生物传感器和纳米传感器在农业生态系统中的应用综述
摘要
过去几十年见证了许多挑战,迫切需要确保全球粮食安全。增加粮食生产的过程使农业生态系统面临许多挑战,例如不同农药残留颗粒的持续存在、重金属的增加以及对农业环境产生负面影响的有毒元素颗粒的污染。这些有毒元素通过农产品进入人体会导致许多健康影响,例如神经和骨髓疾病、代谢紊乱、不孕症、细胞水平生物功能的破坏以及呼吸系统和免疫系统疾病。考虑到报告的每年 220,000 人因残留农药颗粒的毒性作用而死亡,可以理解监测农业生态系统的紧迫性。目前用于监测农业生态系统的做法依赖于气相色谱、高效液相色谱、质谱等技术。 它们有多种限制,昂贵,繁琐的协议,需要复杂的设备和熟练的人员。过去几十年见证了纳米技术科学的巨大发展,这种发展在很大程度上促进了适度、快速和经济可行的生物和纳米传感器的发展,用于检测污染自然农业生态系统的不同实体,其优点是对人类无害健康。纳米技术的发展促进了生物和纳米传感器的快速发展,可用于检测多种复合材料,从多种金属离子、蛋白质、农药到完整微生物的检测。因此,本综述重点关注用于监测农业生态系统的不同生物和纳米传感器,并试图强调从概念验证到商业化阶段影响其实施的因素。
介绍
过去几十年来,人口长期紧张、气候条件不断波动、资源抽奖增加等诸多挑战,都构成了巨大的威胁,迫切需要保障全球粮食安全。现有的满足食品需求的农业做法包括不加控制地使用资源、先进的机械以及增加和不加区别地使用农用化学品。这些做法导致土壤、空气和水资源的严重恶化,从而明显提高了农业环境的污染水平,进而严重影响了人类/动物的健康。根据全球每年有 2600 万人成为农药中毒受害者的信息,可以估计农药使用对健康的影响程度,每年约有 220,000 人死亡 [1]。此外,由于其持久性,农药残留在环境中停留的时间较长,从而污染土壤,从而引起人们对土壤功能、生物多样性和食品安全的担忧[2]。此外,已有许多关于农药残留进入食物链,然后在消费者体内积累,进一步导致严重健康问题的报告。已知这些杀虫剂本质上具有细胞毒性和致癌性 [3,4,5,6]。它们还可诱发各种神经和骨髓疾病、不孕症以及呼吸系统和免疫系统疾病 [7,8,9,10]。因此,环境中农药残留的监测成为当务之急。此外,定期监测此类残留农药还将提供有关其发生是否在可接受范围内或超出可接受范围的信息[11]。
农业生态系统面临的另一个重要挑战是包括镉、汞、铜、锌、镍、铅和铬在内的致命重金属的持久性,因为它们通过破坏生物活动对各种生物系统造成长期和重大损害。细胞水平 [12, 13],例如,通过破坏光合作用、破坏矿物质吸收、破坏电子传递链、诱导脂质过氧化、干扰必需元素代谢、诱导氧化应激和破坏植物器官,如根、叶和其他细胞成分 [14,15,16]。当然,它们在地壳中的自然存在是不可否认的事实,但不受控制的人类活动已在显着程度上扰乱了这些元素的地球化学循环和生化平衡。这导致这些金属在不同植物部位的流行率增加。总之,各种生态系统中重金属的存在和流行所带来的所有风险都强调需要开发即使在环境样本中的低浓度下也能感知它们的系统[17]。
目前,可用于监测农业生态系统的各种方法包括气相色谱、高效液相色谱、质谱等(图 1)。所有这些技术都可以轻松检测和量化环境和农业样品中的污染物。相反,此类测量的灵敏度、特异性和可重复性是无可争议的,但这些方法的部署主要受到时间消耗、成本高以及需要复杂设备和熟练人员的限制 [8]。因此,迫切需要适度、快速且经济可行的方法来监测此类农业污染物 [18,19,20]。纳米传感器是纳米级元件装置,旨在识别特定分子、生物成分或环境情况。这些传感器具有高度特异性、方便性、成本效益,并且与它们的宏观类似物相比,检测水平要低得多。一个典型的纳米传感器设备操作包含三个基本组成部分:
- 1.
样品制备:它可以是气态、液态或固态的均质或复合悬浮液。由于杂质和干扰,农业生态系统的样品制备非常具有挑战性。样品包含传感器可以瞄准的特定分子、分子或生物体的功能组。这些被称为分析物的目标分子/有机体可以是分子(染料/颜色、毒物、杀虫剂、激素、抗生素、维生素等)、生物分子(酶、DNA/RNA、过敏原等)、离子(金属、卤素、表面活性剂等)、气体/蒸气(氧气、二氧化碳、挥发性化合物、水蒸气等)、生物体(细菌、真菌、病毒)和环境(湿度、温度、光照、pH 值、天气等)。 )
- 2.
识别:某些分子/元素识别样品中的分析物。这些识别分子是抗体、适体、化学传奇酶等,对其分析物具有高亲和力、特异性、选择性,可将其量化到可接受水平。
- 3.
信号转导:某些信号转导方法将这些普通设备分为不同类型,例如光学、电化学、压电、热释电、电子和重力生物传感器。它们将识别事件转换为可计算的信号,这些信号经过进一步处理以生成数据(图 2)。
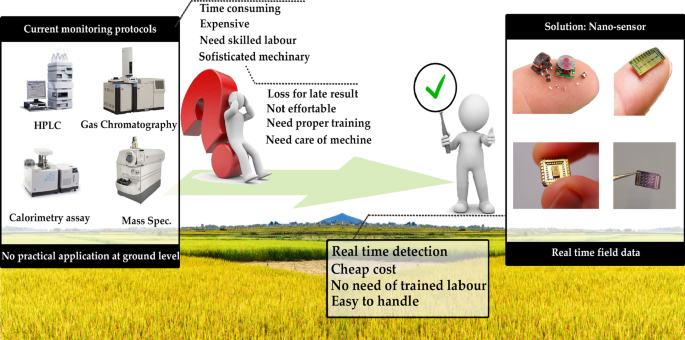
突出传统与先进监测技术差异的示意图
图> <图片>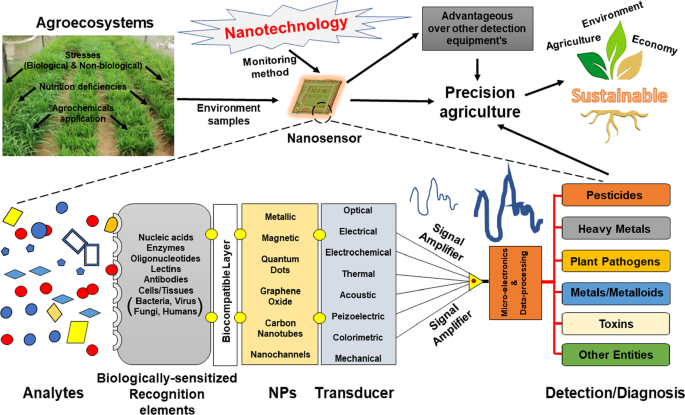
用于监测农业生态系统的纳米传感器组件的简化表示
图>纳米技术干预将刺激定位于改变诊断的不同领域,如健康、药物、食品、环境以及农业部门,从而将投机特征转化为实际输出 [21,22,23,24, 25,26,27,28]。纳米技术通过为人类提供现代工具,包括建立在生物技术上的传感器、基于纳米的医疗设施,以及简化杀虫剂、药物残留、食品检测的生物光子学,在众多诊断方法的进步中发挥着重要作用。传播的病原微生物、毒素污染物和重金属离子 [24, 29]。幸运的是,纳米技术领域包括对原子或分子尺度的控制材料的理解,其中当等同于类似物质的块状形式时,物质展现出独特的属性和性能[30]。目前,在所有方法中,生物传感器是一种适度紧凑的研究装置,它能够通过采用生物起源的识别组件以定量方式或半定量方式产生确定的系统数据,该识别组件与信号转换单元 [31,32,33]。信号转导方法的应用类型已将这些普通设备分为不同类型,例如光学、电化学、压电、热释电、电子和重力生物传感器 [34]。纳米技术的最新进展为设计生物传感器开辟了各种新途径 [29, 35]。纳米材料与不同生物传感平台(纳米生物传感器)的杂交提供了大量的联合和多用途方法,以提高检测的灵敏度 [36],从而提高对单个分子的监测能力 [32, 37] , 38]。纳米尺度被定义为大约 1-100 纳米,也相当于十亿分之一米。通过将其与直径约为 1000 nm 的平均细菌细胞的尺寸进行比较,可以很容易地理解它[39]。用于传感的纳米材料被称为纳米传感器,它是在原子尺度上构建的,用于数据收集。纳米材料被进一步重新分配为可用于多种应用分析的信息,例如,密切关注难以接近的区域的各种物理和化学迹象,检测各种细胞器中生物来源的不同化学物质,并确定纳米级粒子在环境和工业中 [40, 41]。使用纳米传感器甚至可以检测到单个病毒颗粒和浓度非常低的物质的存在。纳米传感器由生物敏感层组成,该生物敏感层与另一个称为换能器的元件共价连接。由于目标分析物与生物受体相互作用而产生的生理化学变化转化为电信号[40]。
近年来,大量卓越的视觉识别生物和纳米传感器已被用于从大量样品中检测几种复合材料。复合材料的范围涵盖了几种金属离子、蛋白质、农药、抗生素,以检测完整的微生物,以及核酸扩增和测序 [19, 33, 42, 43]。除了监测农业控制过程和残留物外,纳米技术的其他潜在应用也在过去二十年中浮出水面 [44,45,46,47]。将纳米技术用于改善农业部门的必要好处包括纳米材料辅助提供生长促进剂 [44, 48, 49]、营养(尤其是微量营养素)[49, 50] 以及植物基因改造 [51, 52] ]。此外,还发现使用纳米杀真菌剂、纳米杀菌剂和纳米杀虫剂形式的各种杀虫剂 [50, 53,54,55]。此外,纳米技术的其他好处包括基于纳米材料的修复 [56]、纳米除草剂 [57] 以及用于生物加工 [58]、水产养殖 [59]、收获后技术 [60]、兽医护理 [61]、渔业 [ 62] 和种子技术 [63]。所有这些应用共同显示出各种优势,如减少污染(主要是土壤和水)、降低环保相关成本以及提高养分利用效率 [45, 46, 50, 56, 64,65,66,67,68](图 3)。鉴于上述事实,本综述的目标是在不同的农业生态系统中使用不同种类的纳米传感器来揭示不同的成分,并检测一些侵入自然农业生态系统的外来成分。
<图片>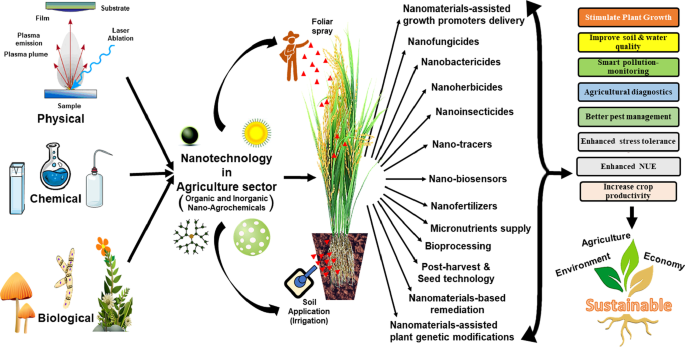
纳米技术在农业领域的各种应用
图>用于农药检测的纳米传感器
农药在农业系统中得到广泛应用,可以避免、调节或消灭害虫、昆虫、杂草和真菌,以提高农业生态系统的生产力 [69]。农药的使用在不断增加,它们可能会确保全球农产品的近三分之一份额 [70]。然而,田间乱用农药污染了地下水,标志着其在食物资源中的积累,从而也严重影响了人类和动物等非目标物种(图4)。人类接触杀虫剂会以多种方式影响健康,随之产生的健康影响范围可以从致突变性、神经毒性、致癌性到遗传毒性 [71, 72]。一些农药(如有机磷)即使在小浓度下使用也会在动物体内积聚,并且暴露于较高浓度会导致乙酰胆碱酯酶等酶受到抑制,这些酶会给人类带来严重的健康风险 [73]。因此,开发优良的农药残留检测方法对于保障食品安全具有重要意义。
<图片>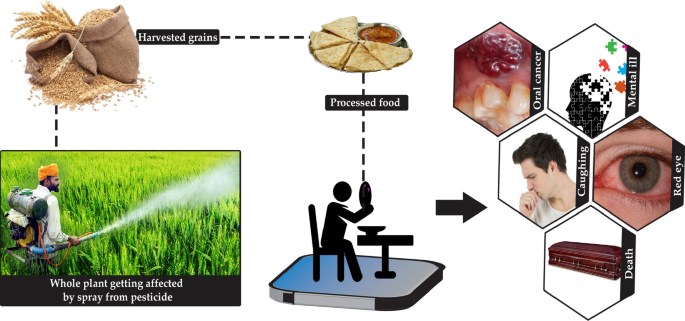
农药对人体健康的不良影响
图>尽管很长一段时间以来,各种方法都被用于检测农药残留,例如高效液相色谱、比色测定、酶联免疫吸附剂测定、液相/气相色谱-质谱、电泳和荧光测定程序 [8] , 74,75,76,77,78,79]。然而,这些技术中的大多数是单信号分析,需要昂贵的设备、专业操作员和复杂的样品预处理,而有些技术甚至容易受到环境条件的影响 [80, 81]。因此,此类检测措施不适用于残留农药的现场检测。此外,还发现它们不适合实时检测,这限制了它们在紧急情况下的使用 [82]。因此,采用多信号的检测方法提高了分析的可靠性和便利性。例如,针对多信号荧光法与比色法相结合的方法能够规避多面结构中背景的影响,并在不同的实际请求中补充裸眼传感[83]。因此,集中更多精力评估以快速、简单、选择性、精细、精确和易于理解的方式检测农药的不同方法,导致了用于检测农药残留的光学传感器的发展[80]。
许多光学策略已经被认可用于农药检测,这些策略利用识别元素,如酶、抗体、分子印迹聚合物、适体和主客体识别器。这种方法可以坚定地识别和检测特定的农药颗粒 [81, 84,85,86,87,88]。此外,识别组件与纳米材料的耦合导致更高水平的灵敏度和极大的瞬时部署特异性,这是快速有效的农药检测的主要要求 [82]。因此,为了寻求一种快速、灵敏、特异、精确且易于操作的残留农药检测方法,纳米传感器因其成本效益、紧凑、易于运输、非凡的灵敏度和更短的检测时间 [89](图 1)。
一般而言,光学传感器由识别元件组成,该元件对特定残留农药颗粒具有特异性,并且可以与其他成分(传感器)联网,传感器用于产生特定农药残留物与传感器结合的信号.由酶、抗体、分子印迹聚合物、适体和主客体识别器组成的识别组件正在引起科学界的考虑,以提高任何传感器的诊断性能。基于信号输出格式,流行的根深蒂固的光学探头可以分为四种类型。这些是荧光 (FL)、比色 (CL)、表面增强拉曼散射 (SERS) 和表面等离子体共振 (SPR) 光学传感器 [90]。
另一种广为人知的纳米传感器是免疫层析条 (ICTS) 纳米传感器,在即时分析设备中得到广泛认可 [91]。由于其即时检测行为,免疫色谱分析也被报道参与监测农业生态系统。例如,在报道的用于检测转基因作物的免疫层析分析中采用了可见的比色读出策略,该策略仅提供是/否响应,并且通常灵敏度不足[92,93,94]。同样,据报道,基于金纳米颗粒的 ICTS 传感器检测灵敏度较低,因为产生的颜色密度相对较弱,这限制了它们的应用 [95, 96]。然而,它们的灵敏度可以通过几种提议的放大策略来提高,例如增强检测信号强度、增强试剂的亲和力、优化标记技术和修改条带装置的形状 [96]。因此,改进的ICTS纳米传感器也可以证明是一种经济可行的农业生态系统农药残留检测工具。
纳米技术与不同电化学方法的结合使传感器具有优越的操作表面积以及对电极微环境的良好检查。纳米粒子具有发散性和众多的特性,因此具有在基于电化学现象的传感结构中发挥多种用途的潜力,例如,催化电化学反应、增强电子转移、标记和作为反应物 [97]。因此,电化学纳米传感器似乎是一种有效的农药检测工具。最近,主要基于胆碱酯酶的电化学生物传感器作为一种有利的装置出现,用于检测残留的杀虫剂颗粒,尤其是属于氨基甲酸酯类和有机磷酸酯类的农药颗粒,这归因于它们的敏锐性、选择性和无痛的创造方法 [98, 99]。然而,基于酶的生物传感器受到很多限制,包括价格高、酶活性降低和重现性被截断 [100]。此外,酶似乎本质上是不稳定的,并且在恶劣的环境条件下也会发生变性,这限制了生物传感器的寿命,从而限制了它们的实际应用 [101]。此外,一些杂质的表现,例如生物来源样品中不同重金属的出现,也会干扰检测过程中酶的选择性和灵敏度,从而可能产生假阳性结果[102]。因此,它引发了对非酶电化学生物传感器的需求。纳米材料似乎是配制非酶电化学传感器的有前途的参赛者 [103]。广泛发现包括纳米粒子(例如,CuO、CuO-TiO2 和 ZrO2、NiO)、纳米复合材料(例如钼纳米复合材料)和纳米管(例如肽和碳纳米管)在内的各种纳米材料都参与电化学测定残留量。杀虫剂颗粒 [104,105,106]。此类纳米材料对残留农药颗粒的明确而深入的研究归因于它们的极小尺寸、更大的表面积以及无与伦比的电学和化学性质[70]。
各种研究报告了各种纳米传感器对特定农药的灵敏度和选择性(表 1),例如,发现基于银纳米枝晶和上转换纳米粒子的两种不同光学传感器可检测农药乐果和赛克津0.002 ppm 和 6.8 × 10 −8 M,分别为 [107, 108]。类似地,使用 3D 石墨烯纳米复合材料装饰的 CuO 纳米颗粒接地的电化学纳米传感器检测到 0.01 nM 水平的马拉硫磷 [109],而通过壳聚糖 - 氧化铁纳米复合材料制造的电化学适体传感器以 0.001 ng/mL 的惊人灵敏度检测到马拉硫磷 [110] .
图>用于检测重金属的纳米传感器
存在多种重金属离子,如 Pb 2+ , 汞 2+ , 银 + , Cd 2+ , 和 Cu 2+ 不同资源对人类及其周围环境产生不稳定的影响。农业和工业成就的不断提升以及废水和家庭排放中重金属离子的排放不足,支持了重金属在不同环境中的积累[111]。因此,为保证环境安全和健康分析,迫切需要通过熟练的操作来筛选出微量重金属离子。对重金属的理解可以通过探索多种分析系统来实现 [112],例如 X 射线荧光光谱法 (XRF)、原子吸收光谱法 (AAS)、原子发射光谱法 (AES) 和电感耦合等离子体质谱法。 ICP-MS),但它们的应用受到很多限制,例如设备过于繁琐、方法耗时和劳动强度大。因此,为了指导这些限制,已经全面审查了多种类型的光学、电化学和比色策略(表 2),以设计适度且有利可图的平台,用于对重金属离子进行细致、仓促和有洞察力的探索 [113, 114]。
图>经常以重金属检测为目标的光学化学传感器适合一组化学传感器,这些化学传感器主要采用电磁辐射在称为转导元件的元件中产生诊断信号。样品和辐射之间的相互作用改变了特定的光学考虑因素,该考虑因素可能与分析物的浓度相关 [115, 116]。例如,使用纳米混合 CdSe 量子点合成的用于检测镉的光学纳米传感器在镉金属的感觉上恢复了其绿色光致发光 [117]。光学化学传感器的工作原理是光学属性(发射、吸收、传输、寿命等)似乎发生变化,这是由于捕获的指示剂(有机染料)与分析物结合而出现的 [118]。吸引人的基于石墨烯的纳米技术的方法开始作为一种可归因的工具,它使这些挑战无能为力,并使传感平台具有增强的性能。近来,主要基于石墨烯纳米材料的光学技术作为检测重金属离子的激动人心的实践之一得到了发展,因为它们可能具有温和的结构和对某些独特金属离子的感知价值 [116]。
Ag、Au、Pd 等贵重纳米粒子具有模拟过氧化物酶活性的独特特性,它们与石墨烯的聚集增强了它们的坚固性和卓越的催化性能。有各种各样的传感器与基于此功能的大量重金属离子的检测有关。氧化石墨烯与银纳米颗粒的杂交产生了模拟过氧化物酶活性的纳米杂交体,并且进一步发现它们能够区分双链和单链 DNA 分子。因此,对Pb 2+ 进行量热检测 和汞 2+ 适合基于金属离子引发的 DNA 构象变化,因为构象在其发生时被改变为四链体排列或发夹状组装 [119, 120]。此外,这种比色方法具有操作简单、经济可行、仪器可移动和易于使用的优点。发现用于检测重金属的化学传感器在消除目标物种方面存在麻烦,因为它们会导致二次污染。因此,将荧光和磁性功能整合在一个单一的纳米复合颗粒中似乎是一种有效的替代品 [121]。然而,磁性纳米粒子的表现强烈地淬灭了荧光部分的光致发光,因此对此类纳米复合材料的发展提出了严峻的挑战。因此,为了避免这种担忧,在分子水平上发生的许多相互作用,例如疏水和静电相互作用、氢键和共价键,通常是纳米复合材料合成的目标。例如,利用硫醇化学方法将量子点置于聚合物层状Fe2O3球体的浅层上。捕获在Fe2O3纳米颗粒等多种材料表面的金纳米颗粒和采用静电连接的二氧化硅微球也已被合成[122, 123]。
利用纳米化学原理合成多模态纳米传感器的方法更具吸引力,因为它不仅可以有效地检测而且可以去除水介质中的重金属离子。 Satapathi 等人合成的多模态纳米传感器。 [124] 通过多步生产实践,需要一个薄的二氧化硅外壳,其中包含磁性 (Fe2O3) 纳米粒子、一个不可移动的间隔臂和一个荧光量子点,用于同时识别和消除点状汞离子。这种纳米传感器的卓越灵敏度可以通过其检测 Hg 2+ 的能力来标记 在纳摩尔水平,检测限仅为 1 nm。纳米传感器的环保方面可以通过使用外部条形磁铁去除检测到的分析物的独特属性来提倡,从而不会留下残留物作为污染物。几种化合物用于稳定纳米传感器,例如多糖柠檬酸盐、不同的聚合物和蛋白质,以改善纳米传感器的属性 [125]。表儿茶素稳定化的银纳米粒子可用于Pb 2+ 的鉴别检测 ,那也是,在发生不同的金属离子窥探。检测下限、易于合成、令人钦佩的辨别力和经济的生产,使 ECAgNPs 成为一种有效的传感器,用于重复检测 Pb 2+ 生态模型中的强度 [126]。量子点的使用在其光物理和化学属性方面提供了显着的优势,从而使基于荧光量子点的传感器成为检测众多金属离子的有效工具 [127, 128]。然而,使用量子点的主要缺点是它们在实际应用中的分离和恢复,这恰好是一项无节制、费力和乏味的任务。然而,将磁性纳米材料 (Fe3O4) 引入基于量子点的荧光传感器解决了这个问题,并由于其高比表面积、特殊的磁性、磁性可操作性和低毒性提供了一些额外的优势。杨等人。 [128] 建立了基于羧甲基壳聚糖的多功能磁性荧光纳米粒子,结合荧光量子点和磁性纳米材料,可以检测和分离Hg 2+ 同时具有 9.1 × 10 −8 的感应电平 摩尔/升。因此,纳米技术的朴实而复杂的方法为未来基于场的重金属传感装置提供了方向,而这现在看来是一项艰巨的任务,同时也存在各种局限性。
Nanosensors for Detecting Plant Pathogens
The ascertainment, recognition, and assessment of pathogens are vital for scientific elucidation, ecological surveillance, and governing food security. It is imperative for investigative outfits that the delicate element of biological origin, which is a constituent of biological provenance or biomimetic constituent, interacts with the analyte in the examination. There are numerous profound, trustworthy, and swift recognition components, for instance, lectin, phage, aptamers, antibody, bacterial imprint, or cell receptor, which have been described for exposure of bacteria [129]. The most widely used biosensing components for analyzing pathogens are bacterial receptors, antibodies, and lectins. These constituents find wide applications as biosensing components to scrutinize pathogens owing to their adaptability of amalgamation into biosensors [130, 131]. Aptamers, the nucleic acids having only a single strand, are economically feasible and chemically steady, as compared to the recognition elements which are based on the antibodies for detecting bacteria [132]. However, they also pose various disadvantages like batch-to-batch variations, sturdiness in complex materials and they are also comparatively complex to prepare. The approach pointing to ‘chemical nose’ is a recently established equipment for detecting pathogens. It appoints multifarious discriminatory receptors that generate a unique response configuration for every objective, thus permitting their ordering. It functions in a fashion analogous to the working of our intellect of smelling something [133]. This technique involves the training of sensors with competent bacterial samples to establish a reference database. The identification of bacterial pathogens is done by equating them with the reference catalog [134]. Usually, nanoparticle-centered “chemical nose” biosensors necessitate the amendment of the surface of the nanoparticle with several ligands where an individual ligand is liable for a distinctive communication with the objective [133]. The variance in the size, as well as the external make-up of the nanoparticles, is selected in a way that every single set of particles can retort to different classes of bacteria in an inimitable way thereby offers supplementary features to the absorption spectrum.
The addition of nanoparticles to the bacteria leads to the development of aggregates encompassing the bacteria as a result of electrostatic interfaces amid the anionic sections of the bacterial cell walls and cationic cetyltrimethylammonium bromide (CTBr). This process of aggregation promotes a change of color induced by a swing in localized surface plasmon resonance. The color variation is further denoted by procuring an absorption spectrum in the existence of several bacteria [135, 136]. The components of the bacterial cell wall which are responsible for this kind of aggregation are teichoic acids in Gram-positive and lipopolysaccharides and phospholipids in Gram-negative bacteria [137]. These aggregation patterns are unique and are motivated by the occurrence of extracellular polymeric substances on the bacterial surface. These varying aggregation patterns are accountable for offering discernable colorimetric responses. Therefore the “chemical nose” established on nanoparticles could be accomplished to sense blends of varying bacterial species. During infections the “chemical nose” is potent enough to differentiate amid polymicrobial and monomicrobial cases, which facilitates superior effectiveness along with prompting antimicrobial therapy, precluding the requirement of extensive and prolonged testing of the sample [133]. The multichannel nanosensors are highly sensitive and can detect bacterial species even strains present in biofilms within minutes.李等人。 [138] established a multichannel sensor based on gold nanoparticles (AuNPs) and used it to spot and recognize biofilms based on their physicochemical attributes. The sensitivity of the nanosensor can be well advocated by its ability to discriminate amongst six biofilms. Another sensor which was designed based on hydrophobically employed gold nanoparticles by Phillips et al. [139] rapidly recognized three different strains of E. coli . The conjugated polymers bearing negative charge in the sensor systems were eventually replaced by the pathogenic cells which differentially restored the polymer fluorescence.
Nanotechnology offers novel prospects for redefining the constraints of human discernment. In the course of evolution, the olfactory system of human beings has got the unique ability to detect volatile organic compounds present at tremendously low concentrations in different complex environments [140]. The great sensitivity and flexibility of human beings to differentiate more than a trillion olfactory stimuli marks olfaction as an encouraging dais for different biotechnological applications [141, 142]. Various effective sensors that primarily function based on olfaction have been proposed for unveiling bacteria. The system of such nanosensors is mainly encompassed of three different constituents:1) surface-functionalized nanoparticles, 2) pro-smell fragments, and 3) enzymes that slice the pro-fragrances for generating the olfactory output. The fine-tuning of these three components offer a delicate sensory system, which allows the rapid detection of bacteria at levels as low as 10 2 CFU/ML [143]. The introduction of magnetic nanoparticles also enables the separation, purification, and recognition of pathogens under complex environments. The nanomaterial-grounded, ‘enzyme nose’ nanosensor is also a convenient investigative method meant for detecting toxicologically significant targets present in natural samples. Sun et al. [134]designed a unique enzyme nanosensor, which was grounded on the non-covalent centers, for detecting pathogens. The employment of magnetic nanoparticles–urease sensors permitted the profound recognition of bacteria with a precision of 90.7% at the concentration of 10 2 CFU/LL in a very small time of 30 min. Similarly, various other different types of optical, electrochemical, and immunosensors have also been developed for detecting diverse plant pathogenic microorganisms (Table 3). For instance, the optic particle plasmon resonance immunosensor synthesized using gold nanorods effectively detected Cymbidium mosaic virus (CymMV) or Odontoglossum ringspot virus at the concentrations of 48 and 42 pg/mL (Lin et al. 2014) whereas the Fe3O4/SiO2 based immunosensor revealed the presence of Tomato ringspot virus , Bean pod mottle virus and Arabis mosaic virus at the concentrations of 10 −4 mg/mL [144]. Therefore, directing the performance of approachable nanomaterials at the molecular scale can be exploited to revise the annotations of humans regarding their environments in a fashion that seems otherwise unmanageable.
图>Nanosensors for Detection of Other Entities
Amino acids are very crucial molecules required by the living systems as they play a pivotal role of building blocks in the process of protein synthesis [145], vital character for maintenance of redox environments in the cell and extenuating destruction from the toxin and free radicals [146]. The investigative methods for detecting amino acids have been reported, especially by chromatography, chemiluminescence, and electrochemistry [147]. However, the application of existing technologies is greatly restricted by the great expenses and time-consuming steps. Currently, nanomolecular sensors have been established for detecting such molecules owing to their chemical steadiness, bio-compatibility, and easy surface alteration [148, 149]. The employment of gold nanoparticles for biosensing solicitations has been reported in different biological environments. The amine side chain and sulfhydryl (thiol) group of amino acids may perhaps covalently bind with the gold nanoparticles, thereby inducing an accretion of these nanostructures which further results in a color alteration from red to blue on the aggregation of amino thiol molecules [150, 151]. Chaicham et al. [147] developed an optical nanosensor grounded on gold nanoparticles that could detect Cys and Lys at concentrations of 5.88 μM and 16.14 μM, respectively, along with an adequate percentage retrieval of 101–106 in actual samples.
Similarly, other metal ions that are required by living organisms for performing various metabolic functions can be detected by employing different nanosensors. A dual-emission fluorescent probe was developed by Lu et al. [152] for detecting Cu 2+ ions by condensing hydrophobic carbon dots in micelles molded by the auto-assemblage of different amphiphilic polymers. A vigorous, self-accelerating, and magnetic electrochemiluminescence nanosensor which was established on the multi-functionalized CoFe2O4 MNPs was established for the foremost and later employed for the extremely sensitive as well as discriminating recognition of the target Cu 2+ through click reaction in a quasi-homogeneous system [82]. Gold nanorods are also exploited for sensing Fe (III) ions. Thatai et al. [17] devised highly sensitive gold nanorods using cetyltrimethylammonium bromide as illustrative material for detecting ferric ions along with a surprising sensing level equivalent to 100 ppb. Zinc is another important element, and it occurs in a divalent cationic form as Zn 2+ ions. Zn 2+ ion has the capability of sustaining important activities counting synthesis of DNA and protein, RNA transcription, cell apoptosis, and metalloenzyme regulation [153, 154]. Usually, fluorescent probes are exploited for detecting the Zn 2+ ions in biological systems. The pyridoxal-5′-phosphate (PLP) conjugated lysozyme cocooned gold nanoclusters (Lyso-AuNCs) can also be exploited for the selective and turn-on detection of divalent Zn 2+ ions in the liquid environment. The yellow fluorescence of PLP Lyso-AuNCs displays noteworthy augmentation at 475 nm in the occurrence of Zn 2+ generating bluish-green fluorescence which is accredited to the complexation-induced accretion of nanoclusters. The developed nanoprobe can detect Zn 2+ ions in nanomolar concentrations (39.2 nM) [154]. The dual-emission carbon dots (DCDs) synthesized by Wang et al. [155] can also be exploited for revealing Zn 2+ ions as well as iron ions (Fe 3+ ) in different pH environments. The ferric ions could also be detected in an acidic environment along with an amazing sensation level equaling 0.8 µmol/L while Zn 2+ ions could be detected in an alkaline environment along with a detection limit of 1.2 µmol/L.
These days groundwater is used for irrigation and it is also the solitary seedbed of potable water in numerous regions, exclusively in the isolated agronomic sections. The capricious expulsion of numerous contaminants into the environment has expressively deteriorated the eminence of groundwater, thus has significantly threatened environmental safety [156, 157]. Although there are numerous micropollutants, however, the rushing of fluoride in groundwater has stretched out accumulative civic consideration as a result of the grave fluorosis, severe abdominal and renal complications persuaded by the elevated intake of fluoride ion [158]. So, there is a quest to diagnose and unveil hardness as well as the presence of fluoride ions in the ground-water which has expected substantial considerations owing to their significant parts in the different ecological, biological, and chemical processes [157]. Although fluorescent probes which are considered as traditional methods, can be exploited for detecting F − , however, the employment of quantum dots, an inorganic nanomaterial, can grab extensive considerations on account of their distinctive optical possessions comprising size-oriented fluorescence, tapered and coherent emission peak with a wide exciting wavelength, and outstanding photo solidity [159, 160]. The creation of a fluorescence resonance energy transmission channel from the carbon dots and the gold nanoparticles appears to be a competent solution for detecting numerous analytes. Therefore, constructing a novel nanosensor via gold nanoparticles and carbon dots for detecting F − seems to be a proficient strategy. The hybrid nanosensor assorted with calcium ions has been reported to spot fluoride ions along with a subordinate recognition level parallel to 0.339 ppm [103]. Lu et al. [161] also developed another novel strategy for detecting fluoride, which was grounded on dual ligands coated with perovskite quantum dots, and the recognition level was found to be 3.2 μM.
The agricultural systems also necessitate the diagnosis of various other entities for the smooth functioning and enhanced productivity of the agroecosystems. The detection of other miscellaneous entities has also been facilitated by the employment of nanosensors (Table 3), for instance, the detection of transgenic plants, the presence of aflatoxins, and even the occurrence of wounds in plants. The SPR nanosensor developed using gold nanoparticles detected the Aflatoxin B1 at the concentration of 1.04 pg mL −1 [162] whereas the SERS-barcoded nanosensor fabricated using the encapsulation of gold nanoparticles with silica followed by the conjugation of oligonucleotide strands effectively detected the presence of Bacillus thuringiensis (Bt) gene-encoded insecticidal proteins in rice plants at 0.1 pg/mL, thereby, clearly advocating the transgenic nature of rice plants [163].
Nanosensors for Detection of Nanoparticles
Nanomaterials can also occur naturally, such as humic acids and clay minerals; extensive human activities can also lead to the incidental synthesis of various nanomaterials in the environment, for instance, diesel oil emanations or by the discharge of welding fumes; or they can also be explicitly concocted to unveil matchless electrical, optical, chemical or physical features [164]. These characteristics are exploited in plenty of consumable merchandise, for instance, medicines, food, cosmetics and suntan lotions, paints, and electronics, as well as processes that directly discharge nanomaterials into the surroundings, such as remediating contaminated environs [165, 166]. Furthermore, the rapid employment of metal nanoparticles in various systems has raised many concerns due to the potential environmental risks posed by them as they are unavoidably lost in the environment throughout the processes meant for their fabrication, conveyance, usage, and dumping [167]. Carbon-based nanomaterials are quite established against degradation and as a result, amass in the surroundings [168]. Nanoparticles, attributable to their greater surface area, find it much easier to bind and adsorb on the cellular surfaces. They harm the cell in several ways, such as, by hindering the protein transport pathway on the membrane, by destroying the permeability of the cell membrane, or by further inhibiting core components of the cell [169]. Currently, an overwhelming figure of the engineered nanoparticles engaged for different ecological and industrial solicitations or molded as by-products of different human deeds are ultimately discharged into soil systems. The usual nanoparticles employed comprise the metal engineered nanoparticles (elemental Fe, Au, Ag, etc.), metal oxides (SiO2, ZnO, FeO2, TiO2, CuO, Al2O3, etc.), composite compounds (Co–Zn–Fe oxide), fullerenes (grouping Buckminster fullerenes, nanocones, carbon nanotubes, etc.), quantum dots frequently encrusted with a polymer and other organic polymers (Dinesh et al. 2012). Different plant growth-promoting rhizobacteria (PGPR) like Bacillus subtilis , Pseudomonas aeruginosa , P. fluorescens, and P. putida , and different bacteria involved in soil nitrogen transformations are inhibited to varying degrees on exposure to nanoparticles in aqueous suspensions or pure culture conditions [170]. The nanoparticles grounded on metals copper and iron are alleged to interact with the peroxides existing in the environs thereby engender free radicals that are notorious for their high toxicity to microbes [171]. Therefore, there is a strong need to monitor the different nanoparticles which find an ultimate sink in the soils especially of agroecosystems.
Various techniques can be reconnoitered for sensing nanoparticles, one among them is the usage of microcavity sensors, which, in the form of whispering gallery resonators have acknowledged extensive consideration. Here, the particle binding on the exterior of the microcavity disturbs the optical possessions thereby instigating a resonant wavelength swing with magnitude reliant upon the polarizability of the particle. The measure of the change facilitates surveillance of the binding actions in real-time and is also used to evaluate the particle size [172]. Optical sensing empowered with the extreme sensitivity of single nanoscale entities is sturdily anticipated for solicitations in numerous arenas, for instance, in environmental checking, other than in homeland security. Split-mode microcavity Raman lasers are also highly sensitive optical sensors that can perceive the occurrence of even a single nanoparticle. The presence of nanoparticles is revealed by observing the distinct alterations in the beat frequency of the Raman lasers and the sensing level has been reported to be 20 nm radius of the nanoparticles [138].
Nanotechnology Implementation in an Agroecosystem:Proof-of-Concept to Commercialization
There are hundreds of research articles and studies that are being published every year on nanosensor's application in agriculture. However, very few nanosensors have yet been commercialized for the detection of heavy metals, pesticides, plant-pathogen, and other substances in an agroecosystem. Because these academic outputs are not properly converted/conveyed to commercial or other regulatory platforms. Certain scientific and non-scientific factors hinder these nanosensors from proof-of-concept to fully commercialized products. These factors are scale-up and real-use (technical), validation and compliances (regulatory), management priorities and decisions (political), standardization (legal), cost, demand and IPR protection (economic), safety and security (environmental health and safety) along with several ethical issues. It is necessary to support enthusiastic researchers and institutions for research and development to develop such nanosensors for agroecosystem, product validation, intellectual protection, and their social understanding and implementation. If we consider these factors strategically, it will help in nanosensor product betterment and implementation to agroecosystem. The US-based startup Razzberry developed portable chemical nanosensors to trace real-time chemical changes in water, soil, and the environment. Similarly, Italian startup Nasys invented a metal oxides-based nanosensor to detect air pollution. There are some other startups nGageIT and Tracense, implementing nanosensor technologies to detect biological and Hazardous contaminants in agriculture.
Perspectives and Conclusions
Since times immemorial, agriculture is the main source of food, income as well as employment for mankind around the globe. In the present era, due to upsurge of rapid urbanization and climate inconsistency, precision farming has been flocking significant attention worldwide. In agricultural system, this type of farming has the ability to maximize the crop’s productivity and improve soil quality along with the minimization of the agrochemicals input (such as fertilizers, herbicides, pesticides, etc.). Precision farming is possible through focused monitoring of environmental variables along with the application of the directed action. This type of farming system also employs computers, global satellite positioning systems, sensors, and remote sensing strategies. As a result, the monitoring of extremely confined environmental situations becomes easy. This monitoring even assists in defining the growth of crop plants by accurately ascertaining the nature and site of hitches. Eventually, it also employs smart sensors for providing exact data that grant enriched productivity by serving farmers to make recovery choices in a detailed manner. Among all the sensors, smart nanosensors are very sensitive and judiciously employed devices that have started proving to be an essential tool for advocating agricultural sustainability, in future.
It has been noticed that the use of nanosensors and or biosensors can accelerate agricultural productivity. These real-time sensors can physically monitor temperature, soil health, soil moisture content and even senses the soil microbiological/microenvironment and nutrient status of soils. Interestingly, these sensors have also been able to detect residual pesticides, heavy metals, monitor plant pathogens and quantify fertilizers and toxins. These nanosensors facilitate speedy, quick, reliable, and prior information that even aid in predicting as well as mitigating the crop losses in the agroecosystems. In addition, the use of nanotechnology-based biosensors also assists in accomplishing the concept of sustainable agriculture. It has been observed that the projection of nanosensors and or biosensors as plant diagnostic tools requires improvements regarding their sensitivity and specificity. Additionally, there is a need for quick, reliable, cheap, multiplexed screening to detect a wide range of plant-based bioproducts. Moreover, the development of broad-spectrum nanosensors that can detect multiple entities will also boost in mobilizing technology. It has been suggested that the biosensor efficiency can be improved further by developing super “novel nanomaterials” that will be available in near future. Perhaps in the coming years, the convergence among nanotechnology, agriculture sciences, rhizosphere engineering, and overall plant engineering will lead to the path towards accomplishment of all Sustainable Development Goals 2030 without incurring any fitness cost on mankind safety, economy, natural resources, and environment.
数据和材料的可用性
不适用。
缩写
- AAS:
-
Atomic absorption spectrometry
- AES:
-
Atomic emission spectrometry
- Ag:
-
Silver
- Al2O3 :
-
Aluminum oxide
- Au:
-
Gold
- CdSe:
-
Cadmium selenide
- CL:
-
Colorimetric
- CoFe2O4 :
-
Cobalt iron oxide
- CTBr:
-
Cationic cetyltrimethylammonium bromide
- CuO:
-
Cupric oxide
- DCDs:
-
Sual-emission carbon dots
- FeO2 :
-
Iron dioxide
- FL:
-
Fluorescence
- ICP-MS:
-
Inductively coupled plasma mass spectrometry
- ICTS:
-
Immunochromatographic strip
- NiO:
-
Nickel oxide
- Pd:
-
Palladium
- PGPR:
-
Plant growth-promoting rhizobacteria
- SERS:
-
Surface-enhanced Raman scattering
- SiO2 :
-
Silicon dioxide
- SPR:
-
Surface plasmon resonance
- TiO2 :
-
Titanium dioxide
- XRF:
-
X-ray fluorescence spectrometry
- ZnO:
-
Zinc oxide
- ZrO2 :
-
Zirconium dioxide
纳米材料