荧光团 [55]。侯等人。研究了将二硫苏糖醇添加到用各种表面活性剂功能化的 SWCNT 中。对于DNA和SDS包裹的SWCNTs,它们的荧光QY显着增加,而其他表面活性剂观察到不同程度的荧光猝灭[56]。因此,在DNA或SDS包裹的SWCNTs中加入二硫苏糖醇是实现碳纳米管在生物医学中应用的可行方案。
石墨烯纳米材料
作为二维碳纳米材料,石墨烯及其衍生物已被广泛探索用于一系列生物医学应用,如生物成像和药物输送。石墨烯纳米材料包括石墨烯纳米片、氧化石墨烯(GO)和还原氧化石墨烯(rGO)纳米片。它们具有高表面积和独特的表面特性,允许与染料分子、生物分子和水不溶性药物进行非共价相互作用。自2004年首次制备成功以来,许多研究人员报道了不同的石墨烯制备方法。石墨烯纳米材料的合成方法可分为自顶向下和自底向上两大类。
自上而下的方法涉及从堆叠的石墨层中分离以形成石墨烯片,包括机械剥离 [57]、基于溶剂的剥离 [58] 和电化学剥离 [59]。顾等人。系统研究了超声辅助溶剂型去角质,发现超声波具有良好的去角质效果。它们还可以影响石墨烯片的尺寸和厚度分布,这使得可控合成成为可能。自下而上的方法涉及使用替代碳源重组碳原子。外延生长 [60] 和化学气相沉积 (CVD) [61] 是最常用的自下而上的合成方法。 GO表由许多sp 2 组成 可以使用 Hummer 方法合成由含氧基团分离的结构域。这些 sp 2 的大小变化 域使 GO 片的 PL 范围很广,从 500 到 800 nm [62]。 rGO 是通过使用还原剂(例如氢醌和肼)进行化学还原而衍生自 GO。与GO相比,rGO的荧光在紫外区呈现蓝移发射和荧光猝灭,这归因于新形成的晶体sp 2 之间的渗流途径。 集群 [63]。阿克巴里等人。阐明了 sp 3 的比例 /sp 2 GO 表中的域决定了它们的荧光光谱。因此,GO是一种在不同还原程度下在宽波长范围内具有前景的荧光纳米材料,可用于生物医学应用。
金属纳米材料
同时,与量子点相比,贵金属原子的细胞毒性更小。金、银、铜纳米粒子受到越来越多的关注,并被应用于众多领域。在生物医学领域,金纳米粒子的量子力学效应,如光致发光发射或等离子体共振使金纳米粒子(AuNPs)成为另一种低细胞毒性的体内纳米传感器的理想候选材料[64, 65]。
AuNPs由于其易于合成和独特的性质而引起了广泛的科学兴趣,并且已经报道了多种合成方法。作为最重要的方法之一,化学方法通常通过在稳定剂的存在下用还原剂处理氯金酸盐水溶液来进行。柠檬酸应用最广泛,既可以作为稳定剂,也可以作为还原剂[66]。然而,在使用硫醇盐配体的功能化开发过程中,用柠檬酸稳定的 AuNPs 会经历不可逆的积累。这个问题可以通过在水溶性聚合物、表面活性剂或封端剂的存在下进行反应来克服,这些聚合物有助于提供更高的稳定性并防止纳米颗粒聚集。 AuNPs的大小和形状可以通过改变金-柠檬酸盐比例、表面改性剂或反应条件来控制。通过一锅超声乳化法,张和他的同事将双(4-(N-(2-萘基)苯基氨基)苯基)-富马腈和AuNPs共同加载到胶束中以获得纳米探针[67]。最重要的是,所获得的纳米探针在体内肿瘤靶向成像和诊断方面具有巨大的潜力,尽管存在金纳米粒子,但仍具有出色的荧光成像能力。虽然AuNPs在一定的实验条件下是无毒的,但其毒性和副作用需要深入研究[68]。
荧光银纳米团簇因其独特的物理和化学性质而备受关注。这种纳米簇的合成过程通过稳定支架分为 DNA 寡核苷酸、肽、蛋白质、树枝状聚合物和聚合物。此外,大量文献已经证明了一些绿色合成,例如 D 的含水茎提取物的应用。三叶草和S. alba 优化制备条件[69]。
铜纳米团簇(Cu NCs)作为贵金属材料应用相对广泛,但由于易氧化,其合成仍然很少。最近,川崎等人。通过微波辅助多元醇方法成功制备了稳定的 Cu NCs [70]。 DNA 可用作合成荧光 Cu NCs 的模板。莫希尔等人。提出了一种基于溶液中双链 DNA 的方法来获得高选择性的 Cu NCs [71]。利用Cu NCs的荧光特性,成功地将其作为有效的荧光开启信号指示剂用于RDX的选择性测定[72]。
二氧化硅纳米粒子
考虑到嵌入荧光团的透明度、机械稳定性、鲁棒性和稳定性,二氧化硅纳米粒子广泛应用于生物领域。例如,二氧化硅核/壳纳米颗粒应用于细胞内检测 Zn 2+ 和 H2PO4 − 在活细胞中合成为“开/关”荧光纳米传感器。近年来,掺杂有机染料的二氧化硅纳米粒子已被合成并广泛用于许多应用,例如生物检测 [73]。二氧化硅纳米粒子最广泛使用的合成方法是 Stöber 法和反微乳液法。 Stöber 方法首先在 1960 年代 [74] 中描述,它涉及硅酸烷基酯的水解和随后通过添加氨催化的醇溶液中的硅酸缩合。二氧化硅 NP 形成的第二种方法,即反向微乳液法,涉及硅酸烷基酯(通常为 TEOS)在油包水微乳液的水滴内反应 [75]。他等人。用Stöber法和反微乳液法将染料嵌入颗粒核中制备了三种染料掺杂二氧化硅纳米颗粒。一些分子对 Zn 2+ 有功能响应 沉积在颗粒表面 [76]。荧光二氧化硅纳米粒子用于细胞内Zn 2+ 的荧光成像 (H2PO4 − ) 在 HeLa 细胞中。当 Zn 2+ 添加到比例 Zn 2+ 纳米传感器,纳米颗粒显示出能够以比例检测H2PO4 - 的浓度 .
一般来说,具有良好单分散性和生物相容性的二氧化硅纳米复合材料可以很容易地用官能团进一步改性 [77,78,79]。李等人。将磁性纳米粒子和荧光染料掺杂到二氧化硅纳米粒子中。这些二氧化硅纳米复合材料不仅可以用作磁共振 (MR) 和荧光成像的多模态成像探针,还可以用作抗癌药物递送载体 [80]。简而言之,二氧化硅颗粒可以成为此类情况下的研究热点,用途广泛。
磷光体
磷光体因其在减少组织自发荧光和光散射干扰方面的独特优势而被广泛应用于生物医学。通常,磷光体由主体材料和掺杂离子组成 [81]。在磷光体的主体材料中,氧化钇 (Y2O3) 由于其低光耐久性和声子能量而极具前景。考虑到镧系元素丰富的电子能级和能量转移通道,镧系元素主要掺杂在磷光体中。已经报道了许多制备荧光粉的方法,包括水热法[82]、火焰喷雾热解法[83]、溶胶-凝胶法[84]和共沉淀法[85]。
水热合成正在成为一种理想的工艺,已被证明是合成荧光粉的有效且经济的方法。余等人。合成 Y2O3:Eu 3+ 在柠檬酸钠存在下通过水热法制备荧光粉 [82]。柠檬酸钠的浓度、水热过程中NaOH和Eu的加入量决定了所得荧光粉的性质。火焰喷雾热解是一种快速连续合成氧化物基荧光粉的有前途的方法。与传统方法相比,该方法提供了具有高结晶度和均匀掺杂分布的磷光体。汗等人。成功生产Tb 3+ – 掺杂 Y2O3 荧光粉,其直径约为 100 nm,使用火焰喷雾热解法制备的粒径分布较窄 [83]。在他们的方法中,碱金属盐与其他金属硝酸盐前体混合,有效地控制了窄范围内的尺寸分布。溶胶-凝胶合成路线提供了几个优点,例如高均匀性和纯度、缩短合成时间、均匀的颗粒形态和窄的粒度分布 [86]。莱昂纳多等人。获得 Sm 3+ 通过溶胶-凝胶工艺掺杂 SiO2-Gd2O3 荧光粉 [84]。共沉淀是一种常见且简单的合成晶体磷光体的方法,可确保高均匀性和可控的形态特征。佩海塔等人。据报道,磷光体的相组成强烈依赖于沉淀过程中的pH值[85]。
Organic Frameworks
Covalent-organic frameworks (COFs) are new porous crystalline materials possessing outstanding stability, adsorption and low toxicity. The design of fluorescent small organic molecules with a combination of fluorescence determination methods can be used to construct more efficient nanoprobes [87]. For selective 2,4,6-trinitrophenol (TNP) determination, a novel Naphthalimide-Benzothiazole conjugate was prepared as colorimetric and fluorescent nanoprobe. The fluorescence emission peaks of receptor were selectively quenched by TNP with a limit of detection as low as 1.613 × 10 –10 M.
Metal organic frameworks (MOFs) are a kind of new generation multifunctional inorganic–organic materials with various holes and functionalized 3D crystalline structures formed by metal ions and linkers. MOFs show potential applications in separation, catalysis and other aspects due to unique attributes such as excellent chemical tenability, specific surface area and confinement of the pores. Some of the MOFs are luminescent and the quantum yield as well as light intensity will be influenced by temperature and excitation wavelength [88]. With the addition of doxycycline, Yu et al. synthesized a new functional metal–organic framework of pyromellitic acid and europium, which exhibited remarkable fluorescence enhancement at 526 nm and 617 nm. Results showed that both fluorescence intensities were positively correlated with the doxycycline concentration. The unique fluorescence response of the system could discriminate doxycycline from other tetracycline antibiotics with high selectivity.
Biomedical Applications of Fluorescent Nanomaterials
Bioimaging
Quantum Dots for Bioimaging
Fluorescent nanomaterials have been widely used in bioimaging. Compared with conventional organic fluorescent molecules, fluorescent nanomaterials are equipped with many superior properties such as high photostability, tunable emission spectra and high quantum yields [89].
As early as 1998, QDs were first successfully applied in biological imaging [90]. Since then, applications of QDs in this field have been springing up gradually. Chen’s group applied it in bioimaging and nuclear targeting with great stability and biocompatibility in living cells [91]. In spite of the extremely high sensitivity and spatial resolution of QDs, poor performances on hydrophilicity and biocompatibility hindered their applications in bioimaging in vivo. To tackle this problem, it has been found that the water-solubility of QDs can be greatly improved by attaching thiol or other hydrophilic groups to the surface of quantum dots [92]. In the same way, with the intention of improving the effectiveness and specificity of in vivo targeted imaging, targeting molecules are attached to the surface of QDs. Furthermore, the wavelength region of the emission light can be controlled by altering the size of QDs.
The combination of QDs and inorganic metal ions can optimize the application of QDs in bioimaging because the QDs’ defect-site PL peaks will be utterly removed by controlling the proportion of doped inorganic metal ions. Kuwabata, S et al. modulated the degree of Ga 3+ doping in Ag–In–Se QDs. Thus, the QDs’ defect-site PL peaks were completely removed and a sharp band-edge emission peak come into appearance [93]. They found a blue shift of the band-edge PL peak ranging from 890 to 630 nm which could be credited to the fact that the energy gap of QDs was enlarged by Ga 3+ doping. After injecting a mouse with QDs, the potential of AIGSe@GaSx core–shell QDs for bioimaging turned out satisfying. The imaging effect of this kind of QDs in mice is demonstrated in Fig. 2. However, sensing of mid-IR wavelengths is challenging due to increased dark currents and noise. HgTe QDs synthesized by colloidal method is a promising candidate for IR bioimaging by virtue of lower dark currents, higher-temperature operation, and higher detectivity [94].
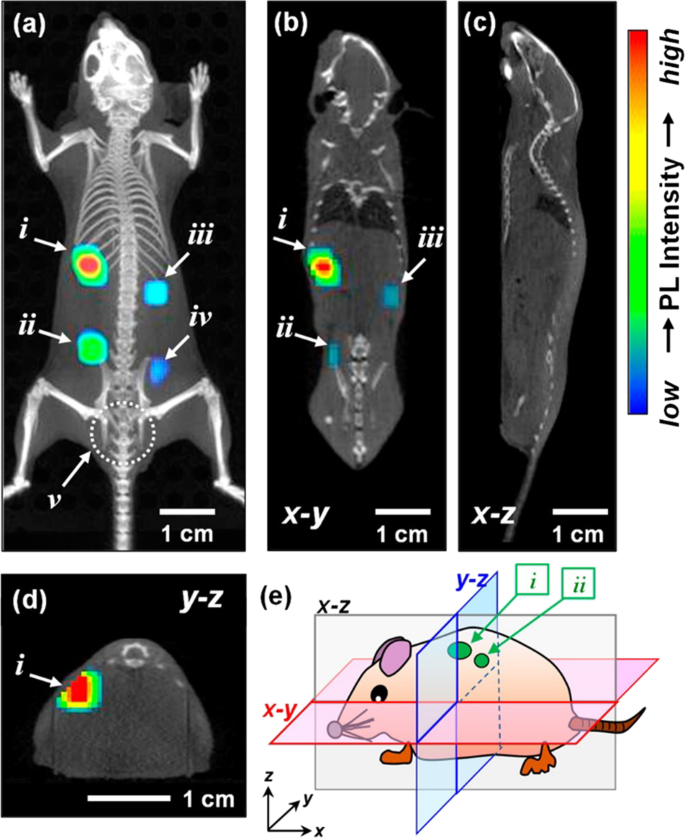
Three-dimensional PL image superimposed on an X-ray CT image of the mouse subcutaneously injected with DSPC-AIGSe@GaSx liposome dispersions (each 50 mm 3 ) in the back [78]
图>Carbon Dots for Bioimaging
The poor photostability of current fluorescent nanomaterials hinders their long-term bioimaging to a large extent. To overcome this limitation, CDs have been studied for bioimaging and some positive results have been obtained due to the great performance on PL efficiency. Enormous efforts have been put to improve their water solubility and lower their toxicity in organisms. At present, most CDs are facing a barrier in bioimaging, that is, their short-wavelength excitation disables deep penetration in tissue. Aside from this, being exposed under the short-wavelength for a long time could do irreversible damages to living cells and tissues. As shown in Fig. 3, with the purpose of overcoming this deficiency, Gao et al. designed fluorescent CDs with red emission which were successfully used for bioimaging of noble metal ions (Pt 2+ , Au 3+ , Pd 2+ ) in cells and zebrafish [95]. Sun and co-workers first studied the near infrared (NIR) imaging of CDs in vivo using mice as a model. Recently, it was reported that molecules or polymers containing plentiful sulfoxide or carbonyl groups can enhance NIR fluorescence through the surface modification. As shown in Fig. 4, under NIR excitation, sulfoxide or carbonyl groups are bound to the outer layers and the edges of the CDs. Thus, electron transitions are promoted, influencing the optical bandgap [96].
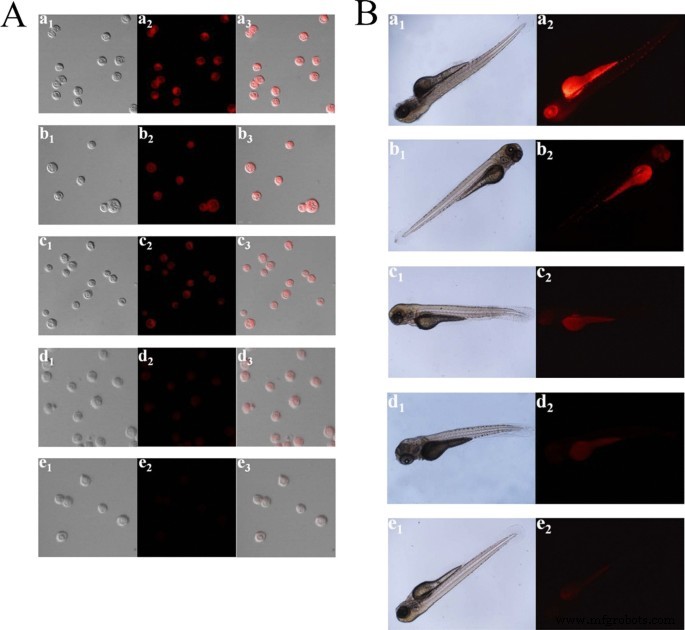
A Confocal imaging of Pt 2+ in PC12 cells. (a1–e1) Bright field images. (a2–e2) Black field images of the CDs in PC12 cells with the different concentrations of Pt 2+ (0, 25, 50, 150, and 300 μM). (a3–e3) Overlay images. B Fluorescence imaging of Pt 2+ in ZF. (a1–e1) Bright field images. (a2–e2) Fluorescence images of the CDs in ZF with the various concentrations of Pt 2+ (0, 30, 60, 100, and 150 μM) [80]
图>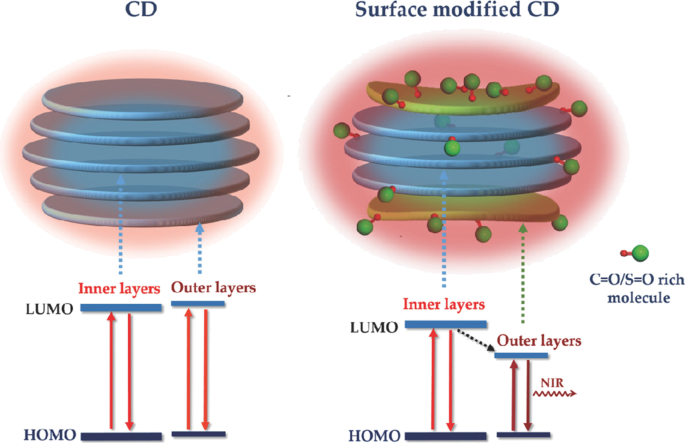
Schematic of structure and energy level alignments of nontreated CDs (left column) and CDs modified with S = O/C = O‐rich molecules (right column). The red (oxygen atom) and green double‐bonded balls represent the C = O/S = O‐rich molecule [81]
图>Carbon Nanoparticles for Bioimaging
In the field of bioimaging, fluorescent carbon nanoparticles show unique chemical and optical properties over traditional fluorescence probes. Different size, shape and elemental composition make carbon nanoparticles with different features. The biomedical fields are always seeking the most promising fluorescent carbon nanoparticles. Gaurav et al. obtained both larger and smaller size carbon nanoparticles with laser ablation method [97]. Both green and blue fluorescence were observed in the cells incubated with the carbon nanoparticles, suggesting their different sizes. Cell viability results indicated that the prepared carbon nanoparticles were nontoxic and safe for bioimaging applications. Shazid et al. employed carbonization method to obtain fluorescenct carbon nanoparticles derived from biocompatible hyaluronic acid. Both the in vitro and in vivo bioimaging studies showed that the prepared carbon nanoparticles would be reliable and stable for opticle imaging. Moreover, based on the experimenal data, their cytotoxicity was proved to be tolerable for biomedical applications.
Carbon Nanotubes for Bioimaging
Fluorescence of carbon nanotubes in the NIR is attracting high attention for their good light penetration depth in biological tissues. However, their low quantum yield requires for considerable excitation doses, leading to a fair degree of blue-shift and failure of penetrating live tissue. Mandal et al. reported that bright and biocompatible p-nitroaryl functionalized SWCNTs, encapsulated in phospholipid-polyethylene glycol, are suitable for bioimaging applications. The prepared SWCNTs enabled high signal-to-noise ratio imaging in live brain tissues using ultra-low excitation intensities. Their 1160 nm emissions in the NIR guarantee that they will provide optimal fluorescence imaging results [98]. Ceppi et al. applied SWCNT-based fluorescence imaging to debulking surgery in an ovarian cancer mouse model. SWCNTs are coupled to an M13 bacteriophage carrying modified peptide binding to the SPARC protein, which is overexpressed in ovarian cancer, leading to real-time imaging to guide intraoperative tumor debulking. This imaging system enables detection in the NIR window with a pixel-limited resolution of 200 μm, demonstrating real potential in fluorescence imaging guided surguries for patients [99].
Furthermore, fluorescent moieties can be conjugated by a carbon nanotube backbone, which integrate strong fluorescent ability with robust mechanism strength, exhibiting ideal bioimgaing results. Katharina et al. functionalized SWCNTs with an amphiphilic C18-alkylated polymer conjugated with bright perylene bisimide fluorophores. The polymers wrapping around the SWCNT backbones not only increase their water dispersibility but also promote their biocompatibility by providing a shield. In vitro studies on HeLa cells demonstrated that the biocompatibility of SWCNTs is dramatically improved. In microscopy studies, direct imaging of the SWCNTs' cellular uptake via perylene bisimide and SWCNT emission proved their potential for bioimging [100]. Park et al. combined carbon nanotubes with mussel adhesive proteins which can be specifically targeted at tumors in tissue. They then made carbon nanotubes conjugated with a ZW800 NIR fluorophore to obtain NIR fluorescence imaging [101]. The prepared carbon nanotube probes react with a specific tumor in one hour and can be easily eliminated via urine, demonstrating great value as tumor imaging and detecting agent.
Graphene-Based Nanomaterials for Bioimaging
Large surface area and feasible further functionalization make graphene-based nanomaterials a promising candidate for biomedical applications. However, as a result of their chemical sturctures, graphene nanosheets lack photoluminescence and rGO only display weak fluorescence, which makes it difficult to be utilized in bioimgaing applications. Many researchers attempted to resolve this problem by conjugation of fluorescent dyes and probes onto the large surface of graphene and its derivatives. Sun et al. reported an assembly strategy to prepare fluorescence probe RACD functionalized a single layer GO via π-π interaction and hydrogen bonding. The resluting nanomaterials exhibited that the fluorescent probes reduce the aggregation degree and acquire very well monodispersion, hydrophilicity and photostability, which is attributed to the strong synergy between RACD and GO [102]. Even so, fluorescence quenching remains a critical issue for these materials. In addition, the biocompatibility and toxicity of polymers applied to connect graphene-based materials and fluorescent moieties have not been adequately investigated. These facts appeal for alternative solutions to utilize graphene and its derivatives in bioimaging applications. Georgia et al. developed intrinsically photoluminescent graphene derivatives that show desirable biocompatibility and tunable fluorescence properties [103]. They can be organophilic or hydrophilic with different amine functionalization dodecylamine and hexamethylenediamine, respectively. The intrinsic fluorescent graphene-based nanomaterials possess great potential in a variety fileds of bioimgaing.
Metal Nanomaterials for Bioimaging
In recent years, fluorescent metal nanoparticles have shown great potential in bioimaging for improved disease diagnosis and treatment [104]. Gold is the most commonly used metal for bioimaging. The surface of AuNPs can be easily modified with various biomolecules such as peptides, proteins, antibodies, enzymes, and nucleic acids. These biomolecules can interact with specific cells or organelles in vivo, which makes it possible for AuNPs to be used for targeted optical imaging.高等人。 reported a real-time in situ imaging of nucleus by AuNPs fabricated with bifunctional peptides constructed with both Au-binding affinity and nucleus-targeting ability. The bifunctional peptides showed strong binding affinity toward AuNPs and ensured good surface coverage of the nanoparticles, which made it stable and efficient for precise bioimaging of the nucleus in cells [105]. The Au-Se bond is considered as a better candidate than the Au–S bond to link the peptides and AuNPs due to the stronger ability against interference of intracellular thiol. Pan et al. prepared the Au-Se-peptide nanoprobes through a direct freezing process. The obtained nanoprobe was successfully applied to identify autophagy and apoptosis in chemotherapeutic drug treated cancer cells [106].
As a novel fluorescent imaging technology, DNA-templated silver nanoclusters (DNA-Ag NCs) have aroused the attention of many scientists due to their unique properties, especially the tunable fluorescence emission range relying on DNA sequences. However, the highly negatively charged DNA backbones have always been a great obstacle for the expansive applications in bioimaging because of poor stability as well as poor cell permeability in physiological environment. It is also noteworthy that the PL property and fluorescent efficiency of DNA-Ag NCs are far from satisfying. As a result, figuring out how to neutralize the negative charge on the surface of DNA strands is of great urgency for researchers. Recently, Lyu and co-workers successfully modified fluorescent DNA-Ag NCs with cationic polyelectrolytes via electrostatic force between the positively charged polyelectrolytes and the negatively charged phosphate groups of the DNA strands, leading to a threefold fluorescence intensity enhancement [7] (Fig. 5).李等人。 reported a facile strategy to make gold nanoclusters with positive charge and silver nanoclusters with negative charge form aggregates by electrostatic interactions. An incredible 40-fold fluorescence intensity enhancement was obtained. Results demonstrated that the physiological stability improved a lot and the cell permeability was also enhanced, which promises its practical applications in the future.
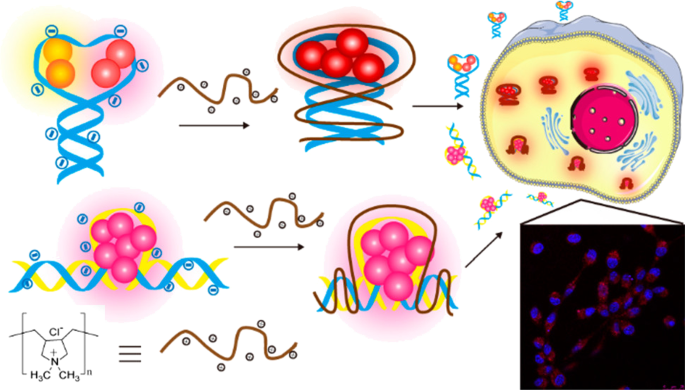
Formation of FL DNA–Ag NC–Cationic Polyelectrolyte Complexes for Cell Imaging [7]
图>Silica Nanoparticles for Bioimaging
Dye-doped fluorescent silica nanoparticles emerge with great potential for bioimaging as a novel and ideal platform for the monitoring of living cells and the whole body. The outer silica shell matrix protects fluorophores from outside chemical reaction factors as well as provides a hydrophilic shell for the inside insoluble nanoparticles, which renders the enhanced photo-stability and biocompatibility to the organic fluorescent dyes. Benefiting from the robust structure of silica matrices, dye-doped fluorescent silica nanoparticles have been presented with several superior properties including good biocompatibility, hydrophilic features, and high fluorescence intensity [107].
Jiao et al. also constructed a local hydrophobic cage in dye-doped fluorescent silica nanoparticles to improve their optical properties, which solves the problems of aggregation-caused quenching (ACQ) and poor photostability in aqueous media by organic fluorescence dyes benefiting from the robust structure of silica nanoparticles [108]. In addition, compared with free dyes, the fluorescent intensity both in water solution and living cells demonstrated a 12.3-fold enhancement due to the limitation of molecular motion, indicating a significant development for silica nanoparticles in biomedical applications. QDs have been developed for bioimaging both in vivo and vitro owing to their excellent optical qualities. However, a critical obstacle faced in QDs’ application in vivo is their poor biocompatibility. Inspired by the organic dye-conjugated silica-NPs, QDs-embedded silica-NPs have also been invented with the advantage that the excellent optical qualities of QDs can be retained, while the silica-NPs coat improves their biocompatibility to a large extent simultaneously. Darwish et al. reported that many QDs could be assembled around a central silica nanoparticle to form supra-NP assemblies. It was expected to be used for enhanced bioimaging because of their higher sensitivity and superior signal-to-background ratios [109]. There is reason to believe that silica-NPs conjugated with fluorescent nanomaterials with ideal optical properties will still be the dominant research interest in the future.
Phosphors for Bioimaging
For bioimaging, the sizes of phosphors need to be controlled so that they are small enough to be integrated with living cells. Furthermore, the aggregation of particles should be avoided for biocompatibility. Hence, the control of both particle sizes and dispersity in an aqueous solution is essential for the bioimaging application of the phosphors. Atabaev et al. prepared Eu, Gd-codoped Y2O3 phosphors which had a spherical morphology within the range 61–69 nm. Enhanced PL emission and low toxicity made these phosphors suitable for bioimgaing applications [110].
Upconversion nanomaterials are able to convert lower-energy near-infrared photons to higher-energy ones as emission. This anti-Stokes photoluminescence process will lead to low background noise, large tissue penetration depth, and low photo-damage in bioimaging applications [111]. Lanthanide-based phosphors are able to show upconversion emission owing to their photodurability and low phonon energy. Nallusamy et al. reported a NIR–NIR bioimaging system based on Er 3+ :Y2O3 phosphors by using NIR emission at 1550 nm under 980 nm excitation, which can allow a deeper penetration depth into biological tissues than ultraviolet or visible light excitation [112]. In addition, the surface of Er 3+ :Y2O3 was electrostatically PEGylated to improve the chemical durability and dispersion stability under physiological conditions. Thakur et al. synthesized Ho 3+ /Yb 3+ co-doped GdVO4 phosphors via a modified sol–gel method. The prepared phosphors showed brilliant red upconversion emission under NIR excitation, which may be useful in bioimaging of the biomolecules [113].
Organic Frameworks for Bioimaging
Careful selection of MOF constituents can yield crystals of ultrahigh porosity and high thermal and chemical stability, with some of them being luminescent [114]. Recently, Sava Gallis’s group described a novel multifunctional MOF material platform which showed a wide spectral region from 614 to 1350 nm covering the deep red to NIR region. Both porosity and tunable emission properties made them highly suitable for in vivo bioimaging [115]. What’s more, to overcome the obstacle of MOF’s low selectivity towards malignant tissues, Liu et al. developed a target-induced bioimaging by conjugating DNA aptamers using ZrMOF nanoparticles as quenchers [116]. Based on the quenching of ZrMOF nanoparticles, target-induced bioimaging is achieved upon binding with the target.
Biodetection
Since fluorescent nanomaterials can amplify the fluorescent signals significantly and be compatible with organisms, there is increasingly more research on their application in the rapid detection of biomolecules [117]. It will shorten the analysis time to a large extent if we are able to establish a real-time detection system by fluorescent nanomaterials. It has been discovered that multiple detection can be achieved by using QDs probes simultaneously [118, 119].
Pathogen Detection
Pathogens have been an unignorable threat to human health for centuries and these include many types of microorganisms ranging from bacteria (pathogenic Escherichia coli, Salmonella, and Streptococcus pneumoniae ) and viruses (Coronavirus, Influenza virus and hepatitis virus ). However, conventional methods for pathogen detection still need improvement of detection limits and detection speeds. For their applications in detecting pathogens, Tan and co-workers reported a bioconjugated nanoparticle-based biodetection for in situ pathogen quantification, which cost less than 20 min [120]. Tan’s success promises that quick and convenient pathogen detection is possible and can be achieved with these ingenious nanomaterials in the future. Here, we list diverse pathogens detected by fluorescent nanomaterials as shown in Table 2 [119, 121,122,123,124,125,126,127,128,129].
图>Nucleic Acid Detection
Apart from pathogen detection, fluorescent nanomaterials have also aroused more and more interest of scientists in the detection of DNA. Owing to the merits that a number of biomolecules can be attached to the surface of fluorescent nanomaterials, the signal intensity of fluorescent nanomaterials in DNA detection can be enhanced significantly. Tan and co-workers developed an DNA detection method to detect gene products using bioconjugated dye-doped fluorescent silica nanoparticle with high sensitivity and photostability [130]. Although the analysis of nucleic acid has been successfully achieved by real-time nanomaterial fluorescence systems, there are still many shortcomings such as complex procedures or expensive instruments. To address these disadvantages, Wang’s group introduced a highly sensitive and visualized detection of nucleic acid by the combination of strand exchange amplification (SEA) and lateral flow assay strip (LFA) [131]. The system, which is possible to be widely used in areas requiring limited resource, is mainly characterized by integrating SEA with LFA (Fig. 6). There is no denying that the extremely high fluorescent signal for bioanalysis plays an irreplaceable role in these applications.
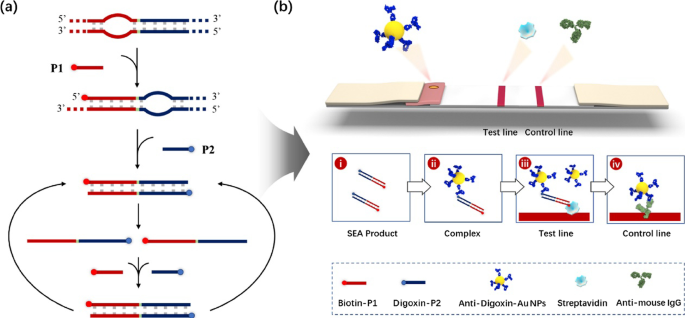
Schematic illustration of the SEA-LFA strip for the detection of nucleic acids [110]
图>Drug Detection
In the field of drug analysis, a facile and low-cost analytical method is always in demand for high-speed detection of specific pharmaceutical compounds. Real-time detection of drugs can be achieved with selective and sensitive fluorescent nanomaterials owing to their outstanding optical properties. In the past decade, the modification of nanomaterials has lowered their detection limit and improved their detection accuracy significantly. Recently, it is reported that ampicillin can be detected in serum sample based on aptamer, its complementary strand (CS) and gold nanoparticles (AuNPs) [132]. The limit of detection (LOD) of this method can be as low as 29.2 pM. However, there are still many limitations in the detection of drugs or target molecules in vivo. Due to low selectivity, conventional fluorescent nanomaterials inevitably generate false positive results and adverse effects in vivo. In addition, current tracking systems can hardly realize real-time tracking because of insufficient labels and excitation sources. Considering the above limitations, a new method using up/down conversion (UC/DC) PL nanomaterials has attracted increasing attention. Seo et al. reported a single-photon-driven UC/DC system which demonstrated outstanding performance in the detection of heavy metal ion (i.e. Hg 2+ ) in mussels [133]. LOD of the nanohybrids was ca. 1 nM. This system is appealing to researchers in the field of fluorescent nanomaterials for biomedical applications.
Drug Delivery
Until now, the technology of treating cancers with high efficiency and targeting function is not perfect enough. Under most circumstances, the anticancer drugs are distributed and released extensively in the body, which endangers the healthy cells and tissues irreversibly. Currently, a large variety of carriers for drug delivery have been designed. However, we can hardly supervise the distribution and result of the whole delivery process. Benefiting from the recent development of the surface modification technique, fluorescent materials capped with polymers like polyethylene glycol (PEG) can bond with drugs strongly and firmly. Then, the loaded drugs will be released in response to certain conditions such as pH, osmotic gradient and the surrounding environment. However, it should be confirmed whether the drugs are transported to the specific site or not. It’s also necessary for us to consider more details such as how much of the drugs is released in different positions. Aside from being drug carriers, fluorescent nanomaterials can also demonstrate the consequences of intracellular uptake due to their fluorescence property. QDs have been applied to monitor some important properties, such as delivery efficiency, release rate and distribution of drug molecules in vivo, which are beneficial for scientists in order to understand the specific targeting pathways of drug delivery within living cells. Duan and co-workers reported a facile pH-responsive fluorescent CDs drug delivery system [134]. Loaded with dox which is effective for gastric cancer, intracellular drug delivery and tracking could be simultaneously realized in patients (Fig. 7). The report highlighted the ability of fluorescent CDs to label and track the drug delivery process for at least 48 h, which showed a great potential in bioimaging, biolabeling and traceable drug delivery. Duan et al. designed a pH and receptor dual-responsive drug delivery system [135]. Hyaluronic acid was covalently attached to the surface of CDs, and doxorubicin was loaded by electrostatic self-assembly. In the tumor microenvironment (pH 5.6), the drug is released rapidly from the drug delivery system, while in the normal physiological environment (pH 7.4), the drug is hardly released. Endocytosis occurs when the drug delivery system reaches CD44 which is a receptor rich in tumor cells and can bind specifically to the hyaluronic acid. In addition, carbon nanotubes can be used for drug delivery by virtue of their high loading efficiency. Strong π-π interactions play a critical role in binding therapeutic agents with carbon nanotubes, which can be broke through changing external conditions, resulting in the release of drugs in specific position. Pennetta et al. functionalized single and multi-walled carbon nanotubes with a pyrrole derived compound to form a doxorubicin stacked drug delivery system. Biological studies showed that the synthesized nano-conveyors can effectively deliver the drug into cell lines and improve the therapeutic effects of doxorubicin [136].
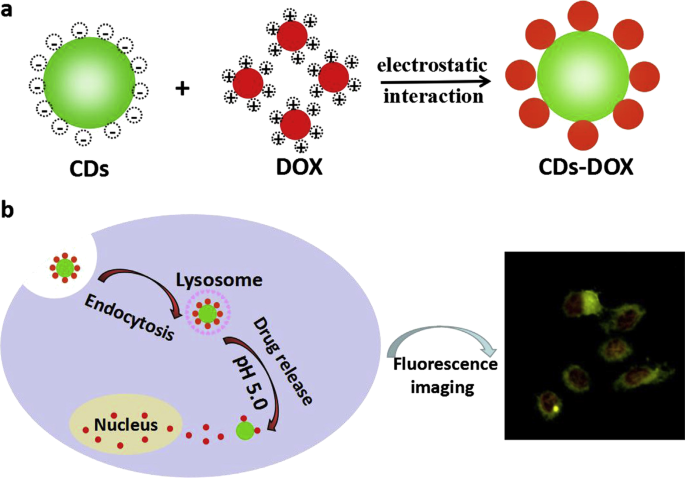
Schematic illustration of the preparation (a ) and cellular uptake (b ) of the CDs-DOX drug delivery system [113]
图>Photodynamic Therapy
Photodynamic therapy (PDT) is a novel therapy method for tumors which utilizes the interaction between light and photosensitizer. In PDT, reactive oxygen species (ROS) is produced from oxygen by photosensitizers in the condition of specific wavelengths of light (mostly in the area of near infrared light). The specific mechanism is presented in Fig. 8. ROS includes singlet oxygen, superoxide radicals, hydrogen peroxide, and hydroxyl radicals that possess strong cytotoxicity which cause significant destruction of tumor cells. However, there exist many defects such as limited penetration depth [137], hydrophobic properties [138], photobleaching [139], complicated procedure [140], and tumor hypoxia. PDT agents can hardly be dissolved and they will disperse extensively in vivo once they are taken, making it impossible to be targeted and selected. Fluorescent nanomaterial based photodynamic therapy developed fast in recent years [141]. Combined with the unique properties that QDs possess, such as high fluorescent efficiency and great spectral resolution, the effect of PDT can be enhanced. Barberi-Heyob, M and coworkers significantly enhanced the photodynamic efficiency with a concentration of 8 nM because of the light dose-dependent response [142]. In addition, photodynamic therapy can sometimes do harm to the skin and eyes of patients due to its photosensitive side-effect. To alleviate these adverse effects, a novel nanoparticle-based drug carrier for photodynamic therapy is reported which can provide stable aqueous dispersion of hydrophobic photosensitizers. Meanwhile, the key step of photogeneration of singlet oxygen was preserved, which is necessary for photodynamic action [143]. It is obvious that QDs combined photodynamic therapy will replace the conventional PDT someday.
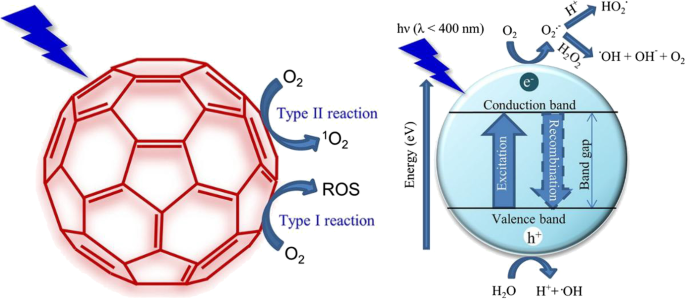
Schematic illustration of producing reactive oxygen species (ROS) for the photodynamic therapy (PDT) [119]
图>Challenges
Synthesis Challenges
Achieving Uniform Distribution
In the synthesis process, the diameter and size distributions of FNMs can be hardly distributed uniformly due to the agglomeration of small particles. This could be fatal to the optical properties of FNMs in biomedical application. For this reason, the applications of FNMs are still at the laboratory scale. It has been confirmed that the surface properties primarily determine the agglomeration state of the nanoparticles and their size. Therefore, surface modification is promising to achieve uniform distribution of FNMs by altering their surface properties [144]. To date, silanized QDs have been widely used because the polymerized silica coating increases the stability in buffers under physiological conditions [145]. Carbon dots synthesized by hydrothermal reaction using water-soluble base were reported to be difficult to control the size and distribution of grain boundary [146]. Khanam et al. reported a facile and novel synthetic method for the preparation of hydroxyl capped CDs using an organic base and a surfactant (Triton X-100) to modify the surface. A narrow particle size distribution at 7.2 nm was found in Raman and DLS studies, which is smaller than the majority of the particles falling within the range of below 10 nm in diameter [147].
Fluorescence Quantum Yield
Fluorescence quantum yield plays a crucial role for FNMs in their efficiency for on-demand light emission. Tunable and highly fluorescent CDs can be prepared with the surface functionalization approach. Nitrogen-doped FNMs are reported to have improved fluorescence quantum yield. With increasing nitrogen content, fluorescence quantum yield can be increased to as high as 56% at high synthesis temperature [148]. A facile strategy was also developed to tune the photoluminescent properties of CDs using a microwave irradiation, with citric acid and nitrogen-containing branched polyethyleneimine (b-PEI) as precursors. At intermediate levels of b-PEI, the CDs produced a high photoluminescence yield [149]. Lin et al. explored carbon dots with a high-fluorescence quantum yield rate synthesized from L-cysteine and citric acid by the microwave-assisted method. The obtained carbon dots exhibited a high-fluorescence quantum yield (up to 85%), which is due to the combination of amidogens and sulfydryl with carbon dots, and henceforth bringing the improved fluorescence property [150]. The above examples demonstrate that nitrogen or other electron-rich atoms like sulphur can obtain satisfying fluorescence quantum yield.
Aggregation-Caused Quenching
Fluorescent molecules can emit light with high efficiency in dilute solution. However, in concentrated solution or solid state, their fluorescence will be weakened or even disappear. This phenomenon is called Aggregation-Caused Quenching (ACQ) [151]. This problem has been puzzling scientists for almost 150 years, thus hindered the extensive application of fluorescent dyes.
In order to make effective use of fluorescent dyes, scientists have attempted many methods. Most of them focused on reducing the concentration of fluorescent dyes to prevent ACQ effect. Tang et al. discovered the phenomenon of Aggregation-Induced Emission (AIE) [152]. Based on rationally designed molecules, the fluorescence of organic molecules in solid state can be attained. Still, for more than one hundred thousand different fluorescent dyes in the world, the problem of ACQ has not been completely resolved. As long as they aggregate together, ACQ will make them lose their fluorescent properties.
It is almost impossible for high concentration or solid state FNMs to show reliable fluorescence due to fluorescence quenching. Although fluorescence quenching can be used as a sensitive signal to indicate substrate concentration in analytical chemistry, [153] in the most circumstances, however, fluorescence quenching is undesirable for FNMs because it always has considerable influence on bioimaging and biodetection. To overcome this long-standing problem, Benson et al. reported a universal solution with the discovery of a class of materials called small-molecule ionic isolation lattices (SMILES) [154]. SMILES are simple to make by mixing cationic dyes with anion-binding cyanostar macrocycles. We draw inspiration from their findings and believe that similar results can be obtained if we replace cationic dyes with cationic modified FNMs.
Application Challenges
Drawbacks of UV Light FNMs
Although FNMs realized the great-leap-forward from in vitro imaging to in vivo imaging, the emission fluorescence of most of FNMs is distributed in ultraviolet region or short wavelength visible region, which limits the optical imaging in living organisms. Moreover, use of UV light for monitoring living processes in cells and tissues has some potential drawbacks as long‐term irradiation of living cells may cause DNA damage and cell death. Therefore, the development of FNMs in near-infrared region is urgently needed in the future. Although NIR FNMs have deep tissue penetration, NIR detectors and filters are needed as the excitation and emission wavelengths are too close to each other, which restricts their range of application.
Interference in Biological Environment
Almost all biological tissues will produce significant autofluorescence under short wavelength, UV and visible light radiation [155]. Autofluorescence reduces the signal‐to‐background ratio and often interferes seriously with the visual effects. Some substances in the substrate of biological tissues also have great influence on the fluorescence, which reduces the selectivity of FNMs significantly. Until now, although the application of FNMs in mice showed acceptable outcomes, it is still difficult to achieve similar results in larger mammals. Much higher luminous efficiency under low power density excitation is required to avoid the background signal interference. Furthermore, temperature and pH conditions of the biological environments strongly affect the fluorescence of some substances as well. Therefore, satisfying fluorescence of FNMs at 37 °C and physiological pH should be guaranteed. It's worth noting that the pH in tumor is lower than normal tissues. Hence, fluorescence with high selectivity in acid environment will improve the efficiency of FNMs.
Biocompatibility
Biocompatibility refers to materials or systems that are nontoxic, safe and not causing physiological or immunological reactions. QDs with unique quantum confinement effect and electro-optical properties are attractive for biomedical applications. However, toxic effects of traditional semiconductor QDs made of heavy metal ions have serious safety concerns for their undesired environmental or health effects. In the purpose of circumventing this problem, core–shell structure modification of QDs by using biocompatible ligands or polymers is one way to effectively minimize toxic effects of traditional QDs. Furthermore, scientists are searching for heavy metal-free QDs formulations. Non-toxic or less toxic carbon dots and silica nanoparticles have shown their potential as the ideal FNMs for biomedical applications. Impurities brought in the process of syntheses may influence the biocompatibility of fluorescent nanomaterials. In order to reduce the influence of toxic impurities, green synthesis methods have been arousing the interest in biomedical fields. Chowdhury et al. utilized cacao extract which is a natural product as a reducing and stabilizing agent in the synthesis of gold nanoparticles [156]. Oxalic acid, as a constituent of cacao, can reduce Au 3+ in HAuCl4 to metallic gold and stabilize the resultant nanoparticle colloidal solution. In vitro studies suggested that the cacao derived gold nanoparticles are biocompatible and suitable for biomedical applications. For MOFs, appropriate metal ions and ligands must be selected to lower the toxicity.王等人。 employed Fe, Ti and Zr as constituents of MOFs, which are harmless and even beneficial elements to the body [157]. In vitro studies indicate that the proposed material has good biocompatibility and safety in biomedical application. What’s more, it is necessary to consider whether the difference in composition, surface charge, or modified group will have different biological effects. Taking these factors into account, we can improve the biocompatibility of FNMs with rational design.
Conclusions
Benefiting from the unique properties of fluorescent nanomaterials, some limitations and barriers of conventional materials and methods in biomedical applications can be broken through. In this review, we comprehensively present the synthesis methods and applications of fluorescent nanomaterials. The advanced synthesis methods can offer us the fluorescent nanomaterials with ideal morphology, size ranges and structures. Meanwhile, the more convenient syntheses can lower the manufacturing cost of fluorescent nanomaterials, which is critical to their widespread applications in biomedical fields. Based on the improved synthesis techniques, the performance of fluorescent nanomaterials is bound to leap in their applications. With the development of fluorescent nanomaterials, bioimaging, biodection, drug delivery and photodynamic therapy will be more widely applied in the diagnosis and treatment of diseases. Finally, challenges in synthesis and biomedical applications point out exiting questions and developing direction. We hope that this review can bring some new insights to the development of fluorescent nanomaterials.
Availability of data and materials
All data and materials are available without restrictions.
Abbreviations
- FNMs:
-
Fluorescent nanomaterials
- PL:
-
Photoluminescence
- CDs:
-
Carbon dots
- QDs:
-
Quantum dots
- b-PEI:
-
Branched polyethyleneimine
- SWCNTs:
-
Single-walled carbon nanotubes
- CQDs:
-
Carbon quantum dots
- GQDs:
-
Graphene quantum dots
- NCQDs:
-
Nitrogen-doped carbon quantum dots
- NSCDs:
-
Nitrogen and sulfur doped carbon dots
- QY:
-
Quantum yield
- MCDs:
-
Multi-doped carbon dots
- MWCHTs:
-
Multi-walled carbon nanotubes
- GO:
-
Graphene oxide
- rGO:
-
Reduced graphene oxide
- CVD:
-
Chemical vapor deposition
- AuNPs:
-
Gold nanoparticles
- Ag NCs:
-
Ag nanoclusters
- Cu NCs:
-
Cu nanoclusters
- NPs:
-
Nanoparticles
- TEOS:
-
Tetraethylorthosilicate
- MR:
-
Magnetic resonance
- COFs:
-
Covalent-organic frameworks
- TNP:
-
2,4,6-Trinitrophenol
- MOFs:
-
Metal organic frameworks
- ACQ:
-
Aggregation-caused quenching
- NIR:
-
Near infrared
- SEA:
-
Strand exchange amplification
- LFA:
-
Lateral flow assay strip
- CS:
-
Complementary strand
- LOD:
-
Limit of detection
- UC:
-
Upconversion
- DC:
-
Downconversion
- PDT:
-
Photodynamic therapy
- ROS:
-
Reactive oxygen species
- AIE:
-
Aggregation-induced emission
- SMILES:
-
Small-molecule ionic isolation lattices
纳米材料