具有高级农业化学活性的智能纳米材料和纳米复合材料
摘要
传统农业仅依赖于对每个生物和整个生态系统的健康产生负面影响的高度化学化合物。因此,以可持续的方式将所需成分智能地输送到作物植物是未来几年保持土壤健康的主要需求。促生长成分的过早流失及其在土壤中的长期降解增加了对可靠新技术的需求。在这方面,纳米技术已提出彻底改变农业技术领域,该领域具有超越传统农业的迫在眉睫的潜力,并有助于改革弹性种植系统,为不断增长的世界人口提供突出的粮食安全。此外,对植物-纳米颗粒相互作用的深入研究通过提高作物产量、抗病性和有效养分利用为作物改良开辟了新途径。将纳米材料与智能农化活动相结合,并建立一个与提高功效相关的新框架,最终有助于解决未来的社会接受、潜在危害和管理问题。在这里,我们强调纳米材料或纳米复合材料在作物保护和生产中作为可持续且稳定的替代品的作用。此外,本文还讨论了控释系统的信息、与土壤和微生物组相互作用的作用、纳米复合材料作为纳米农药、纳米除草剂、纳米肥料的前景及其在农业化学活性中的局限性。
<图片>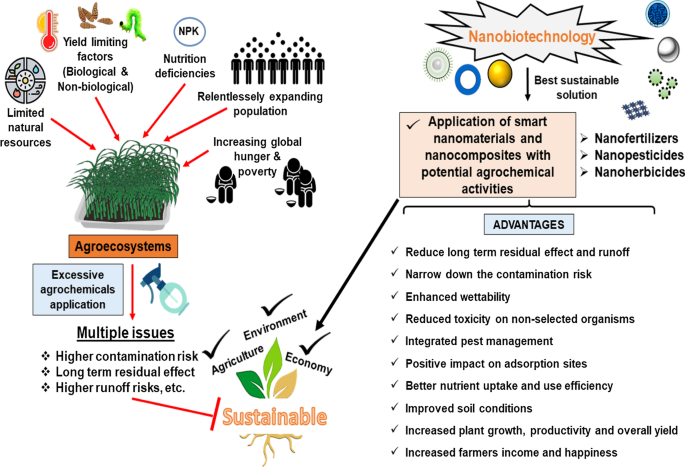
介绍
在全球范围内,人们受雇于农业以种植基本粮食作物和各种必需品,如纤维、燃料、饲料和原材料。有限的资源和呈指数增长的人口(估计到 2050 年将达到 96 亿)迫使衍生的地区要求制定非常可持续的农业,同时允许减少全球饥饿和贫困 [1, 2]。为了满足这种不断扩大人口的需求,迫切需要将粮食产量提高 50% 以上 [2, 3]。由于自然资源(水、土地、土壤、森林等)数量有限且作物生产力有限,因此对经济和生态友好且可行且可靠的有效农业方法存在巨大需求。为了克服这些困境,已经开发出合成农用化学品(除草剂、杀虫剂、杀菌剂和肥料)并用于提高农业产量 [4, 5]。然而,在过去几十年中,此类农用化学品的应用有助于提高食品质量和数量,以评估此类农用化学品对土壤健康和生态系统的长期不良影响 [6]。然而,在过去十年中,纳米颗粒应用作为农业部门效用的化学替代品的研究越来越受欢迎,后来被称为纳米农用化学品 [7]。就可预期的环境问题而言,在环境中有意和定向输送纳米农用化学品可能被认为是特定的,因为它们将代表工程纳米颗粒 (NPs) [8, 9] 的单一扩散原因。鉴于此,采取的一项此类举措是智能纳米材料的前沿,用于彻底改变当前的农业实践,由于它们具有良好的反应性,因为它们具有巨大的表面积与体积比和特殊的物理化学特性,可根据不断增长的需求提供新的改性优势 [2]。
现代农业正在通过使用这些能够从有限资源中获得最大产出的现代材料改造为可持续农业 [10]。一般来说,农用化学品对于提高作物生产力至关重要,但相反,它们的应用会通过阻碍土壤矿物质平衡而降低土壤肥力 [11]。此外,直接叶面或喷洒可能具有成本效益且非常高,这会流失并需要控制[12]。在农业中开发的基于纳米材料的化学品可调节养分消耗率、产量减少、作物种植、保护、生产的投入成本,并最大限度地减少收获后损失 [3]。纳米复合材料由于其固有的独特的热、电、化学和机械性能,已成为研究和刺激植物生命周期的纳米材料的关键组成部分。大小依赖的易位在植物部分内的 0.1-1000 nm 范围内,并根据表面组成、NP 的电荷(带高负电荷显示更多易位)和植物大小排除限制而改变 [10, 13]。这些渗透途径通过使用纳米农药的不同体外(滤纸、水培、琼脂培养基、Hoagland 溶液、Mursashige 和 Skoog 培养基、营养液)和体内(叶面吸收、分枝取食、树干注射和根部吸收)实验得到证实、纳米除草剂、纳米除草剂和纳米生长促进化合物 [2, 9]。然而,在某些情况下,尺寸排阻很高,因此很难限制对植物生长阶段产生正面和负面影响的特定传代和浓度(图 1)。
<图片>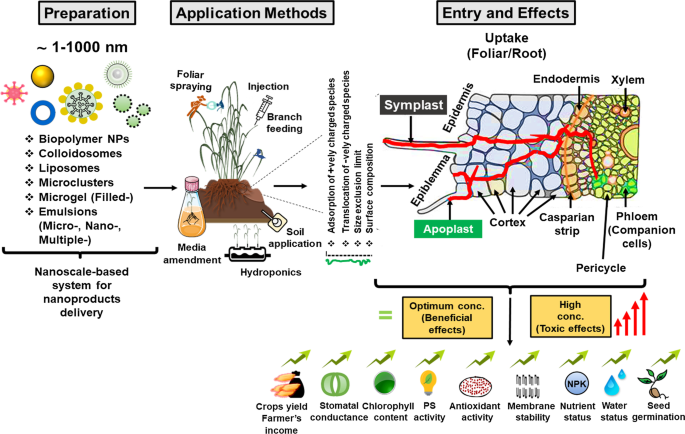
纳米粒子在作物中运输及其相互作用的示意图
图>近年来,已经报道了许多在农业中利用智能纳米材料的成功例子,包括多壁碳纳米管 [5, 14]、金属基纳米复合材料 [15]、银抑制真菌萌发 [16] 等等。这种新时代的纳米制剂有可能微调刚刚进入土壤-植物复合体的生理学,可以单独利用它来发现横向效应[17]。
基于纳米颗粒的产品(NMs)包括以纳米复合材料为主要成分的智能农化输送系统正在不断开发中。仍需要进行大量深入研究,以通过改进工作设计、商业化监管以及纳米肥料、纳米农药和纳米除草剂的风险评估来实现纳米农用化学品的实际优势 [18, 19]。可以承受高温、干旱、盐度和农业系统中其他未解决挑战的新作物品种扰乱了全球主要种植实践的整个范围。此外,预计在自然环境中实施 NMs 会降低基于化学品的危险水平 [12]。我们坚信,它们在农业中的应用将缩小可持续农业系统与化学农业系统之间的差距。除此之外,它还通过解决水和土壤污染问题,以环保的方式在全球范围内提高粮食产量和质量[20]。因此,实际上它们可以提供关于开发新的基于 NM 的产品的新途径 [14]。传统农药在活性成分(AIs)的非选择性和吸附率方面存在诸多缺陷。
据报道,超过 99.9% 的农药未能到达目标地点,对土壤、水、空气的健康造成有害影响,增强了病原抵抗力和生物多样性丧失 [12, 21, 22]。总体而言,我们旨在强调当前有关纳米材料或纳米复合材料提供有效解决方案以升级和推进农业创新、粮食系统、可持续作物保护和生产的事实的信息。此外,本文还讨论了控释系统、与土壤和微生物组相互作用的作用、纳米复合材料作为纳米农药、纳米除草剂、纳米肥料的前景以及对农业化学活性的限制等方面的信息。
具有受控释放系统 (CRS) 的纳米结构化合物
由于优于传统化学应用方法的几个优点,许多研究人员提出了控释系统模型 [15, 23,24,25,26,27,28,29] 以提供替代品以减少环境污染。控释 (CR) 允许在所需的时间间隔内更积极地在土壤和植物中有效递送 AI,从而减少对操作应用仪器至关重要的农用化学品、能源、人力或其他资源的使用量,如以及提高处理其应用程序的人类的安全性 [26, 29,30,31,32]。此外,CR 显示出优于传统方法的许多优点,包括降低植物毒性,减少由于挥发、浸出、漂移、不当处理和土壤降解造成的农用化学品损失,并且控制输送与植物中合适的浓度相吻合,以防止以蒸发形式出现不可预测的损失, 浸出和天气 ( 图 2) [16, 33]。
<图片>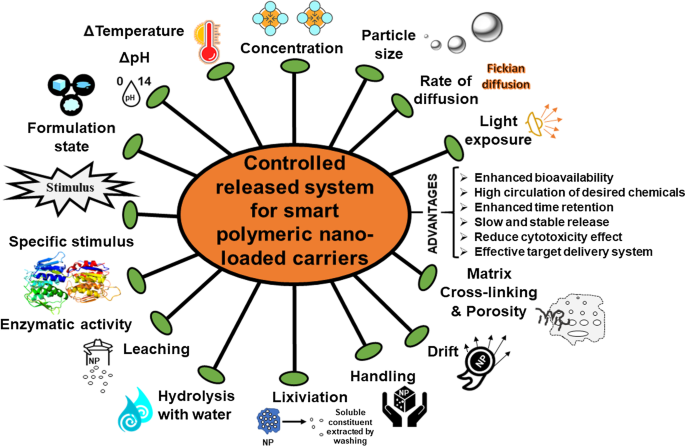
纳米粒子传递系统的类型
图>综合表征是预测或解释智能纳米负载农用化学品的效率和行为的重要先决条件。特别是,AI 的保留、行为、组成和相、zeta 电位和聚合物纳米载体的内部结构,以及它们在粒子环境条件下的释放被总结为重要的特性 [30, 34,35,36]。从纳米载体加载和释放 AI 的速率在预测或评估其功效方面起着核心作用。这些可以通过聚合物基质中残留的成分浓度和释放成分的量来评估 [37, 38]。释放机制可以通过不同的方式实现,例如:
通过纳米颗粒的松弛/膨胀进行扩散
在浓度梯度现象(或菲克扩散)中,即使在灌溉或降雨事件下,使用浓缩或固体制剂稀释纳米载体时,也会以高速率释放。可以通过增加纳米颗粒尺寸或增加介质内的距离来减慢扩散,在这些介质中,在载有聚乳酸 (PLA) 的吡草胺中观察到 AI 发生扩散 [32, 39, 40]。类似地,如聚合物交联前后装载灭多威的壳聚糖(叠氮苯甲醛-羧甲基)农药所表明的那样,增强的交联被认为是一种通过聚合物基质增加曲折度或降低孔隙率来延迟扩散的有效方法[40, 41,42,43]。
突发发布
最常见的快速释放方法,如果初始大量的 AI 不利于目标的应用,则 AI 会不合需要地释放。该现象将表明存在于 NPs 附近或表面上的 AI 浓度增加表明高显着的爆发释放。例如,载有PLA的吡草胺(除草剂)纳米胶囊或表面涂层已被推荐用于抑制纳米球常见的初始快速爆发[35]。
退化
纳米粒子的释放可以通过物理、化学和生物降解来触发或加速,这些降解可以通过水水解、光照、温度、pH、特定刺激和酶活性来实现。例如,PLGA(聚乳酸共乙醇酸)NPs 显示出随着水的表面积-体积比的增加而增加的水解降解,并且它们的扩散速率可以通过适当的纳米载体进行微调 [44]。此外,PLGA-NPs 中掺入的 mPEG(甲氧基聚乙二醇)通过增强亲水性和最终水解降解类型的水解可及性来提高 NPs 的降解率。在酶促降解中,由磷酸酶、糖苷酶和蛋白酶的活性引起的事件,即:PCL(聚(ε-己内酯)降解随着脂肪酶活性的增强而增强 [44]。类似地,γ-PGA(聚(γ-谷氨酸)酸)降解由 γ-GTP(γ-谷氨酰转肽酶)介导的被认为是导致快速降解的最常见酶 [38]。在另一项研究中,玉米醇溶蛋白纳米颗粒显示包封的环丙沙星抗生素的快速和广泛降解和释放,在胰蛋白酶酶高于胶原酶[37]。
在某些情况下,可以使用光敏聚合物观察到刺激反应释放,例如胶束或 UV(紫外线)不稳定的核壳 NPs 生成到 PEG 和硝基苄基到羧甲基壳聚糖。因此,基于刺激的纳米复合材料可以对目标或邻近环境产生的刺激做出智能反应,最终触发 AI 释放以有效调节害虫 [45, 46]。然而,当聚合物呈弱碱性或酸性时,某些 NP 的物理稳定性会因 pH 值而改变,因此静电和电荷在 pH 值下是可靠的 [40, 41, 47]。例如,羧甲基纤维素和羽毛角蛋白加载了阿维菌素。观察到在低 pH 值(Fickian 传输)和较高 pH 值(非 Fickian)下扩散速率更快 [46]。
纳米制剂作为农业系统中很有前途的工具
农用化学品包括杀虫剂、除草剂、杀菌剂、杀菌剂、杀线虫剂、杀鼠剂,用于针对害虫、杂草、病原真菌、细菌、线虫和啮齿动物(图 3)[48,49,50]。在全球范围内,除草剂市场正在扩大,预计在 2016 年至 2022 年期间的复合年增长率 (CAGR) 为 6.25%,预计将在 27.21 至 391.5 亿美元之间。除此之外,到 2021 年全球农药市场将达到 705.7 亿美元,预计 2016 年至 2021 年间的复合年增长率为 5.15%。除此之外,全球封装农药市场呈指数增长,预计到 2025 年将达到 8 亿美元的基准并获得收益2019-2025年间复合年增长率为11.8% [18, 19, 48, 49]。
<图片>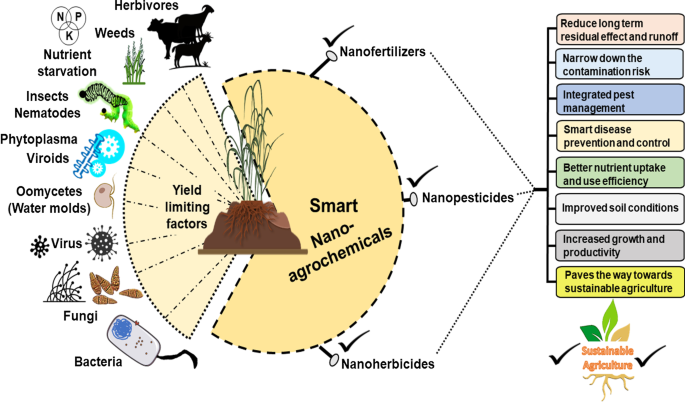
不同纳米颗粒在植物生长调控、病原体管理和养分吸收中的应用在可持续农业中
图>以无机化学品为代表的家族有三嗪、苯氧基和苯甲酸氯乙酰苯胺代表除草剂,苯基吡咯、苯并咪唑、二硫代氨基甲酸酯和腈类杀真菌剂、氨基甲酸酯、有机磷酸盐、与杀虫剂有关的有机氯。与传统化学品相比,具有纳米制剂的智能纳米农用化学品必须提供广泛的益处,包括增强的耐用性、有效性、润湿性、良好的分散性、较低的毒性、在土壤和环境中的良好生物降解能力,以及具有最少残留物的光生性质 [51,52,53 ]。过去,人们对纳米农用化学品进行了广泛的研究,以了解它们在影响土壤-植物养分循环方面的重要作用和污染范围[19]。
纳米农药
纳米化学品在害虫综合管理 (IPM) 中的潜在效用取决于有针对性地递送具有至少药物浓度增加活性的 AI,以及对农药与周围环境相互作用的熟练监测。在恶劣的条件下,化学稳定性可以通过有效的纳米载体来实现,这些载体具有更大的分散范围、润湿性和对杀虫剂的更多保护,而没有流失的风险 [54,55,56,57]。其他值得注意的杀虫纳米组合物的特征可以在热稳定性、大表面积、增加的目标亲和力和成功交付后的生物降解性方面观察到。这些传递系统可以针对单个目标或多个组合进行调节,即;空间目标释放、时间控制释放、远程或自我调节释放以克服成功目标中的生物障碍 [21, 58,59,60]。然而,纳米封装或纳米载体的功效是 (1) 防止载体中的 AI 在其释放到目标中之前发生预降解 (2) 提高 AI 的渗透性和缓解目标位点内的溶解度 (3) 以监测或调节AIs在目标位点的降解[61, 62]。
根据克雷默等人的说法。 [63] 农药和纳米颗粒之间的吸附相互作用显示出离散的分子动力学。这种相互作用应该通过生理形态、结合能力、抗氧化系统和农药在植物中的转运能力对吸附位点产生积极影响 [64]。在拟南芥 , 银纳米颗粒与甲基双氯芬酯(芽后除草剂)之间的拮抗作用,其中除草剂的存在下降或影响了 Ag + 来自银 NP。此外,必须降低农药浓度,以避免它们对非选定生物体产生毒性并缩小污染风险 [65,66,67]。已经开发了几种农药的纳米组合物,例如纳米乳液、纳米悬浮液和纳米胶囊。此类纳米材料专门用于通过多种方式维持 AI 的调节释放,包括磁释放、超声释放、pH 释放、热释放、水分释放、基于 DNA 的释放、特异性释放、快速和缓慢释放 [19]。>
在某些情况下,中空二氧化硅 NP 中的纳米颗粒递送用于防止阿维菌素免受紫外线辐射,并为纳米农药提供光稳定性,从而对目标生物产生长期影响。几个 NP 使用了各种形式的封装,包括 (1) 基于脂质纳米材料的封装。 (2) 基于金属-有机框架的封装。 (3) 基于聚合物的 6 封装。 (4)基于粘土纳米材料的封装。 (4) 绿色封装[9, 42, 43, 45, 47, 68,69,70]。
纳米肥料
除了植物保护,这些智能 NPs 还广泛用于调节生理过程。例如,SiO2 NPs(二氧化硅 NPs)可提高 Lycopersicon esculentum 的种子发芽率 [71, 72] 壳聚糖-聚甲基丙烯酸-NPK 增加了菜豆的生物量、养分吸收和抗氧化酶 [73, 74], Au-NPs(金 NPs)促进 Zea mays 的种子萌发、幼苗生长、酶活性和养分吸收 [75, 76], SiO2-NPs 提高了Hyssopus officinalis 的NPK 吸收,提高酶活性和种子发芽率 和 Z。可能 [77, 78, 79],壳聚糖-CuNPs(铜NPs)增强了Z中的种子萌发、α-淀粉酶、蛋白酶和各种抗氧化酶的活性。可能 [2, 80, 81] 壳聚糖-ZnNPs(锌纳米颗粒)增加了硬粒小麦中锌含量和防御酶的积累 [82, 83],壳聚糖-γ-聚谷氨酸-赤霉酸纳米颗粒促进种子萌发、根发育、叶面积、激素效率、细胞外酶和营养效率 [83、84],壳聚糖-聚甲基丙烯酸-NPK纳米颗粒促进蛋白质含量和养分吸收 [74, 85],ZnO-NPs(氧化锌 NPs)增加过氧化氢酶的活性(60.7%),超氧化物歧化酶(22.8%)和养分获取 [86, 87],CeO2-NPs(氧化铈 NPs)增强菠菜的种子萌发和活力、酶活性和养分吸收 和 Z。可能 [88,89,90,91], AuNPs提高芥菜的叶绿素含量和抗氧化酶活性 [92] 和 TiO2 NPs(氧化钛 NPs)可提高 S 中的叶绿素含量、养分吸收、Rubisco 和抗氧化酶的活性。甘蓝 和 Cicer arietinum [89, 93](表1)。
图>纳米杀虫剂
随着封装 NP 的趋势和需求呈指数增长,对其管理的监管压力也同时增强。截至 2017 年,包封杀虫剂占农药总收入的 42% 以上 [60, 94, 95]。最近,2019 年在线农药手册分类的包封杀虫剂中含有危险的有毒 AIs,如二甲戊灵、乙草胺、敌菌灵、七氟菊酯、依托芬酯、毒死蜱、百威威、呋喃硫威等商业化水平 [19]。 AI 的毒性水平不仅取决于封装材料,而且有助于调整目标物种在体内条件下暴露于 AI 的动态 [21, 25, 96]。使用苯乙烯和甲基丙烯酸甲酯作为包封壁材料提高了杀线虫活性以抑制引起小麦锈病的病原体Puccinia reconditea的生长 .类似地,Zhang 等人报道了尿素甲醛和聚脲树脂壁对气孔毒性、接触毒性、辛硫磷微胶囊功效和光解特性的影响。 [97]。在另一项研究中,观察到海藻酸钠吡虫啉包封的害虫效率提高和细胞毒性较差,有利于直接施用吡虫啉[68]。
另一项研究表明,与自由形式的 picloform 相比,硅胶封装的 picloram 对土壤微生物群的毒性降低。通过调整二氧化硅壳的壁特性,可以提高二氧化硅 NPs 对非选择生物的生物利用度 [98]。在一项研究中,雅克等人。 [99] 报道了封装的聚合物和脂质纳米组合物中的阿特拉津对线虫、秀丽隐杆线虫的毒性 ,但相比之下,在基于三聚磷酸盐/壳聚糖的封装中没有观察到毒性,这本身可归因于低毒性。此外,油包封的 PCL 印楝衍生纳米胶囊在暴露 300 天后未表现出对气孔导度、玉米光合能力的任何不利影响。这些发现表明,AIs 的壁材料/封装和理化性质及其组成和应用部位需要谨慎选择 [19, 100]。
据报道,Si-NPs(硅 NPs)可有效保护储存的甲虫Callosobruchus maculatus 在豆类中,例如Vigna unguiculata、V. mungo、V. radiate、Macrotyloma uniflorum、C. arietinum 和 Cajanus cajan [101]。尽管纳米农药具有优异的性能,但其商业化和稳定性较差。 pH 值、温度、湿度、紫外线辐射影响 AI 的可用性并影响理化特性。除了这些数量、质量、严格的法规、昂贵的 AI 和降解期等问题外,纳米农药在使用过程中也面临着新的问题 [19, 54, 79]。
纳米杀菌剂
除了纳米载体应用,纳米材料作为作物保护的人工智能是研究的一个主要方面。纳米杀真菌剂的广谱抗真菌特性可以提高它们作为杀虫剂的效率。例如,铜、银和锌 NPs 解决了化学 AI 对病原体耐药性的缺点,具有显着的抗菌活性和无毒性 [19]。此外,在过去十年中,许多研究人员报告称,基于壳聚糖的 NPs (Ch-NPs) 显示出有效的抗真菌活性并限制其生长。例如,Ch-NPs 对抗Alternaria alternata, Macrophhomina phaseolina , 立枯丝核菌 [102], Pyricularia grisea, Alternaria solani , 尖孢镰刀菌 [102, 103], 稻瘟病菌 铜-壳聚糖纳米粒对抗茄病镰刀菌 [104],Cu-壳聚糖NPs-针对R。索拉尼 和 Sclerotium rolfsii [105], 壳聚糖-皂苷 NPs [102], 油酰-壳聚糖 NPs 对抗 Verticillium dahaliae [106], 负载水杨酸的壳聚糖纳米粒对轮枝镰刀菌 [107],Ag-壳聚糖 NPs 对抗 R。 solani, 黄曲霉 和A。替代 [108], 二氧化硅-壳聚糖纳米粒对Phomopsis asparagi [109] 壳聚糖胡椒树 (Schinus molle ) 精油 (CS-EO) NPs 对抗 Aspergillus parasiticus [110] 壳聚糖勃姆石氧化铝纳米复合薄膜和百里香油对抗松散念珠菌 [111] 杀菌剂 Zineb (Zb) 和壳聚糖-Ag NPs 对 Neoscytalidium dimidiatum [112] 壳聚糖-百里香-牛至、百里香-茶树和百里香-薄荷 EO 混合物对黑曲霉、黄曲霉、寄生曲霉 , 和 Penicillium chrysogenum, [113], 壳聚糖-百里酚 NPs 对抗灰葡萄孢 [39], 壳聚糖-Cymbopogon martinii 精油对抗禾谷镰刀菌 [114].
与传统的农用化学品相比,纳米颗粒被证实即使在微量浓度下也能非常有效地保护作物,即:0.43 和 0.75 毫克/板浓度的掺银中空氧化钛 (TiO2) 纳米制剂可对抗马铃薯病原体,例如 不等文丘疹 和F。索拉尼 [115](表2)。此外,近年来,针对非生物胁迫耐受性广泛研究了几个成功的 NP 实例 [116,117,118]。为了应对耐旱性,过去几十年发表的几篇关于纳米颗粒的应用的报告,如 TiO2 在 Linum usitatissimum 中的应用 通过提高色素沉着和降低丙二醛 (MDA) 和过氧化氢 (H2O2) 的活性 [119],ZnO 促进 Glycine max 中的有效种子萌发 [120],CuNPs 改善了 Z 中的色素沉着、生物量和谷物产量。可能 [121]。在盐胁迫的情况下,种子浸泡、营养液和种子引发方法用于在G. max、S. lycopersicum 和 Gossypium hirsutum 分别为 [122,123,124]。
图>该应用通过提高叶绿素含量、生物量数量、可溶性糖含量、种子发芽来提高胁迫耐受性 [125,126,127]。根据 Shoemaker [128] 在 Triticum aestivum 中应用 AgNPs(银 NPs) 增加幼苗生长和叶面积,而叶面施用硒纳米颗粒(硒纳米颗粒)可提高 双色高粱 的抗氧化酶活性和类囊体膜稳定性 热应力下[129](表3)。
图>纳米除草剂
这些 NPs 抑制了几种杂草的生理过程和生长阶段。例如,Ch-NPs 延迟 Bidens pilosa 的萌发和生长阶段 [130, 131] NPs atrazine 破坏了Amaranthus viridus 中的 PSII 活性 [132], Fe3O4 NPs(氧化铁 NPs) + 纯化硅藻土 + 草甘膦降低了狗牙根的 pH 值 [133],零价铁纳米颗粒(铁纳米颗粒)延缓了多年生黑麦草的萌发 [32]。通过使用 NPs 来维持杂草种群的生长,包括Melilotus album、T. aestivum、Agrostis stolonifera,提高了metribuzan(一种商业除草剂)的功效 和狗尾草 [19].
载有莠去津的纳米载体用于穿透气孔区域、水体并确保它们直接进入维管组织。由于 NPs 的某些特性,它确保了靶向、细胞摄取并克服了细胞内运输:(1)相互作用亲和力。 (2) 形状和尺寸的机械效应。 (3)催化作用。 (4) 表面电荷/疏水性。弗莱塞托等人。 [19] 描述了在 Brassica 中比传统喷雾系统更喜欢使用三磷酸盐/壳聚糖纳米载体的非靶向植物中百草枯的毒性水平降低 sp.同样,在 B.毛毛 和C。指尖 使用封装的草甘膦磁性纳米载体提高了幼苗的死亡率 [19, 131]。纳米胶囊使用低剂量的除草剂,可以有效降低除草剂在目标物种和农田中的长期残留效应。综上所述,纳米除草剂可以增强植物组织中AIs的释放,相对降低环境毒性的机会[60, 94, 95]。
对植物-土壤微生物组的影响
NPs 面临着大量的经验转化、土壤微生物群中的溶解聚集、与关键调节剂的吸附,这些调节剂介导了有机含量、pH、二价阳离子和粘土(对于 NPs 的保留最重要)的降解命运。根据 Asadishad 等人的说法。 [134],AgNPs 的毒性取决于微生物底物对氨氧化细菌的呼吸作用,随着 pH 值和粘土含量的升高而降低。低 pH 值会导致 AgNPs 溶解,而高土壤 pH 值会增加负电荷位点数量并导致 Ag 吸附增加 [19]。在一项研究中,关于 CuONPs(氧化铜 NPs)在低粘土含量和有机质与粗糙土壤质地的研究中报告了类似的结果。这种酸性土壤有利于 Ag 和 CuNPs 的溶解并释放出游离离子,这可以提高 NPs 的短期影响 [9]。翟等人。 [135] 还得出结论,离子农药的纳米制剂可以显示出不同的影响,更常见的是与部分离子释放相关。其他作者指出了离子和纳米形式的 AgNPs 的差异和相似之处,其抗菌活性或对土壤微生物群落的影响及其在体外条件下的反应 [19, 136, 137]。
In long-term studies, Guilger et al. [66], ensuring routes predictably depend on biogenic NPs, that show the least effect on human cells and denitrification process but are likely to show more impact on plant fungus relationship. At the microscale level, denitrification is a prime microbial activity that gets affected by AgNPs by modulating hydric conditions, pH and creating a devoid zone for fundamental accessories (carbon, nitrate, and oxygen). However, by high soil redox potential value and sandy texture soil favored denitrification, whereas textured clay soils provided offers low redox potential and lies in range for biological transformation [19]. Such impact is correlated by the affinity of AgNPs to denitrification and physicochemical properties ex:surface charge, coating, size, sedimentation rate, dispersibility, and solubility [138]. The biogenic AgNPs are derived from the green process and have no effect on N-cycle reported by Kumar et al. [67]. While the effect of nanocapsules, nanogels, nanometal, and nonmetal particles on soil microbiota as non-selected microbes has been documented.李等人。 [139] evidenced the negative impact of nanopesticide CM-β-CD-MNPs-Diuron complex (carboxymethyl-hdroxypropyl-β-cyclodextrin magnetic NPs) on the activity of the urease enzyme.
The Diuron NPs complex causes declined in the population status of soil bacteria except for actinobacteria with an increase in reactive oxygen species. All these indicate toxicity of CM-β-CD-MNPs-Diuron exert stress on soil microbes and did not reduce even by using Diuron nanoencapsulation [12, 19]. The bionanopesticides treatment was confirmed to improve soil microbiome including weight gain and survival percentages in beneficial earthworm Eudrilus eugeniae . It also shows excellent larvicidal, antifeedant, and pupicidal activities against Helicoverpa armigera and Spodoptera sp. at 100 ppm nanoformulation dose [19, 50, 55].
Drawbacks using nanoagrochemicals on plants
The nanopesticides are also showing some adverse effects on crop plants directly or indirectly. The most favorable and used AgNPs and their complex nanoparticle have been attributed to their diverse range in each class of pesticides due to low toxicity but still many reported published that explained the drawback of these smart nanoagrochemicals [61, 140, 141] (Table 4). For example, In Vicia faba , the AgNPs internalization in leaves can abrupt the stomatal conductance CO2 assimilation rate and photosystem II [142]. Furthermore, the binding of AgNPs attaches with Chlorophyll forming a hybrid, that excites electrons 10 times due to fast electron–hole separation and plasmon resonance effect. In another study, AgNPs and AgNPs-graphene oxide GO (Ag@dsDNA GO) effect also observed in L. esculentum exhibit antibacterial activity toward Xanthomonas perforans [143]. Various reports were submitted in recent years such as ZnO NPs reduced root growth in Allium cepa [89], Ch-NPs + paraquat biomass reduction, lipid peroxidation, genotoxicity and leaf necrosis in Brassica sp. [144], SiO2NPs affect biomass, germination, protein content, photosynthetic pigment in Taraxacum officinale and Amaranthus retroflexus [76], AgNPs cause lipid peroxidation, leaf damages and alters catalase activity in G. max [145], NPP ATZ + AMZ Raphanus raphanistrum suppresses plant growth [146].
图>Besides these, NPs show an adverse impact on plant physiology, soil microbiota, and declined enzymatic population. For instance; Al2O3 (Aluminium oxide) reduces bacterial growth and reduces seedling growth [147, 148], C60 fullerene restricts bacterial growth up to 20–30% [149], ZnNPs decrease enzymatic activities in soil and reduces transpiration rate and photosynthetic rate in Z. mays [150]. Conclusively, NPs are very reactive and variable in nature, so always a concerning risk for workers who may come across during their application.
Limitation and challenges at commercial scale implementation
As with documentation, the lack of finding on behavior and fate in the environment of nanoagrochemicals and their impact on faunal diversity may put challenges on their incorporation in agriculture. Instead of the benefits of using nanoencapsulation systems, their implementation requires caution, since it is mandatory to calculate their behavior in the environment and non-targeted communities to develop safer product development policies [54]. Although, it needs to develop smart nanoagrochemicals that are focused on biological nanoformulation and that offer a simple handling process, low cost, more AIs persistence with a sharp release system, and high degradation rate without leaving any residue [148]. Besides these, poor demonstrations at field conditions, cost-effectiveness, consumer acceptance, and feasibility of technology are major constraints on commercial implementation [152].
The limited management guidelines, inconsistence legislative framework, and regulatory models, and lack of public awareness campaign creates inconsistent marketing of such incipient nanoagricultural products. The national and international arrangement that fits at ground level is the only way that supports Nanotechnological development [49]. However, the community seeking approval for nanoagrochemicals must demonstrate the precautionary uses of these new products by proposing unjustifiable safety risks to the user and environment. Thus regulatory guidelines and frameworks are becoming primarily important to resolve the emerging issues of nanoagrochemicals [153]. Moreover, the need for collaboration, discussion, and information exchange forums among countries to ensure threat mitigating strategies should be considered as a milestone in nanoagrochemicals. So consolidates efforts of governmental organizations, scientists, and social communities are needed to preventing the adverse effect of nanoagrochemicals on humans and the environment [59].
In this scenario, the toxicity measuring instrumental setup is used in the characterization of toxicity type and their level to access the potential intrinsic hazards [59]. Currently, the main focus of experimental investigation on nanomaterial translocation in biotic/abiotic systems, monitoring and revealing interaction Among nanotoxicity and nanomaterial in the physical and chemical environment [48, 54, 151,152,153].
Transformation
Due to high reactivity, the interaction of nanocomponents with organic and inorganic components in the soil as well as for plants is undetermined and unregulated. The changing in physiochemical properties and transformation behavior after implementation creates chances of heavy metal toxicity. Biotransformation was demonstrated in Cucumis sativa, using CeO2 bioavailability cause 20% to Ce(III) in the shoots and 15% of Ce(IV) being reduced to Ce(III) in the roots [154]. In another study, AgNPs were oxidized and forming the Ag-glutathione complex in the lettuce plant [154].
Accumulation of NPs
Because of variability in binding, the accumulation of NPs causes toxicity in plants, humans, and animals. In soybean, CeO2 application shut down the Nitrogen fixation cycles and causes toxicity. However, ROS production, growth inhibition, cellular toxicity, and other phytotoxic effect were reported in Amaranthus tricolor . The application of C60 fullerene enhanced DDT accumulation in soybean, tomato, and zucchini plants [155].
Time to switch toward more sustainability
Most agrochemicals are not fully utilized by plants or seep off into the soil, air and water unintendedly causes toxic ill effects and accumulated through biomagnification. Moreover, global pesticide rise threatened biodiversity and led to the adverse effect on human intelligence quotient and fecundity in recent years. Still, it’s also enhancement the resistance in weeds and plant pathogen against agrochemical turn them to super pathogen/weed. New doses after the changing in strategies of pathogens or new strain resurgence enhance cost-effectiveness and put the question on existing regulatory recommendations. [14, 106, 156,157,158].
The chemicals persist in soil particles, agricultural residues, irrigation water and migrates into the different layers of soils turns into a serious threat to the ecosystem. Leaching of synthetic pesticides, abrupting soil-pest, soil-microbe activities, algal blooms formation, eutrophication, altering soil physiochemical properties [159], and salt toxicity via creating salt buildup in soil [160].
Low-cost oxides of Mg, Al, Fe, Ti, Ce, and Zn (Magnesium, Aluminium, Iron, Titanium, Cerium, Zinc) are ideal candidates and provides greater affinity, a large number of active sites, minimum intraparticle diffusion distance, and maximum specific surface area [160]. NP implementation help to successfully chase down the inorganic residues of various chemicals such as permethrin, 2–4 Dichlorophenoxy acetic acid (2–4-D), Dichlorodiphenyltrichloroethane (DCPT), Diuron (Adsorption), Chlorpyrifos, Chloridazon, Methomyl (Photocatalysis) from the soil. Some nanocomposites are used for complete degradation of lethal agrochemicals for example silver- doped TiO2 and gold doped TiO2, Zerovalent Fe (nZVI), endosulfan, TiO2, nZVI for atrazine, Ag for chlorpyrifos, Pd–Mg, Ni–Fe bimetallic system, nZVI for DDT, nZVI, nitrogen-doped TiO2, Fe–Pd (iron–palladium), Fe–S (Iron-sulfur) for Lindane [161] (Table 5).
图>Smart agrochemical:a step ahead toward more sustainability
Al-Barly et al. reported the slow release of nanocomposite fertilizers to depend upon phosphate and nitrogen content availability in soil [162]. TiO2 NPs derived from Moringa oleifera leaf extract are used to control the red palm weevil (Rhynchophorus ferrugineus) and exhibits antioxidant and larvicidal activities. In the case of Zanthoxylum rhoifolium , nano-encapsulated essential oil was reported to maintain the population of Bemisia tabaci [19, 163]. Nanopesticides derived from pyrethrum insecticides cause an impact on the population status of honey bees. Except for these studies, agrochemical degradation can also be accomplished using adsorption, membrane filtration, catalytic degradation, oxidation, and biological treatment. Since, adsorption using smart Nanosorbents also relies on environmental factors including pH, temperature, and competitive adsorbing molecules [19]. At low pH, the protonated charged active site of NPs disturbs the binding ability of positively charge agrochemical whereas, high temperature creates hinders the electrochemical interactions between active sites and agrochemicals due to elevated vibrate energy of active site of adsorbent and kinetic energy of agrochemicals [79]. Moreover, chitosan-coated and cross-linked chitosan-Ag NPs used as composite microbeads that incorporated into reverse osmosis filters help in the effective removal of atrazine content from the water. According to Aseri et al. [164] integration of membrane filters and magnetic NPs-based beads enhances microbial elimination and resonance activation of water, respectively.
Secondly, targeting a not selected species with possible adverse effect is a key issue emerging that put a loophole of criticism for these smart nanoagrochemicals. For example; 1–10 mg L −1 of Polyhydroxybutyrate-co-hydroxyvalerate (PBHA) encapsulation for atrazine in lactuca sativa for 24 h reduced genotoxicity in plants [165], PCL atrazine nanocapsules ill effect on Daphnia similis and Pseudokirchneriella subcapitata, after exposure up to 24 h [166], Solid lipid NPs encapsulating simazine 0.025–0.25 mg mL −1 exhibits Caenorhabditis elegans Induction of mortality and decrease in the body length after exposure of 48 h [167]. The uncontrolled non-targeted release of AIs in plant cells causes lysosomal damage with increasing pH. After the cellular compartment, nanoagrochemicals may bind or channelization into cell organelles and causes damage to protein, pigments, and DNA [98].
The binding ability of nanocompositions with selected and non-selected binding helps to recognize its distribution, bioavailability, toxicity level, and exclusion from the plant cell. Several proteins acquire a wide range of functional and structural properties including ligand boding, metabolite production, catalysis, cellular and molecular reorganization [19]. The protein- nanopesticide complex can cause minor structural configuration and denaturation of proteins. Similarly, conformational changes and movement of the genomic DNA mediated through NPs also induced cytogenetic abnormalities. These nanopesticide toxicity are solely dependent upon the balance between key factors like biodegradability, concentration, and size of incorporated AIs. In Prochilodus lineatus 20 μg L −1 concentration using PCL nanocapsules containing atrazine up to 24–48 h declined toxicity, as they did not induce carbonic anhydrase activity, alterations in glycemia and antioxidant response [168], in Enchytraeus crypticus causes a decrease in hatching due to the delayed number of adults and juveniles [19, 158, 169].
No doubt, intervention of nanoagrochemicals, resolve many threats mitigation put forward by the implementation of agrochemical but still more validation is required to lowering the agroecological risks. The persistent use of novel monitoring applications always knocks down the door of improvement of sustainable crop production and protection without creating the threats of NPs as a new contaminant.
Conclusion and future perspectives
During the entire course of million years of evolution, the green plants had evolved without any interference from other eukaryotes. However, for the last fifty years, continuous human activities have introduced many contaminants in the environment that altered the ecological balance and raised the eye-brows of researchers towards combating the new pathovars and pathotypes. These thrusting biological stresses have severely damaged global crop production. Concerning, the environmental penalty of conventional agrochemicals at present, nanoformulations seem to be a potential applicant for plant protection. The use of controlled biodegradable polymers especially polyhydroxyalkanoates shows significant and attractive properties of biocompatibility, biosorption rate, low-cost synthesis, thermoplastic nature, and ease in biodegradation rate that have popular advantages conventional chemical delivery systems. However, sustainable and efficient utilization with promising target delivery and low toxic effects are prerequisites of commercial implementation. Although, the studies on the soil–plant microbiome and nanoscale characterization highlight the impact of chemical agrochemical on the environment.
The use of nanocoated AIs biopesticides is expected to surpass the challenges of chemical residual management gap and premature degradation of AIs. Instead, these, applying new nanocomponents along with existing chemicals should follow regular checks on resistance strategies of targeted organisms, new resistance pathways, and revolutionized pest strains. Although, smart agrochemicals or nanoagrochemicals resolve so many issues and gives an instant solution.
To ensure these, it is essential to develop more international and national risk assessment, management, and mitigating strategies. Beyond these challenges, social acceptance with reduced environmental cost chiefly soil deterioration, microbiome disruption, depleted water resources need keen monitoring. Ecologically, the continuum uses of agrochemical put the question on survival challenges result in more resistance races creating a vicious loop in which pesticides concentration help to revolutionizing the organism more toward superiority.
For this, alternative strategies with strong monitoring are required, together recommendations of IPM practices help to eliminate shortcomings in individual practices. Despite the advancement in studies on nanoformulation and plant response more extensions in genomic, proteomics, physiological, and metabolic studies help to understand the interaction in the mechanism.
数据和材料的可用性
Not applicable.
缩写
- NPs:
-
Nanoparticles
- NMs:
-
Nanomaterils-based products
- AIs:
-
Active ingreadents
- CRS:
-
Controlled release system
- CR:
-
Controlled release
- PLA:
-
Poly lactic acid
- PLGA:
-
Poly(lactic-co-glycolic acid)
- mPEG:
-
Methoxy polyethylene glycol
- PCL:
-
Poly(ε-caprolactone
- γ-PGA:
-
(Poly (γ-glutamic acid)
- γ-GTP:
-
(γ-Glutamyl transpeptidase)
- UV:
-
Ultraviolet
- PEG:
-
Polyethylene glycol
- CAGR:
-
Compound annual growth rate
- IPM:
-
Integrated pest management
- Ag + :
-
Silver
- SiO2NPs:
-
Silicon dioxide nanoparticles
- Ch-polymethacrylic NPK:
-
Chitosan polymethacrylic nitrogen phosphorus potassium
- Au-NPs:
-
Gold nanoparticles
- ZnO NPs:
-
Zinc oxide nanoparticles
- CeO2-NPs:
-
Cerium dioxide nanoparticles
- TiO2NPs:
-
Titanium oxide nanoparticles
- S. oleracea :
-
Spinacia oleracea
- Si NPs:
-
Silicon nanoparticles
- V. mungo :
-
Vigna mungo
- V. radiate :
-
Vigna radiate
- C. arietinum :
-
Cicer arietinum
- Ch-NPs:
-
Chitosan nanoparticles
- CS-EO:
-
Chitosan essential oil
- MDA:
-
Malondialdehyde
- H2O2 :
-
Hydrogen peroxide
- PS II:
-
Photosystem II
- Fe3O4 NPs:
-
Iron oxide nanoparticles
- Fe NPs:
-
Iron nanoparticles
- T. aesitivum :
-
Triticum aestivum
- B. pilosa :
-
Bidens pilosa
- C. dactylon :
-
Cynodon dactylon
- AgNPs:
-
Silver nanoparticles
- CM-β-CD-MNPs-Diuron complex:
-
Carboxymethyl-hdroxypropyl-β-cyclodextrin magnetic nanoparticles diuron complex
- Ag@dsDNA GO:
-
Ag@dsDNA-graphene oxide
- L. esculemtum :
-
Lycopersicon esculentum
- Z. mays :
-
Zea mays
- CeO2 :
-
Cerium dioxide
- ROS:
-
Reactive oxygen species
- Mg:
-
Magnesium
- Al:
-
Aluminium
- Fe:
-
Iron
- Ti:
-
Titanium
- Ce:
-
Cerium
- Zn:
-
Zinc
- 2-4-D:
-
2-4 Dichlorophenoxy acetic acid
- DCPT:
-
DDT- Dichlorodiphenyltrichloroethane
- nZVI:
-
Zerovalent iron
- Fe-Pd:
-
Iron-palladium
- Fe-S:
-
Iron-Sulphur
- PBHA:
-
Polyhydroxybutyrate-co-hydroxyvalerate
- P. vulgaris :
-
Phaseolus vulgaris
- C. annum :
-
Capsicum annum
- S. oleracea :
-
Spinacia oleracea
- B. juncea :
-
Brassica juncea
- CNTs:
-
Carbon nanotubes
- Cu3(PO4)2 :
-
Copper(II) phosphate
- X. perforans :
-
Xanthomonas perforans
- B. sorokiniana :
-
Bipolaris sorokiniana
- X. alfalfa :
-
Xanthomonas alfalfa
- C. riparius :
-
Chironomus riparius
- CrBR2.2:
-
Balbiani ring protein gene
- CrGnRH1:
-
Gonadotrophin-releasing hormone gene
- D. melanogaster :
-
Drosophila melanogaster
- L. usitatissimum :
-
Linum usitatissimum
- G. max :
-
Glycine max
- SLN:
-
Solid lipid nanoparticles
- G. hirusutum :
-
Gossypium hirusutum
- PVA:
-
Poly vinyl alcohol
- S. lycopersicum :
-
Solanum lycopersicum
- S. bicolor :
-
Sorghum bicolor
- PVC:
-
Polyvinyl chloride
- PHSN:
-
Polystyrene nanoparticles
- O. sativa :
-
Oryza sativa
- SnO2 :
-
Stannic oxide
- H. vulgare:
-
Hordeum vulgare
- A. cepa :
-
Allium cepa
- T. repens :
-
Trifolium repens
- H. vulgare :
-
Hordeum vulgare
- S. tuberosum :
-
Solanum tuberosum
- MSN:
-
Mesoporous silica nanoparticles
- C. sativus :
-
Cucumis sativus
- B. cinerea :
-
Botrytis cinerea
纳米材料