油纳米胶囊:开发、应用和融入食品市场
摘要
油是人类营养中非常重要的物质。然而,它们对氧气、热、湿气和光敏感。近年来,人们对油品的改性技术越来越感兴趣。越来越多地研究了改变油特性并使油适合应用的方法。纳米技术已成为最有前途的研究技术之一,可以彻底改变传统食品科学和食品工业。油纳米胶囊可能是一种有前景的替代方法,可以提高纳米胶囊化合物的稳定性和生物利用度。油纳米胶囊化的出现迅速增加,尤其是在食品工业中。应用于不同油品的常规纳米封装技术对油品纳米颗粒的合成产生直接影响,影响zeta电位、尺寸和多分散指数等参数;这些特性可能会限制油在不同行业的使用。本综述总结了食品工业中的油纳米胶囊,并重点介绍了获得稳定油纳米胶囊的不同技术的技术、优势和局限性;它还说明了技术创新的关键机会和好处,并分析了通过专利申请对这项技术的保护。在过去的 20 年里,油纳米胶囊在食品工业中得到了长足的发展。虽然目前在食品工业中还没有发现纳米封装的油产品,但食品科学领域有大量文章报道称,纳米封装油将成为一种市场趋势。然而,正如通过专利申请所证明的那样,不同领域可以应用纳米胶囊油。
介绍
油在人类营养中具有重要作用。除了提供热量外,它们还是脂溶性维生素(如 A、D、E 和 K)的载体。油也是必需脂肪酸(如亚油酸、亚麻酸和花生四烯酸)的来源,它们有助于到食物的适口性。最具表现力的油脂成分是甘油三酯,这些甘油三酯的物理性质取决于现有脂肪酸的结构和分布[1,2,3,4]。
大约 90% 的油产品来自植物,来自种子加工,用于人类消费。在行业中,市场对来自各种天然来源的油的需求不断增加,特别是在用于配制蛋糕、饼干、面包、人造黄油和乳制品等产品以及用于油炸食品的食品应用中,其他应用[5, 6]。
剩余 10% 的石油产量用于动物饲料生产,并用于多种工业过程,例如制造杀菌剂、肥皂、洗涤剂、肥皂、可生物降解柔软剂、化妆品和生物柴油的原材料 [5]。
考虑到食用油的营养和经济重要性,近年来人们对这些油的改性技术越来越感兴趣。人们越来越多地研究改性技术以改变油的特性并使它们适用于某些应用。研究人员发现了各种技术来提高食品质量和安全性。纳米技术在食品工业中的应用导致食品生产具有更好的热稳定性、更好的溶解性和新的、更高水平的口服生物利用度[7]。
纳米技术已被建议通过延长食品的保质期、实现更好的污染物跟踪和追踪方法、创造改进的食品储存策略以及推进将保健品或抗菌剂纳入食品,对食品科学领域产生积极影响。因此,纳米技术确实对食品科学做出了巨大贡献[7]。
纳米技术已成为彻底改变传统食品科学和食品工业的最有前途的技术之一。纳米技术辅助加工和包装已证明纳米技术在食品系统中的重要性。不同的制备技术可以产生不同物理性质的纳米颗粒;因此,这些颗粒可用于食品[8, 9]。
封装是一个过程,其中生物活性脂滴被外壳回收或封闭在异质或同质基质中,以产生纳米级 [10] 的小胶囊 [3],其尺寸小于 1000 纳米,纳米是一米的十亿分之一 [3]。 11];封装具有许多有用的特性 [3]。根据 Gonnet 等人的说法。 [12],封装是一种潜在的方法,可以随着时间的推移保持天然/天然油的特性。纳米或微囊化开发的经典系统是基于储层或基质颗粒。
除了它的好处之外,纳米封装的特点是提高被封装的活性物质的生物利用度并保护它们免受自然和加工影响,如化学作用 [13, 14]、酶促作用和功能性加工过程中出现的物理不稳定性。 、保健品 [13]、药品和化妆品 [3] 产品 [10]。封装也代表了一种提高生物效率的手段,例如控制活性成分的传递和保质期,并可以防止副作用的出现[12]。
油包封可以防止或减缓氧化反应,考虑到这些系统可能构成对抗促氧化元素(如氧、自由基或紫外线辐射 (UV) [12, 15])的物理化学屏障,并拓宽了旨在用于浓缩目的。例如,生物活性油包封代表了一种有效、可行的方法,可以改善油的释放、防止环境氧化反应、增加物理稳定性、降低挥发性、降低毒性、增强生物活性,以及提高患者依从性和便利性 [3]。具体而言,在食品工业中,该技术可提高加工食品的品质,例如风味保留、抗氧化、保质期、颜色和异味;延长食品储存时间;保护成分免受环境影响,减少保存过程中的风味损失,控制生物活性物质的释放[16]。
许多技术被应用于封装。通常,三种方法用于生物活性剂的包封: (a) 在包封的药剂周围形成屏障结构; (b) 被污染的材料被拒绝进入; (c) 封装的药剂用于防止不希望的损害[17]。
在许多情况下,纳米胶囊化始于纳米乳剂的生产,纳米乳剂是由油相和水相形成的系统;在大多数情况下,通过使用乳化剂来乳化纳米胶囊。此外,形成的纳米乳液具有小液滴尺寸和高表面积 [18]。这些特性赋予它们优于传统乳液的潜在优势,例如良好的物理稳定性和更高的生物利用度 [19]。为获得油纳米乳液和油纳米胶囊而研究的一些技术包括纳米沉淀、喷雾干燥、离子凝胶、预制聚合物的界面沉积、乳液扩散、乳化-溶剂蒸发、脂质体的使用、高剪切均质化(微流化)、自发乳化和纳米结构脂质载体(NLCs)。
本研究的目的是调查油包封在食品工业中的潜力和当前应用,说明创新的主要好处和机会,并考虑未来的挑战,包括食品市场中的当前产品和专利申请。新的纳米胶囊油产品和专利申请为石油在各个行业领域的使用带来了希望。此外,微米和纳米封装可以促进 (a) 降低核心材料向外部环境的蒸发或转移速度; (b) 通过降低对外部环境的反应性来保护核心材料免于降解; (c) 控制核心材料的释放速度,无论是随时间缓慢还是在特定时间; (d) 修改原始材料的物理特性以使其更易于处理; (e) 掩盖核心材料的不想要的味道或味道; (f) 分离混合物组分,否则会相互反应; (g) 核心材料的稀释,只需少量即可在主体材料中实现均匀分散[17]。
胶体纳米颗粒的一般纳米封装
近年来,纳米粒子和其他纳米结构的合成受到了相当多的关注,因为它们的光学、机械和化学性质等性质在很大程度上取决于它们的尺寸、几何结构和成分,这些与大块材料的性质有很大不同。 20, 21]。
纳米粒子是胶体粒子。具有足够小颗粒以实现光学透明性的两种最常见类型的胶体递送系统是微乳液和纳米乳液。两个系统都包含小颗粒 (d <200 纳米)。与微乳液相比,纳米乳液的主要优点之一是它们形成所需的表面活性剂要少得多。食品级纳米乳可以通过高能方法(如高压均质或超声)或低能方法(如相转化温度、自发乳化或乳液相转化)形成[22]。
胶体颗粒可以用于不同的用途,例如在金属 [20]、生物医学 [23]、医学 [24]、传感器 [25]、光学 [25]、调味剂、饮料、驱虫剂、香水和化妆品中的应用;用于它们的药用特性 [26]、食品 [22] 和用于不同用途的精油 (EOs) [27, 28]。
胶体输送系统,包括乳液,可以设计成将多不饱和脂肪酸 (PUFA) 结合到水性环境中,以提高系统的氧化稳定性。大多数这些基于乳液的递送系统包含尺寸与光波长相似的颗粒,因此它们强烈散射光,导致高浊度或不透明度。对于某些应用,使用透明输送系统是有利的,这样它就可以加入光学透明的食品或饮料产品中,例如一些强化水、软饮料和调味品 [22]。
关于软饮料,Ziani 等人。 [29] 形成了含有柠檬油、非离子表面活性剂(吐温 80)和缓冲液(pH 2.6)的胶体分散体。该研究为合理设计食品级胶体递送系统提供了有用的信息,用于封装食品和饮料中的风味油和其他功能性脂质。
固体脂质纳米颗粒 (SLN) 在制药和食品工业中受到越来越多的关注,因为它们能够克服微胶囊和前面提到的纳米级胶体载体系统的缺陷。 SLN 是最新一代的纳米级封装系统,结合了母体液体纳米乳剂或微乳剂的优点,其高溶解速度与活性化合物通过肠壁的高渗透性相关,同时解决了与物理和相关的现有问题。封装化合物的化学稳定性和易处理性[30]。
具有固体颗粒基质的脂质纳米颗粒通过用固体脂质替代液体脂质(油)而衍生自 O/W(油/水)乳液。这些脂质通常是低毒性的生理脂质(生物相容性)[3]。 SLN 由脂质组成,在室温和体温下都是固体。 SLNs 的主要优点是它们的高封装效率、大规模生产的可能性、由于固体基质而在控释曲线方面的灵活性以及它们到达靶器官的高能力。然而,SLN 可以结晶,从而为油的掺入提供非常小的空间,因此负载能力较低 [31]。脂质纳米颗粒的直径可以在 50 纳米到 1 微米之间 [3]。 SLNs封装负荷低,储存过程中容易发生爆炸[31]。
米糠油纳米胶囊是使用聚(ε-己内酯)(PCL)作为壁材料合成的,以评估它们对 UVB 辐射引起的小鼠皮肤损伤的保护作用,作者得出结论,米糠纳米胶囊(200 nm,潜在的 zeta 为 - 9 mV 和 <0.2) 的低多分散指数 (PDI) 抑制了 60% 由 UVB 照射引起的水肿 [32]。
厄尔克等人。 [33] 用阿魏酸 (FA) 和生育酚 (Toc) 制备了 SLN。不同的配方,含有高达 2.8 mg g −1 FA 或 Toc 在室温下可稳定保存至少 15 周。作者得出结论,这些 SLN 适合作为食品添加剂,其中活性化合物的逐渐释放可能是有益的。
油纳米封装的趋势
过去 20 年的许多出版物都包含 4 个术语纳米封装、纳米乳液、纳米颗粒和纳米技术(图 1)。然而,在 2000 年代之前,包含这四个术语的关于 1990 年代后期开始的石油和食品应用研究的文章仅占所审查出版物的不到 2%,使该主题成为纳米技术的一个小领域(图 1)。> <图片>
Scopus 数据库中使用以下关键字的每年纳米胶囊、纳米乳液、纳米颗粒和纳米技术出版物的数量:a 纳米胶囊、纳米乳液、纳米颗粒和纳米技术; b 纳米胶囊与油、纳米乳剂与油、纳米粒子与油、纳米技术与油;和 c 纳米胶囊与食品与油、纳米乳液与食品与油、纳米粒子与食品与油、纳米技术与食品与油
图>纳米技术一词在许多出版物中被用作更通用的术语(图 2)。当使用这些术语和“油”(图 1b)的组合时,观察到涉及术语“纳米颗粒”的出版物增加。自 2010 年以来,无论是在一般领域还是与食品相关的领域,涉及“纳米乳液”和“油”的出版物数量都显着增加(图 1b)。
<图片>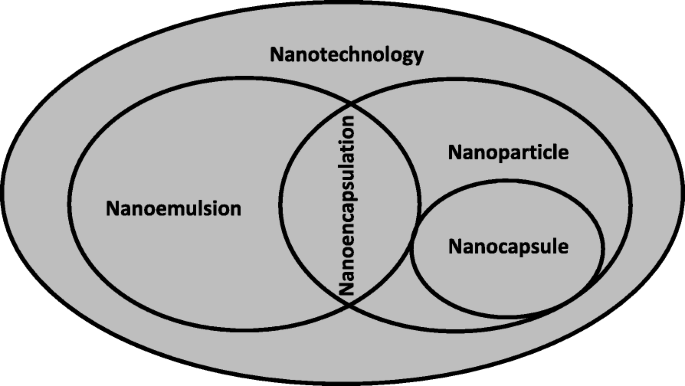
油类常用纳米封装定义示意图
图>尽管有更多的出版物涉及“纳米粒子”和“纳米技术”(图 1a),但封装是用于描述将物质包装成微米粒子和纳米粒子的最合适的术语,它被定义为涉及一种物质的过程,称为作为“活性剂”,在另一种产品中称为“壁材”[34,35,36]。
大多数关于油纳米胶囊的出版物使用术语“纳米胶囊”[2, 37,38,39,40,41,42] 或“纳米乳液”[10, 43,44,45,46,47,48]。一些作者使用术语“纳米胶囊”[49,50,51],而其他作者使用“纳米粒子”[35, 41]。然而,这两个术语最初的意思是“纳米封装”(图 2),它已被用于最广泛的意义上,包括纳米胶囊和纳米颗粒的形成 [52]。
术语“纳米粒子”是纳米球和纳米胶囊的统称 [17]。纳米胶囊具有带有液核的聚合物膜,其中活性化合物被限制在一个空腔中,该腔由被聚合物膜包围的内部液体核组成(核壳结构可以是亲脂的或亲水的)[3, 17]。另一方面,纳米球可以定义为固体胶体碎片,其中生物活性堆肥被扩散、捕获、封装并化学连接或吸附到聚合物基质中。聚合物基体形成多孔或固体基体,核可能会根据共聚物结构变成固体材料 [3, 53]。纳米颗粒通常涂有非离子表面活性剂,以减少免疫相互作用并有助于减少颗粒表面化学基团的分子相互作用(范德华、氢键或疏水相互作用)。纳米颗粒的细胞内摄取高于其他封装系统。根据所应用的方法学,纳米胶囊可以充当将活性材料保留到聚合物内膜的载体。从这些系统中释放出来的油可以通过解吸、扩散或侵蚀从纳米颗粒传输到目标组织[3]。
纳米乳液是纳米胶囊化的开始,纳米胶囊是由油相和水相形成的系统,并通过使用乳化剂对这些相进行乳化。此外,形成的纳米乳液具有小液滴尺寸和高表面积 [10, 18, 37, 54]。这些特性赋予它们优于传统乳液的潜在优势,例如良好的物理稳定性和更高的生物利用度[10, 19]。
1959 年,著名物理学家理查德·费曼 (Richard Feynman) 在他的演讲中讨论了第一个纳米技术定义底部还有很多空间 ,其中他描述了通过直接原子操作合成的可能性。 “纳米技术”于 1974 年由 Norio Taniguchi 首次使用。纳米技术在 1980 年代作为一个领域出现,从那时起,该领域的科学出版物和意识有所增加;该领域的研究在 2000 年代得到加强(图 1),科学、政治和商业方面的关注也在加强,导致争议和进展。此外,基于纳米技术进步的产品商业化开始出现[55]。
纳米技术是一个多学科领域,涵盖了广泛的材料、工艺和应用,包括化学、物理、生物、电子和工程科学。它专注于纳米级范围内物质的制造、表征和实验,几乎在 1 到 100 nm 之间。与生长表面积相关的最小粒径表现出独特而新颖的特性,并为技术应用创造了巨大的潜力[55,56,57]。
纳米技术可以推进热稳定性和储存稳定性、水溶性和生物活性物质的策略,提高食品使用的生物利用度,并增强食品的宏观特性,如味道、质地、工业过程和着色强度 [58]。各大食品公司都利用自己的研究部门设计将纳米技术应用于功能性食品的策略[59]。
油纳米封装应用的现状
食品学科的增长在图 1b、c 中被量化为在其摘要中包含关键词“食品”和“油”和“纳米胶囊”、“纳米乳液”、“纳米颗粒”或“纳米技术”的出版物总数;该信息显示为出版年份的函数。如图 1 中的趋势所示,食品纳米技术领域的大部分增长发生在 2010 年之后,这是因为九十年代后期大量的纳米技术研究以及适用于纳米颗粒工艺的食品级添加剂的增长。表 1 总结了工业油纳米胶囊的应用。
图>目前,食品行业的纳米技术产品价值达到10亿美元(主要包括用于健康促进产品、包装技术和草稿的纳米粒子涂层),未来10年它们有机会增加超过200亿美元.许多评论对引领食品纳米技术领域的研究小组以及私人和公共组织进行了出色的总结 [11, 13, 60]。
尽管许多评论 [11, 13, 55, 60, 61] 讨论了食品纳米技术投资和纳米技术在初级生产中的新兴应用,但在将油视为封装材料时,还没有涉及油纳米封装的评论。此外,关于纳米技术应用的评论有很多 [13, 53, 55, 61,62,63,64,65,66,67,68],其中大部分都集中在食品应用中的纳米技术 [13, 52, 55, 61,62,63,64,65,66,67,68]。
在食品工业中,微囊化工艺可用于多种原因,Desai 和 Park [4] 总结如下: (a) 核心材料通过减少其对外部环境的反应来防止降解; (b) 主要物质向外界环境的蒸发或转移速度降低; (c) 修改原始材料的物理特性,以便更容易处理; (d) 核心材料的释放被调整为随着时间的推移或在特定时间缓慢发生; (e) 核心材料的不想要的味道或味道被掩盖; (f) 实现了保持器材料的均匀分散; (g) 否则会相互反应的混合物组分被分离。这些应用也适用于油纳米封装。里考特等人。 [10] 和坎波等人。 [37] 研究了不同目的的高油酸棕榈油 (HOPO) 和奇亚籽油。第一项研究旨在为从 HOPO 获得的纳米乳液寻找最有利的微流化、形成和储存条件,第二项研究承诺提供替代品以保护油免受脂质氧化并提高溶解度和稳定性(表 1)。
库申等人。 [9] 肯定了上述关于食品微胶囊化的断言;微胶囊鱼油已被应用于面包中,以获得功能性健康益处。微胶囊化工艺掩盖了鱼油难闻的味道,这款面包在市场上已经可行。食品工业中化合物的纳米封装和添加是该技术的合乎逻辑的发展 [2, 68]。此外,氧化反应是脂肪、油和脂类食品的主要变质过程,会导致营养价值和感官质量下降,而油纳米胶囊通过形成纳米胶囊过程中形成的保护屏障来促进氧化减少,如前面说过[2]。
在他们的评论中,Walker 等人。 [47] 强调了使用纳米乳液封装、安全和释放 omega-3 脂肪酸的前景。这些携带系统可用于食品工业中含有这些生物活性脂质的饮料和强化食品,或者它们可用于补充剂或制药工业以增强功能性 omega-3 脂肪酸组合物的生物活性。
Sozer 和 Kokini [67] 简化了纳米技术在食品和食品包装行业的应用。食物益处的类型包括防止氧化;包封成分(水分或 pH 值)的控制释放;测试伪装;输送纳米胶囊营养物质、维生素和香料;食品系统中的病原体检测;食品安全;和质量分析。一些食品包装应用包括改进包装(气体和水分屏障、拉伸强度);通过活性包装、纳米添加剂、智能包装、营养品递送和控释延长保质期;自清洁包装的抗菌作用;以及运输过程中的产品状态监控。食品包装中的应用被认为是非常有前景的,因为它们可以提高食品的安全性和质量。这些应用包括能够与食品互动的智能包装。然而,对于食品工业中的油纳米封装应用,通常使用鱼油,纳米封装的目的主要是保护油免受脂质氧化以进行食品强化[34,38,40]。
可以看出,鱼油是微胶囊和纳米胶囊中最常用的油。它是不饱和脂肪酸和多不饱和脂肪酸的来源。人类可以产生大部分脂肪酸。然而,人类营养中必不可少的 omega-6 (n-6) 和 omega-3 (n-3) 脂肪酸无法由人体合成。因此,人类必须从食物中获取它们。摄入植物油(食用油),包括多不饱和脂肪酸,与心血管或神经系统疾病等慢性疾病的低发病率以及癌症发病率的降低有关 [3, 69]。
生物活性油通常因其营养特性而被应用,但与其使用相关的主要问题之一是储存过程中活性成分的损失 [70]。这是因为生物活性油含有 PUFA 和其他对氧气、水分、热和光敏感的物质(叶黄素、甾醇、类胡萝卜素、单萜、黄酮醇等)[71]。在氧化油中形成的产物包括许多自由基种类、初级氧化产物如脂质氢过氧化物和次级氧化产物如烃、醛、环氧化物和酮。其中一些产品会对生物组织产生负面影响 [72]。由于这种氧化,油的特性和营养价值会丧失,并产生令人不快的味道和气味[3]。
这些油中的其他活性化合物具有抗氧化、抗炎、抗病毒、抗菌、抗癌和/或组织再生特性 [73]。油中的多酚和生育酚具有重要的抗氧化活性。因此,抗氧化剂的特性和成分因油类型而异。因此,橄榄、向日葵、摩洛哥坚果和葡萄籽油含有高含量的抗氧化化合物 [72]。此外,甾醇、类胡萝卜素、叶黄素、黄酮醇和单萜等不稳定化合物的存在也有助于油的营养价值和健康特性[3]。
此外,EO 是由生物活性材料混合物组成的常见植物产品,它们提供潜在的生物活性化合物和新型分子模板 [74, 75]。 EO 由挥发性次级代谢产物组成,具有抗真菌、抗菌、抗氧化、抗炎、抗病毒和抗癌活性 [76]。 EO 效率取决于其化学成分、基因型以及环境和农艺条件 [77]。这些油的一些例子是百里香、薰衣草、薄荷、肉桂、茶树、迷迭香、桉树和柠檬草油,以及其他一些油。这些油已被证明具有抗菌特性,但极易氧化 [15, 27, 78]。
EO 被归类为天然生物活性分子,被认为适用于抑制食源性病原体的生长。然而,由于某些 EO 成分的高挥发性、EO 难以掺入水性配方以及食品的感官特性可能发生剧烈变化,因此将 EO 直接掺入食品中存在技术挑战。在具有抗菌活性的成分中,牛至、香芹酚、百里酚和γ-萜品烯已被用于食品中。
一些精油已被用于改善肉类、鸡肉和果汁等食物的微生物、感官和化学质量 [28, 79,80,81]。 Ghaderi-Ghahfarokhi 等。 [28] 纳米胶囊化百里香精油并用于牛肉汉堡。他们观察到,封装过程延长了百里香精油的保质期,并最大限度地减少了储存开始时活性化合物的蒸发。此外,百里香精油在储存过程中的缓慢释放可以保持甚至增加油的抗氧化和抗菌活性,直到冷藏结束。此外,与对照组相比,汉堡的红度和氧合肌红蛋白含量有积极的变化,游离百里香精油提高了牛肉汉堡的可接受性和感官质量。
有研究将食品中的精油作为天然防腐剂来提高食品安全和质量,替代有害的合成食品防腐剂[49, 82]。赫库拉诺等人。 [82] 包裹桉树并测定负载的纳米颗粒对单核细胞增生李斯特菌的抗菌作用 和肠炎沙门氏菌 细菌。作者观察到纳米颗粒的杀菌作用对革兰氏阳性菌比革兰氏阴性菌更有效,因为纳米胶囊油对S 表现出增强的活性 . 肠炎;这些纳米颗粒可用于食品的天然保鲜。
腰果胶 (CG),其结构类似于阿拉伯胶,是从 Anacardium occidentale 的分泌物中提取的杂多糖 ,一种常见于巴西东北地区的树。腰果胶能够与水相互作用,从而起到稳定剂、乳化剂和粘合剂的作用,可以很好地替代价格更高的阿拉伯树胶。 Herculano 等人使用了 CG。 [82] 封装Eucalyptus staigeriana 精油 (ESO) 以及来自制剂的胶囊的直径 (nm) 和 zeta 电位 (mV) 分别为 F1:153.80 ± 8.20 和 - 24.50 ± 0.45; F2:27.70 ± 3.42; - 14.47 ± 1.42,和F3:432.67 ± 41.47; - 10.45 ± 0.21。这些配方由F1:CG:ESO =2:1; ESO:吐温 80 =2:1; F2:CG:ESO =4:1; ESO:吐温 80 =2:1; F3:CG:ESO =2:1; ESO:吐温 80 =1:1。 F1和F2样品呈单峰分布,而F3呈双峰分布(纳米颗粒和微粒)。
Nanoencapsulation Methods Applied in Different Oils
In this review, 11 studies that used nanoencapsulated oils in the food industry were analyzed [10, 16, 35, 37, 38, 83,84,85,86,87], and 1 figure, Fig. 3 was made that describes the technologies, nanoencapsulated oils, and wall materials used. Generally, there are many methodologies for the production of nanocapsules containing oils, such as emulsion-diffusion [16, 38, 85], emulsification-solvent evaporation [83], high-shear emulsification [10, 87], spontaneous emulsification [84, 88], homogenization [37], spray drying [35], and the emulsion supercritical fluid extraction process [86] (Fig. 3a). In general, the techniques are similar, with some particular similarities between each of them.
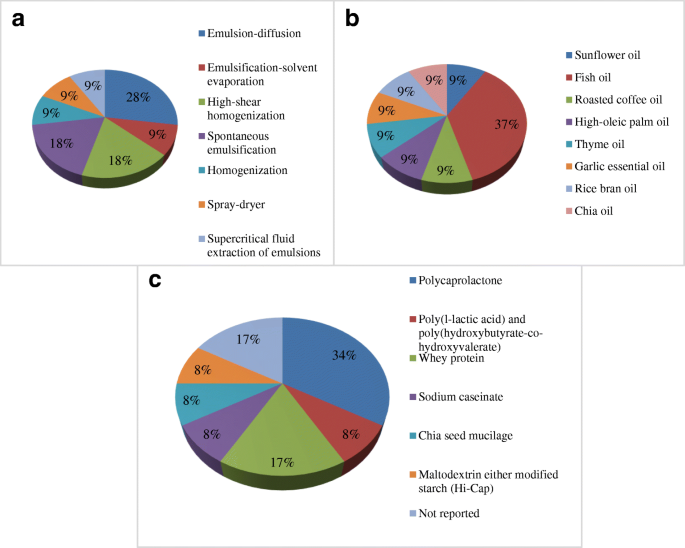
Proposal and techniques (a ), employed oils (b ), and wall materials (c ) of some studies using oil nanoencapsulation in food
图>In emulsion-diffusion, an emulsion is produced after a dilution causes the deposition of a polymer around the droplets, whereas in emulsification-solvent evaporation, an emulsion is formed with a polymer solution and an aqueous phase. The solvent is evaporated at the end of both techniques. High-shear homogenization, or microfluidization, is a kind of high-energy emulsification which uses microfluidizers to create mechanical shear. This equipment works by dividing a liquid jet into two parts. Every part passes through a narrow opening. Normally, emulsions with a diameter greater than 1 μm are first formed by other methods, after which their sizes are then reduced in a microfluidizer [3].
Spontaneous emulsification, or low-energy emulsification or self-emulsification, is a process which depends on different variables:interfacial and bulk viscosity, interfacial tension, phase transition region, and surfactant structure and concentration because the emulsion is formed spontaneously as a result of the low interfacial tension from high surfactant levels. In the homogenization (nonspecific name) technique, the emulsion is composed of an organic phase, which has a surfactant, organic solvent and oil, and of an aqueous phase, which is composed of water and a polymer. The organic phase is added dropwise to an aqueous solution. Then, the solvent is removed by a vacuum process [37].
The spray dryer technique is based on dissolving or dispersing the active ingredient in a biopolymer solution. Then, the dispersion is atomized in a heated air chamber that rapidly removes the solvent and produces a dried particle consisting of the active ingredient embedded in a porous wall material [31]. The supercritical fluid extraction of emulsions (SFEE) technique is based on the use of supercritical carbon dioxide (CO2) to rapidly extract the organic solvent from an oil-in-water emulsion, in which a bioactive compound and its coating polymer have been previously dissolved. By removing the solvent, both compounds precipitate, generating a suspension of particles in water [86].
In addition to that in oil microencapsulation, the oil usually used in nanoencapsulation is fish oil [16, 35, 38, 86] (Fig. 3b). However, sunflower oil [85], roasted coffee oil [83], HOPO [10], thyme oil [87], garlic essential oil [84], rice bran oil [88], and chia oil [37] are also used (Fig. 3b).
Oil nanocapsules have been produced with the application of different wall materials (Fig. 3c), depending on the usage and kind of oil to be nanoencapsulated. Unlike oil microencapsulation, oil nanoencapsulation does not usually involve wall material mixtures. Usually, the wall material is used alone because the particles formed must have a size of 1000 nm, and, as there is a larger contact surface, the fewer the compounds in the nanocapsule formulation the better the interaction is among the compounds, ultimately favoring the particle size.
The wall materials most used in the techniques are biodegradable polymers. Some usual wall materials used in oil nanoencapsulation are polycaprolactone [16, 38, 86, 88], whey protein [10, 35], sodium caseinate [87], chia seed mucilage [37], maltodextrin, or modified starch [35] (Fig. 3c). Some authors did not report the wall material used in their study [88], probably because of the spontaneous emulsification technique that was employed.
Wall material is chosen according to the size of the required nanoparticles, aqueous solubility and stability, and other factors. Among polymers, most of the wall material utilized is poly(ε-caprolactone) (PCL). PCL is a polymer obtained through the ring-opening polymerization of the cyclic monomer Ɛ-caprolactone utilizing cationic or anionic, coordination, or the radical catalysts mechanism. This polymer is semicrystalline, and its crystallinity is directly associated with its molecular weight. It is soluble in inorganic solvents and has a good blend compatibility that provides a transformation of chemical properties, such as solubility and porosity, and it presents a low melting point (59–64 °C). Furthermore, PCL is a synthetic, biocompatible, and fully biodegradable polymer that has a semi crystalline nature (glass transition temperature of 213 K). It is approved for drug delivery by the Food and Drug Administration (FDA). Due to its slow degradation, PCL is ideally suited for long-term delivery or when a targeted delivery to the intestinal tract is intended. PCL has a high hydrophobicity, high in vitro stability, and low cost [87]. Usually, PCL is utilized in the emulsion-diffusion method and supercritical fluid extraction of emulsions, especially for fish oil encapsulation [16, 86].
Whey protein may also be applied to nanoencapsulate bioactive compounds such as oils because of its functional characteristics, such as its surface activity, gelation, shielding, and protective properties, e.g., biocompatibility and biodegradability [58]. Ricaurte et al. [10] applied HOPO and obtained nanocapsules with whey protein from microfluidization, confirming that this methodology was able to create stable nanocapsules with a diameter of 163 nm.
After synthesis, the basic characterization of the oil nanoparticles is determined by important parameters, such as the size, polydispersity index (PDI), and zeta potential. The size and size dispersion of nanocapsules are important because of their ability to transform the physicochemical and pharmaceutical behaviors of the encapsulated ingredients [58].
Nanoparticle size, also named the mean diameter or z-average, may be established by several methods, such via laser diffraction (LD) and a Coulter counter; however, the most applied technique is dynamic light scattering (DLS) [58, 89], which allows the description of particle size distribution and destabilization phenomena. Nevertheless, it is not very precise when used with large size differences; it is noted that particles larger than 1 μm will be subject to gravitational movement in addition to Brownian motion, which makes this technique suitable for the characterization of particles only < 1 μm.
For nanoencapsulated oils, the diameter size is usually between 100 and 1000 nm [10, 16, 35, 37, 38, 83, 85, 87] or less than 100 nm [84, 86,87,88]. Diameters larger than 1000 nm were found by Ricaurte et al. [10]; those authors reported diameters between 163 and 2268 nm using the microfluidization method and whey protein as a wall material in the nanoencapsulation of HOPO.
Size dispersion is indicated as the PDI, an index that describes the particles uniformity in suspension; PDI values between 0.1 and 0.25 [10, 38, 87, 88] indicate a small size distribution, and PDI values higher than 0.5 indicate a broad distribution [50]. Although some authors, such as Choi et al. [16], Campo et al. [37], and Jafari et al. [35], did not report PDI, it is a good parameter for characterizing nanoparticles when used with particle size and zeta potential. Campo et al. [37] did not perform PDI analysis, but they found a bimodal figure in one of the diameter size results, suggesting the presence of nano and microparticles; if PDI was performed, the values would likely be greater than 0.25.
Zeta potential is a physical characteristic that is shown by particles in suspension, macromolecules, or substance surfaces; it corresponds to the nanoparticle’s electrical potential, as influenced by the nanocapsule ingredients and the medium in which they are distributed. This parameter is widely applied to indicate suspension stability in colloidal dispersions, where zeta potential values higher than 30 mV and lower than − 30 mV promote high stability and prevent particles aggregation [90]. The majority of the studies examined here obtained results between these values (30 mV and − 30 mV) [10, 37, 38, 84, 85]. Some authors, such as Choi et al. [16], Freiberger et al. [83], Bernardi et al. [88], Jafari et al. [35], and Pietro and Calvo [86], did not report the zeta potential.
For nanoencapsulated oils, the zeta potential is usually variable because of wall material characteristics. Campo et al. [37] obtained a zeta potential of − 11.58 ± 1.87 mV for encapsulated chia oil with chia seed mucilage as wall material. Nanoparticles of anionic gums, such as chia seed polysaccharide and cashew gum, can present negative zeta potential due to the presence of carboxylic acids groups in the carboxylate form (-COO-) that generates negative charges [82].
Another important analysis for the characterization of nanoparticles is Fourier-transform infrared spectroscopy (FTIR), which is a technique used to obtain an infrared spectrum of the absorption or emission of a solid, liquid or gas. An FTIR spectrometer simultaneously collects high spectral resolution data over a wide spectral range. This provides a significant advantage over a dispersive spectrometer, which measures intensity over a narrow wavelength range. FTIR is a less intuitive way to obtain the same information. Usually, oil nanoparticles are used in the transmittance mode, operating with wavelengths between 400 and 500 and 4000 cm −1 and a resolution of 4 cm −1 [37, 84, 91].
Based on FTIR analysis, it is possible to physically perceive the interactions that take place between the nanoparticle components; for example, the FTIR results of nanoencapsulated garlic essential oil showed the characteristic Tween 80 (the emulsifier used) peaks. This phenomenon could be related to coverage in the garlic oil nanoemulsion spectrum due to the stretching vibration of the extracted garlic bands. The band at 1325–1450 cm −1 showed the presence of S=O, and the band at 1675–1600 cm −1 showed a -C-C=C symmetric stretch, both of which are present in garlic EO compounds [84].
Incorporation of Nanoencapsulated Oils into the Food Market and Patent Application
According to the House of Lords [92], food currently contains structures at the micro and nanoscale. Fruit juice is composed by plant material that was built from nanoscale ingredients, while Bailey’s Irish Cream contained nanoemulsions with an average droplet size of 190 nm. Margarine had water droplets smaller than 10 μm across, with even smaller fat crystals interspersed in them. The naturally occurring nanomaterials found in food ranged from particles smaller than 100 nm found in drinks such as tea, beer, and coffee to protein structures of approximately 300 nm found in eggs or soy to larger oil particles of approximately 800 nm found in substances such as milk. All fresh and processed food was structured at the nanoscale, and consequently, the body evolved over time to deal with nanoscaled materials.
Few studies have been performed on the incorporation of nanotechnology incorporation into trade [65]. Furthermore, no products that contained oil nanoencapsulation were found in the market. However, there are numerous oil microencapsulation products that can be found in trade, and there is an article that highlights this information [93]. This finding may be attributed to the fact that, in general, nanotechnology is relatively new, and it is a relatively complex technology to employ. However, it is possible to notice some similarities between the methods used for the micro and nanoencapsulation of oils. In addition, the regulation gap in nanotechnology raises some uncertainties about the use of this technology in the market.
Concerning nanotechnology regulation, there are a number of ongoing EU research projects aimed at addressing all aspects of nanosafety, including toxicology, ecotoxicology, risk assessment, exposure assessment, mechanisms of interaction, and standardization. Examples of ongoing EU projects include the NanoLyse project, which is dedicated to the development of analytical tools for the detection and characterization of engineered nanoparticles in food, and the NanoReTox project, which seeks to address the human health and environmental implications of exposure to engineered nanoparticles [94]. However, regulatory institutions such as the Environmental Protection Agency (EPA) and the Food and Drug Administration (FDA) in the USA or the Health and Consumer Protection Directorate of the European Commission have started addressing the potential risks posed by nanoparticles. So far, neither engineered nanoparticles nor the products and materials that contain them are subject to any special regulation regarding production, handling, or labeling.
Although there is no specific nanoparticle regulation, there are some food industry patent documents deposited in different countries. WO2018029626, a patent application from Argentina, focused on chia oil with an edible nanoemulsion. It described a chia oil nanoemulsion comprising between 10 and 20% of chia oil (Salvia hispanica L. ), between 2 and 5% of polysorbate, between 0.5 and 5% of at least one emulsifier other than the polysorbate, between 0.05 and 0.2% of at least one antioxidant, and water. Formulations of edible chia oil nanoemulsions used in transparent drinks and desserts, such as juices and jellies, were disclosed [95]. A patent application from the Republic of Korea, KR20160005182, focused on cinnamon oil nanoemulsions to inhibit the development and increase of dangerous food microorganisms. Furthermore, this invention could not only be used for food additives, food packaging materials, preservatives, etc. but also be utilized in the pharmaceutical and cosmetic industries [96]. A mustard oil nanoemulsion application patent from China, CN103315956, was prepared to alleviate the pungent smell of mustard oil to avoid volatilization, and the mustard oil may be used for bacterial resistance in food and drugs [97]. Wang Weichun Feng Wei submitted an application patent from China, CN103750050, describing a palm oil nanoemulsion that solved the problems of high grease costs, low absorption rates, low oil content in the existing prepared palm oil nanoemulsions, large granularity, poor stability, long production periods, high equipment investments, and high production costs in existing young animal feeds. The palm oil nanoemulsion was prepared by mixing an emulsifier with palm oil, cutting and emulsifying the mixture, and ultrasonically performing cell breaking in the mixture. The process was simple, the entire reaction process was easily controlled, the entire process production period was short, the equipment investment and production costs were low, the oil content of the produced nanoemulsion was high, the distribution granularity was small, the stability was good, and the digestion by livestock increased [98].
There is a growing trend of oil nanoencapsulation patent applications, indicating that many innovations have been made and attesting to the technology the global market.
Conclusion
Nanoencapsulation is well-established for oil preservation. It offers a plethora of advantages, including the effective protection of the encapsulated oil against degradation, the possibility of accurate control of the oil release, easy administration, and avoidance of the evaporation of the volatile components. Moreover, nanoencapsulation may be achieved by a variety of techniques. Technique selection will depend on the physicochemical characteristics of the active compounds, the processing conditions, particle size and density necessary to incorporate the oil properly into the final product, the mechanism of release, and the cost constraints. Although there currently are not many oil nanoencapsulation products in the food market, there is no doubt that if boosted by recent remarkable scientific advances, new approaches in oil nanoencapsulation will soon be considered in the application of oils in food additives and nutritional supplements, and patents application will continue to increase.
缩写
- CG:
-
Cashew gum
- CO2 :
-
Carbon dioxide
- DHA:
-
Docosahexaenoic acid
- DLS:
-
动态光散射
- EOs:
-
Essential oils
- EPA:
-
Eicosapentaenoic acid
- ESO:
-
Eucalyptus staigeriana essential oil
- FA:
-
Ferulic acid
- FDA:
-
Food and Drug Administration
- FTIR:
-
傅里叶变换红外光谱
- HOPO:
-
High-oleic palm oil
- LD:
-
Laser diffraction
- n-3:
-
Omega-3 fatty acids
- n-6:
-
Omega-6 fatty acids
- PCL:
-
Poly(ε-caprolactone)
- PDI:
-
Polydispersity index
- PUFA:
-
Polyunsaturated fatty acids
- SFEE:
-
Supercritical fluid extraction of emulsions
- SLN:
-
Solid lipid nanoparticles
- Toc:
-
Tocopherol
- US EPA:
-
United States Environmental Protection Agency
- 紫外线:
-
Ultraviolet radiation
纳米材料