基于金纳米粒子检测体外糖化血红蛋白 A0 样品中的低分子量 AGE
摘要
蛋白质糖基化是一种主要的生化事件,由于糖水平升高而发生在糖尿病患者的血浆中。广泛的糖基化导致晚期糖基化终产物 (AGEs) 的形成,众所周知,AGEs 会对糖尿病患者产生不利影响。在目前的工作中,我们在体外对生理上重要的蛋白质血红蛋白 A0 进行糖化,以将其用作金纳米颗粒 (GNP) 合成的模板来研究 AGE 的形成和活性。发现合成的 GNP 的表面等离子体共振与糖化程度高度相关。在分级分离时,糖化血红蛋白 A0 分离成两个不同的产品群,一个由蛋白质、交联的血红蛋白 A0 的较大片段和第二个非蛋白质低分子量 AGEs 组成。在使用馏分作为模板时,只有低分子量 AGE 有助于 GNP 的合成,证实了所提出的基于 GNP 的测定的原理。由于其生理重要性,AGEs 可用作糖尿病及其相关并发症的诊断手段。在这项研究中,我们利用 AGE 的高反应性开发了一种基于 GNP 的新型比色传感器,以实现对它们的检测。我们提出的基于 GNP 的传感在检测糖尿病及其相关复杂性方面具有很高的临床意义。
背景
II 型糖尿病 (T2DM) 是一种复杂的代谢紊乱,其特征在于存在高血糖水平。体内持续存在的高血糖会引发一系列化学和生化反应,在高血糖过程中发生的最重要的反应称为美拉德反应,它涉及糖和蛋白质之间的非酶促反应,形成可逆的醛亚胺(席夫碱)连锁。相对不稳定的希夫碱互变异构为更稳定的酮形式,也称为“Amadori 产物”[1]。 Amadori 产物,也称为糖基化中间产物或早期糖基化产物,经过基团重排、环化、脱水、降解反应形成一系列化合物。已知这些化合物是蛋白质和糖基化剂的潜在交联剂 [2]。它们容易进一步降解,导致形成晚期糖基化终产物 (AGEs) [3]。在高内源性浓度下,AGEs 通过诱导蛋白质交联或通过受体介导的细胞内信号转导 (RAGE) 导致氧化应激和炎症 [5, 6],从而导致糖尿病并发症 [4]。已知它还与心血管疾病 [7, 8]、肾病 [9, 10]、视网膜病 [11, 12]、衰老 [13, 14]、关节炎、癌症 [15,16,17]、神经退行性疾病有关像阿尔茨海默氏症 [15] 和抑郁症的发展 [18]。除了美拉德反应外,其他几种途径包括葡萄糖氧化、脂质过氧化和多元醇途径也导致体内 AGEs 的形成 [19, 20]。除此之外,某些已知富含AGEs的食物的膳食摄入量也对体内总AGEs有贡献[6]。
尽管大多数 AGE 产品具有共同的结构元素 [21],但数百种 AGE 的确切化学结构仍有待确定 [22]。尽管它们具有异质性,但与蛋白质形成共价交联和“褐变效应”是所有已知 AGE 的典型特征 [23]。在糖化的早期阶段形成的涉及糖和蛋白质的加合物被称为早期糖基化加合物。果糖基赖氨酸和果糖胺就是这种结构的例子,它们是潜在的交联剂 [24,25,26]。一些被广泛研究的 AGE 包括 N -羧甲基赖氨酸 (CML), N -羧乙基赖氨酸 (CEL) [27]、戊糖素 [28]、葡萄糖烷 [29]、吡咯啉、Argpyramid、Crossline 和 Vesperlysine C [30]。
关于 AGEs 在糖尿病、衰老和相关并发症进展中作用的研究正在增加,据报道它们是相应研究的良好生物标志物 [https://clinicaltrials.gov/ct2/show/NCT02863224][ 31、32、33、34]。然而,AGEs结构的复杂性和异质性是开发通用检测系统的主要障碍[35]。 AGE 的定性分析通常通过光谱和比色技术进行 [36],其中美拉德反应产物的演变通过测量与早期美拉德反应产物相对应的 280 nm 处光吸收的增强 [37] 或通过测量450 nm 处的荧光发射 [38,39,40,41,42]。鉴定不同 AGE 产品的研究仍在进行中;然而,对戊糖苷 [43] 和羧甲基赖氨酸 [44] 的定量进行了一些尝试。大多数 AGE 是在组织水平上通过免疫组织化学和基于 ELISA 的量化使用一系列多克隆和单克隆抗体进行研究的 [21, 45]。迄今为止,可用于 AGE 检测的最佳分析技术是液相色谱或气相色谱,然后是分光光度法或质谱法检测 [12, 46,47,48,49]。 AGEs 的定量分析仍然是一个主要的技术挑战,少数可用的技术是昂贵的,因此限制了它在即时应用中的使用。由于 AGEs 对威胁生命的疾病的影响,因此需要开发用于定性和定量评估 AGEs 的新策略。
由于比色传感器检测简单、响应时间快和成本效益,特别是对于生物应用[50],这些传感器的开发已经付出了巨大的努力。其中,基于表面等离子体共振(SPR)的传感器由于其检测灵敏度高而受到特别关注[51]。在本研究中,我们探索了金纳米粒子 (GNP) 的光学特性,用于定性鉴定 AGE 产品。 GNP 以其独特的、可调节的 SPR 着称,因此进化为用于检测各种生物分子的比色报告基因。基于 GNP 的光学传感器包括用于小化学分析物 [52]、糖 [53]、不同蛋白质 [54]、蛋白质聚集体 [55] 和蛋白质构象变体 [56] 的光学传感器。以前,我们表明 GNP 在糖化蛋白质模板上播种时可以响应糖化的进展 [57]。在这里,我们通过利用 AGE 表现出的还原特性,扩展了这一概念,以比色法对不同糖基化产物进行定性检测。简而言之,HbA0 在体外被糖化,使用果糖作为还原糖,AGE 形成和相关的蛋白质主链结构变化通过光谱学得到证实。使用凝胶过滤色谱法对糖化 Hb 进行分级,以根据其分子量分离产物,并使用获得的级分合成 GNP。只有非蛋白质的AGE产物指导金纳米结构的合成,从而能够对其进行鉴定。
越来越多的证据支持不同的糖基化产物与疾病有关,包括糖尿病、衰老、阿尔茨海默氏症、肾病、动脉粥样硬化和不同类型的癌症。我们的研究结果强调了 GNP 作为一种简单且高度灵敏的比色传感平台的用途,可用于识别可能与糖尿病相关健康并发症预后有关的不同 AGE 产品。
方法
材料
血红蛋白 A0 (HbA0) 和 Sephadex (G25) 购自 Sigma Aldrich India Pvt。 Ltd. 氢四氯金酸盐 (III) 三水合物 (HAuCl4·3H2O) 购自 Loba Chemie。果糖、正磷酸二氢钾、正磷酸氢二钾、正磷酸二氢钠、正磷酸氢二钠、铁氰化钾、三氯乙酸、氯化铁、硝酸(HNO3)、盐酸(HCl)、丙烯酰胺、双丙烯酰胺、过硫酸铵、TEMED、钠十二烷基硫酸盐、考马斯亮蓝、甘油、二硫苏糖醇、TRIS 碱和其他使用的化学品均为分析纯,无需进一步纯化即可使用。所有实验均使用 MilliQ 超纯水 (>18 MΩ)。
方法
HbA0 的糖化
在高压灭菌的磷酸钾缓冲液(0.1 M,pH 7.4)中制备 HbA0 和果糖的储备溶液,并在使用前使用 0.2 μm 注射器过滤器过滤。将 HbA0 样品与不同浓度的果糖在 37 °C 的培养箱中培养不同的时间段(1 至 10 天)。果糖和 HbA0 的终浓度分别为 0.1 M 和 1 mg mL -1 分别。还保留了相同浓度的 HbA0 和果糖对照。糖化 10 天的样品标有前缀“第 10 天”,未孵育的对照样品标有前缀“第 0 天”。所有实验均在层流罩内无菌条件下进行。
减少属性分析
糖化样品的还原特性由Gu描述的方法测定。等。 [37] 稍作修改。然后,将 100 μL 糖化样品及其各自的蛋白质和糖对照与 1 mL 0.2 M 磷酸钠缓冲液和 1 mL 1% 铁氰化钾混合。将混合物在 50°C 的水浴中温育 20 分钟。将混合物冷却至室温后,加入 1 mL 10% 三氯乙酸。向 1 mL 该混合物中加入 1 mL MilliQ 水和 200 μL 0.1% 氯化铁。在700nm处测量所得混合物的吸光度。保留阴性对照,其中添加 0.1 M 磷酸盐缓冲液代替糖基化样品,并将其用作吸光度测量的空白。 700 nm处吸光度越高,还原性越强。
使用 Sephadex (G25) 的第 0 天 Fruc-Hb 和第 10 天 Fruc-Hb 的凝胶过滤色谱法
简而言之,将 Sephadex (G25) 珠子在 MilliQ 水中浸泡过夜,然后装入长 15 厘米、直径 1 厘米、内容积 15 毫升的玻璃柱中。首先,用大量 MilliQ 水和磷酸钾缓冲液(0.1 M,pH 7.4)洗涤柱子。在用磷酸盐缓冲液平衡后,将大约 600 μL 的 Fruc-Hb 样品加载到柱中。使用相同的缓冲液以每小时 7.5 mL 的流速进行洗脱。一旦加载的样品穿过柱子的大约 10 cm 距离,就收集级分。从每个样品中收集了大约 30 个馏分,然后对其进行表征。在这里,第 0 天 Fruc-Hb 及其衍生的馏分作为第 10 天 Fruc-Hb 样品及其各自馏分的对照。
金纳米粒子合成
所有用于 GNP 合成的玻璃器皿都用王水(HCl:HNO3,体积比为 3:1)洗涤,并在使用前用乙醇和超纯水冲洗(注意!王水是一种腐蚀性很强的氧化剂,应该小心处理 )。利用 Hb 糖化产物的还原特性,进行了 GNP 的合成。在室温 (RT) 下进行的典型实验中,32 μL HAuCl4 (1% w /v ) 在不断搅拌下加入到 3.868 mL MilliQ 水中。金盐完全溶解后,溶液变成淡黄色。接下来,将 50 μL 必需的 Fruc-Hb 样品或 100 μL 级分加入金盐溶液中。一旦所有反应物完全混合,就停止搅拌并且让反应混合物不受干扰以允许 GNP 生长。在最终反应混合物中,Fruc-Hb 样品的浓度为 12.5 ng μL -1 (12.5 μg mL −1 ) 并且馏分的量为 1 ng μL -1 (1 μg mL −1 )(详细计算在 S6 中给出)。根据加入的样品,反应混合物中出现从粉红色到紫色不等的颜色。所有样品均保持至反应混合物颜色稳定且未观察到进一步变化。
布拉德福德检测
使用 Bradford 测定分析从 Fruc-Hb 样品收集的级分是否存在蛋白质。简而言之,向 20 μL 未稀释组分中的每一个中加入约 200 μL Bradford 试剂,并在 595 nm 和 450 nm 处测量吸光度 [58]。将 595 nm 和 450 nm 处的 OD 比值与馏分数作图,较高的比值表示相对较高的蛋白质百分比。
光谱
紫外可见光谱
在 Perkin Elmer, Lambda 25 紫外-可见光谱仪中记录所有 Fruc-Hb 样品、它们各自的对照和来自糖化样品的色谱级分的紫外-可见光谱。通过以 240 nm/min 的扫描速度和 1 nm 的狭缝宽度运行从 800 到 200 nm 的扫描来获取光谱。所有测量均使用 1 cm 光程、1 mL 石英比色皿进行。 GNPs的紫外-可见光谱也以类似的方式记录。
荧光光谱
为了识别蛋白质结构改变和 AGE 形成,使用安捷伦科技公司的 Cary Eclipse 荧光分光光度计对 Fruc-Hb 样品和第 0 天 Fruc-Hb 和第 10 天 Fruc-Hb 的馏分进行荧光发射谱分析。激发和发射狭缝宽度设置为 5 nm,使用 1 cm 路径长度的石英比色皿获取光谱。样品分别在280 nm和350 nm激发,分别检测色氨酸荧光/内在蛋白荧光和AGE荧光[34,35,36,37,38]。
圆二色光谱
Fruc-Hb 样品中的二级结构改变使用圆二色光谱法进行鉴定。使用具有停止流动的圆二色性 (CD) 光谱仪、Applied PhotoPhysics Chirascan (Applied Photophysics Limited, UK) 进行测量。光谱在190~260 nm的远紫外波段拍摄。
对于所有光谱测量,0.1 mg mL −1 的 Fruc-Hb 样品 使用 0 天 Fruc-Hb 和第 10 天 Fruc-Hb 的级分,无需任何稀释即可进行分析。磷酸钾缓冲液 (pH 7.4, 10 mM) 在所有实验中用作空白。所有读数在室温下一式三份。
透射电子显微镜
为了确定从 Fruc-Hb 样品及其各自对照合成的 GNP 的大小和结构,使用透射电子显微镜 (TEM)-JEOL 2100F 进行电子显微镜分析。通过以 6000 rpm 的速度离心浓缩 GNP,并将其滴铸到 300 目大小的镀铜碳网格上。在分析之前,让样品在室温下干燥过夜。
GNP 的颜色强度分布
为了以可测量的值表达 GNP 的颜色,通过使用数字颜色从每种金胶体中提取红色、蓝色和绿色平面来计算 (RB)/G((红色强度-蓝色强度)/绿色强度)米。
SDS聚丙烯酰胺凝胶电泳
进行十二烷基硫酸钠-聚丙烯酰胺凝胶电泳 (SDS PAGE) 以观察糖化后 HbA0 的分子量差异。第 0 天、第 10 天的 HbA0 对照以及第 0 天和第 10 天的 Fruc-Hb 与含有 6X 上样缓冲液的 10% SDS 混合并煮沸 5 分钟,然后将 30 μL 样品加载到浇铸凝胶(堆叠凝胶- 5%;溶解凝胶 - 12%)。使用来自 Bio-Rad 的 Mini PROTEAN Tetrad 系统,在 100 V 下将样品与 10-175 kDa PiNK Plus 预染色蛋白阶梯(货号 PM005 0500)一起运行。凝胶在 Bio-Rad Trans 照明器的白色光源下可视化。
结果
从果糖、血红蛋白 A0 和糖基化血红蛋白 A0 合成 GNP
众所周知,还原糖葡萄糖在体内与 HbA0 反应产生 HbA1c,这是众所周知的早期糖基化加合物,它是与糖尿病相关的高血糖水平的主要生物标志物 [59]。为了在体外实现更快的糖化动力学和高 AGE 积累,使用果糖代替葡萄糖,与后者相比,葡萄糖是一种有效的糖化剂 [55,56,57,58,59,60]。在我们的研究中,我们使用果糖作为还原糖进行血红蛋白 A0 的糖化,并监测糖化直至 10 天,据报道这足以形成大量的 AGE [57]。图 1 说明了在体外与果糖孵育 10 天后 HbA0 样品中 AGE 的形成。通过在 350 nm 处激发后定性测量 450 nm 处的荧光发射来评估 AGE 形成 [38,39,40,41,42]。与非糖基化 HbA0(第 0 天 Fruc-Hb-HbA0 和果糖的物理混合物,无需孵育)相比,450 nm 处的荧光发射增加了十倍以上,证实了糖基化 HbA0(第 10 天 Fruc-Hb)中 AGE 产物的形成。样品的详细生物物理特征在附加文件 1 (S1 &S2) 中讨论。
<图片>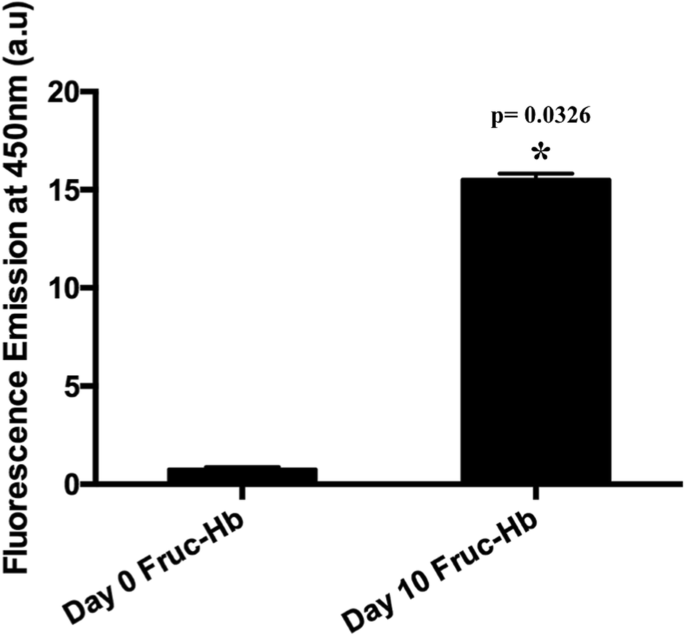
当血红蛋白与果糖孵育 10 天时,通过在 450 nm 处产生的荧光来测量 AGE 的形成。 Fruc-Hb 第 0 天和第 10 天 Fruc-Hb 450 nm 荧光发射的比较
图>为了将 GNPs 作为蛋白质糖化衍生的 AGEs 的比色传感器,研究糖和蛋白质反应物的 GNPs 形成动力学是先决条件。据报道,果糖和血红蛋白 A0 单独使用或与强还原剂结合使用时,可指导金纳米结构的合成 [53, 61,62,63]。然而,为了了解形成动力学的差异,我们比较了从 Fruc-Hb、果糖和 HbA0 在没有额外还原剂的情况下分别孵育 0 天和 10 天合成的 GNP。这些模板的还原特性是根据方法部分中描述的协议进行生化测量的。总体而言,第 10 天的样品显示出比第 0 天的样品更高的还原性,并且在这两种情况下,Fruc-Hb 表现出最大的还原性,其次是果糖和 HbA0,这可能归因于 Fruc-Hb 样品中 AGE 的存在。图 2a)。在这些样本中,在典型浓度下,只有第 10 天的 Fruc-Hb 有助于在 4 天结束时合成稳定的 GNP(图 2b),而其余的样本只有在允许它们的情况下才能合成保持较长时间(通常超过 10 天),结果与获得的样品还原特性一致(数据未显示)。第 10 天 Fruc-Hb_GNP 的可见光吸收光谱在 530 nm 附近产生了一个峰(图 2c),并且还通过透射电子显微镜(TEM)对颗粒进行了表征,以研究其尺寸和形态(图 2d)。电子显微照片显示存在平均直径为 21.930 ± 2.4 nm 的球形颗粒(图 2e)。此外,颗粒本质上是多晶的,其晶格点对应于 fcc 晶格的 111、200、220、311 个平面(图 2f)。 S3给出了差异糖化样品中GNP形成的详细动力学分析。
<图片>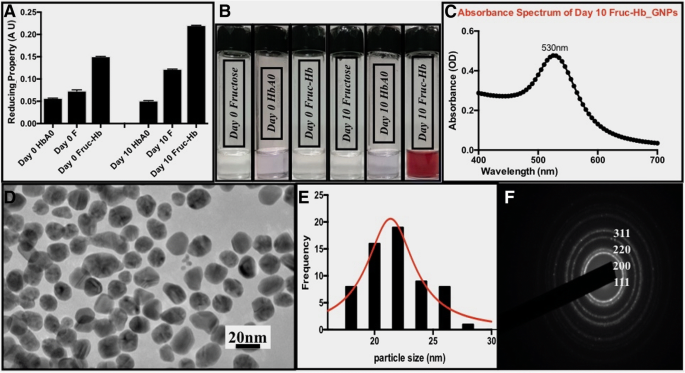
表征来自 HbA0、果糖和 Fruc-Hb 样品的 AGE 形成和 GNP 合成。 一 HbA0、果糖、Fruc-Hb 在培养 0 天和 10 天时的还原特性,分别通过三价铁离子还原试验测量。 b 从各个样品合成的 GNP 的照片。 c 第 10 天 Fruc-Hb_GNP 的紫外-可见吸收光谱。 d Day10 Fruc-Hb_GNPs 的透射电子显微照片(比例尺:20 nm)。 e 颗粒的尺寸分布和f 颗粒SAED
图>在这里,Fruc-Hb 作为模板和稳定剂来指导球形金纳米结构的合成。由于与糖和蛋白质对应物相比,只有第 10 天的 Fruc-Hb 能够在规定的时间内合成颗粒,因此该机制可用于区分糖化和非糖化蛋白质模板。
分馏 Fruc-Hb 可解决两类不同的糖基化产物
观察 AGE 或蛋白质骨架的改变是否是导致观察到的在第 10 天 Fruc-Hb 模板上接种的 GNP 中的差异 SPR 响应的原因很有趣。为了进一步研究,我们使用凝胶过滤色谱分离第 10 天的 Fruc-Hb 和第 0 天的 Fruc-Hb,如方法部分所述。来自第 0 天 Fruc-Hb 的级分用作对照来自第 10 天 Fruc-Hb 的级分。图 3 总结了馏分的光谱表征。图 3a 显示了从第 0 天 Fruc-Hb(红色)和第 10 天 Fruc-Hb(黑色)收集的馏分的洗脱曲线。 Fruc-Hb 分离得到的特征洗脱曲线与之前的报道一致[37]。
<图片>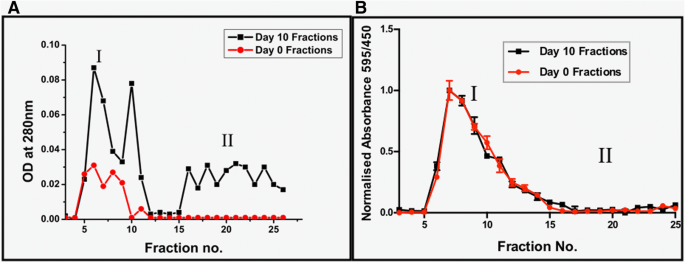
第 0 天 Fruc-Hb(红色)和第 10 天 Fruc-Hb(黑色)组分的洗脱曲线。在 280 nm (a ) 和 Bradford 检测是否存在蛋白质 (b ) 第 0 天 Fruc-Hb 和第 10 天 Fruc-Hb 的分数
图>蛋白质在第 0 天和第 10 天的馏分中的存在通过蛋白质中存在的芳香族氨基酸在 280 nm 处吸收紫外线来证实。通过 280 nm 光吸收测量的第 10 天 Fruc-Hb 的洗脱曲线显示存在两种标记为 I 和 II 的产物。群 I 由高分子量产物组成,范围从第 1 级开始。 5 到 12 和分数没有。 15 以后对应于低分子量产品属于第二类。在 I 和 II 之间观察到跨越 2-3 个分数的小范围,其中没有观察到紫外线吸收。另一方面,第 0 天 Fruc-Hb 的单个组分群显示出紫外光吸收(群 I),证实第 10 天组分中 II 区的吸收是由第 10 天 Fruc-Hb 产生的产物产生的的糖化。据报道,美拉德反应的中间体在近紫外范围内吸收光 [64],这支持了观察。与第 0 天部分相比,第 10 天部分对 280 nm 的紫外线吸收增强可能是由于糖基化导致蛋白质广泛展开,导致芳香族氨基酸暴露(图 3a)。>
如 S1 中所述,糖化显着改变了蛋白质的二级和四级结构。为了确认馏分中蛋白质的结构状态,我们对馏分进行了 SDS 聚丙烯酰胺凝胶电泳,发现在第 10 天 Fruc-Hb 样品中观察到融合带代替单体和二聚体带,证实了蛋白质的交联(附加文件 1:图 S4)。
在比较第 0 天 Fruc-Hb 和第 10 天 Fruc-Hb 的洗脱曲线时,我们发现落入群体 I 的级分本质上是蛋白质,而在第 0 天级分(非糖化)中完全不存在的第二个群体由非蛋白质糖化产物。这些发现得到 Bradford 测定的蛋白质估计的支持,其中证实了第 0 天和第 10 天 Fruc-Hb 群体 I 中级分的蛋白质性质(图 3b)。简而言之,凝胶过滤色谱法对第 10 天的 Fruc-Hb 进行分离,产生了两种不同的馏分群,均在 280 nm 处吸收光,一种是蛋白质性质的,另一种是非蛋白质性质的。
蛋白质糖化产物和非蛋白质糖化产物本质上是荧光的
了解了自然界中蛋白质和非蛋白质糖化产物的洗脱情况,在 450 nm 处测量了这些级分的荧光发射,以表征 AGE 产物的存在。与 UV 洗脱曲线类似,在第 10 天的馏分中观察到两组荧光发射,但第 0 天的馏分中没有一个在 450 nm 处显示荧光发射,因为在第 0 天 Fruc-Hb 中没有发生 AGE 形成(图 4)。荧光强度在第 10 天的馏分之间变化很大,表明糖基化产物的化学性质不同。在荧光洗脱图谱中,属于群体 I 的初始洗脱物由高荧光强度的糖化产物组成。这与图 3 中显示的蛋白质估计一起表明在这些级分中包含蛋白质结构的高荧光糖化产物的洗脱。与初始部分相比,第二个群体表现出较低强度的荧光发射。发现它们本质上是非蛋白质的(图 3b),但能够吸收 280 nm 的紫外光(图 3a)。
<图片>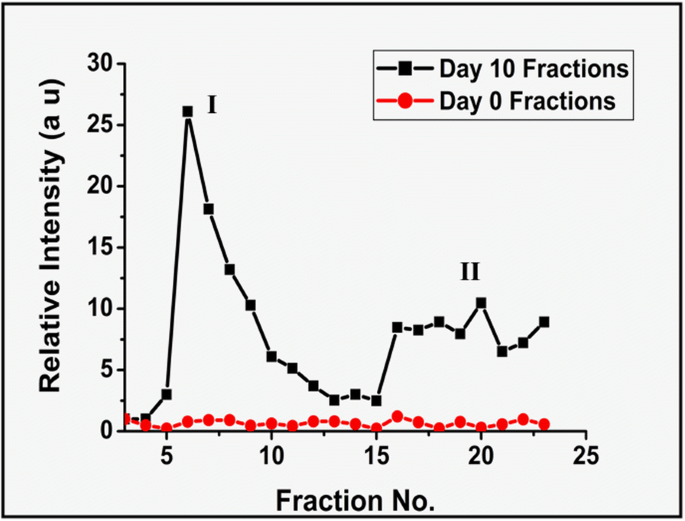
0 天 Fruc-Hb 和 10 天 Fruc-Hb 组分的 AGE 荧光比较,以 450 nm 处的荧光发射强度表示
图>考虑到第 10 天级分的 UV 吸收(图 3)和荧光发射(图 4),很明显该分级区分了 HbA0 的两种不同类型的糖化产物。最初从 G25 柱上洗脱的馏分具有高分子量、蛋白质性质,并在 450 nm 处发出强荧光。第二类产品是低分子量的非蛋白质结构,在 450 nm 处发出荧光,但强度相对较低。由于这两种产物都表现出“AGE”荧光,蛋白质结构可能是在美拉德反应过程中最初形成的与蛋白质主链交联的 AGE 产物,而第二个群体的部分可能是糖化的晚期产物,由此形成AGE-蛋白质交联的降解。
播种在 Fruc-Hb 样品馏分上的 GNP 可以区分糖化产物
很明显,GNP 的合成可用于区分糖化和非糖化模板(图 2)。现在,为了研究 GNP 是否可以区分第 10 天 Fruc-Hb 的蛋白质和非蛋白质洗脱物,我们使用从第 10 天 Fruc-Hb 获得的级分合成了 GNP,如方法中所述。监测形成的 GNP 的颜色,直到所有样品稳定。
如图 5 所示,由蛋白质结构组成的级分(群 I)没有一个表现出显着的 GNP 形成,而含有非蛋白质 AGE 产物的级分(群 II)产生了在胶体溶液中表现出一系列颜色的稳定 GNP。 GNP 的形成通过使用紫外-可见光谱进行表征,并且将吸光度最大值与分数数作图。光谱数据很好地支持了可视化的 GNP 颜色配置文件。该数据得出结论,基于 GNP 传感机制的糖化蛋白模板与非糖化蛋白模板的区分是由低分子量 AGE 产物介导的,相同的机制可用于区分蛋白质和低分子量非-蛋白质糖化产物。
<图片>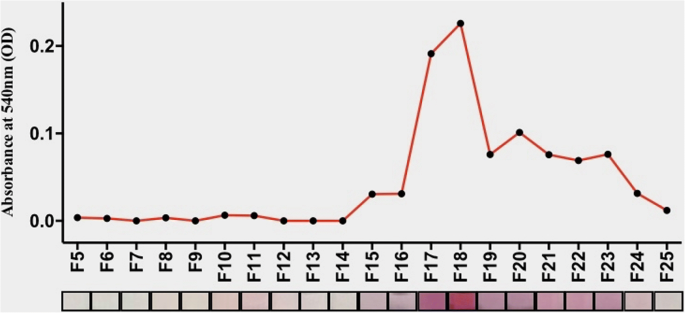
从第 10 天的 Fruc-Hb 级分合成 GNP。从第 10 天的馏分合成的 GNP 的比色曲线及其吸收最大值与馏分数的关系
图>通过简单地增加 Fruc-Hb 和金盐的浓度,可以将基于 GNP 的比色传感的动力学增强到更大的范围,从而缩短响应时间。 When the concentrations of the Fruc-Hb and gold salt was increased four times than the concentrations used initially in this study, GNP formation was completed within 1 day, substantiating the use of the proposed colorimetric sensor for point-of-care applications (Additional file 1:Figure S5).
The linearity of detection for this colorimetric sensor was also confirmed using different concentrations of the day 10 glycosylated HbA0 (day 10 Fruc-Hb) (Fig. 6). Colour formation was not prominent enough for the lower concentrations of day 10 Fruc-Hb used, and as the concentration was increased from 20 to 40 ng/μL, the colour intensity increased linearly. This confirms that the colour intensity profile extracted from the GNPs obtained from differentially glycated haemoglobin samples can be used for qualitative measurement of AGEs in respective samples.
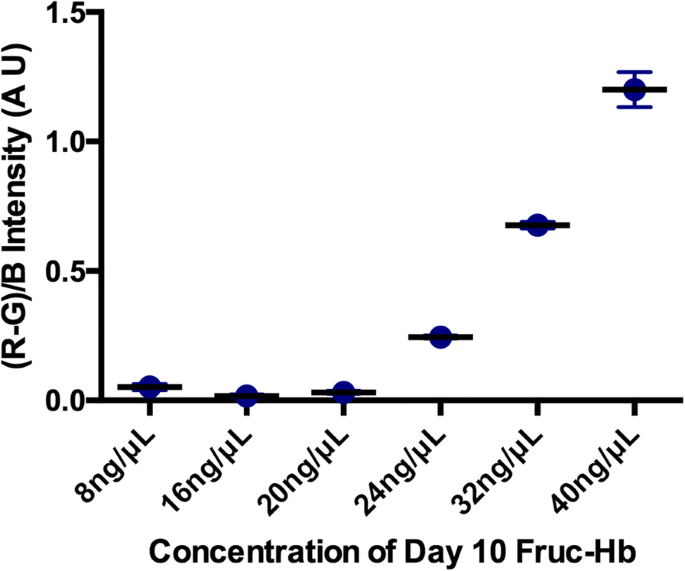
Linearity of detection. The red colour intensity as quantified by (R-G)/B intensity of GNP colloids plotted against the concentration of day 10 Fruc-Hb used for the synthesis. The concentration was varied from 8 to 40 ng/μL
图>Discussion
The study presented here provides a detailed mechanism of GNP formation from a glycosylated HbA0 template and also discusses how this technique can be expanded for highly sensitive detection of AGE products in a simple manner. Our primary objective for this work was to establish a colorimetric sensing mechanism based on GNPs for the differentiation of glycated and non-glycated samples. The glycated HbA0 was found to be having high reducing property in comparison to the protein and sugar counterparts, enabling formation of stable and mono dispersed GNPs (Fig. 2). Since, among the control samples tested for GNP synthesis, only day 10 Fruc-Hb showed AGE fluorescence, the reactivity can be attributed to the AGE products than a mere structural alterations of the due to glycation (Figs. 1 and 2). To confirm this norm further, the products of glycation obtained from day 10 Fruc-Hb was fractionated to separate the products according to their molecular weights.
Fractionation of glycated Hb segregated two major population of products, high molecular weight proteinaceous (population I) and low molecular weight non-proteinaceous (population II) glycation products (Figs. 3 and 4). When GNPs were synthesised using these fractions of day 10 Fruc-Hb, it was found that only the products belonging to population II, the non-proteinaceous glycation products, were capable of carrying out the synthesis of particles (Fig. 5). This confirmed that the non-proteinaceous AGE products can initiate GNP synthesis by their own and the formation of GNPs from a Fruc-Hb template can be clearly attributed to the AGEs. Also, this opens up the possibility of using this property for the colorimetric detection of AGE products along with the distinction between proteinaceous and non-proteinaceous glycation products of HbA0.
Generally, CARBONYL groups present in the protein and sugar enable the stable synthesis of gold nanostructures in biological syntheses. Here, the generation of AGE products consisting of dicarbonyl functional groups during glycation might be providing the reducing environment for the proposed GNP synthesis [65]. As a matter of fact, the suggested mechanism can be used for the detection of advanced lipid per-oxidation end products (ALEs) as well, since the latter is also characterised by dicarbonyl functional groups and shares structural similarities with AGEs [66]. In short, GNPs synthesised from fractionated glycosylated Hb samples can clearly distinguish between proteinaceous and non-proteinaceous products. Our GNP-based simple colorimetric sensing of glycation products is highly sensitive and can detect nanogram levels of glycation products (S6) in a concentration-dependent manner (Fig. 6). The detection limit using the proposed sensing mechanism for the fractions is 1 ng/μL. The method developed in here can be scaled down to smaller reaction volumes without affecting the reaction kinetics, thus enabling lower sample requirements (data not shown) as well as enhance the reaction kinetics by increasing the concentrations (S5).
Conclusions
Concisely, in this study, we have demonstrated a colorimetric sensing mechanism for the non-proteinaceous AGE products which is simple and highly sensitive with a detection limit down to nanogram levels. Conditions like type 2 diabetes requires continuous monitoring of sugar levels at regular time intervals. Currently, levels of HbA1c formed as a result of extensive glycation of haemoglobin is used as a diagnostic means for diabetes. Chromatographic [67], electrophoretic and advanced technologies including high-performance liquid chromatography (HPLC) and mass spectrometry (MS) [12, 46,47,48,49, 68] are used for HbA1c detection. Early glycation adducts including fructosamines are also indicative of the glycemic control [69] which can be used as a marker for diabetes detection. Monitoring the AGE levels in addition to the HbA1c levels can significantly improve the determination the degree of complexity associated with diabetes, since the advancement of the disease is often associated with AGE formation and is involved in the progression of the disorder as well. Till date, the LC-MS/MS offers the highest selectivity and sensitivity in AGE detection [70]. But when it comes to simple, cost-effective, point-of-care diagnostics, our method can detect few nanograms of the sample compared to other fluorescence emission-based techniques [71, 72]. The method is highly specific for the AGEs, such that sugars and proteins do not develop any colour as such which are the expected interferences in the clinical samples such as blood or serum for the proposed study (Additional file 1 section 7). In this study, we have also segregated the products of glycation to proteinaceous and non-proteinaceous components and proved that non-proteinaceous AGEs are more reactive by the GNP based colorimetric assay. Further research can explore how this idea can be expanded for the distinction between different AGEs and thereby apply it for the diagnosis of organ specific diabetes-related complications.
缩写
- AGEs:
-
Advanced glycation end products
- Fruc-Hb:
-
Glycated Hb
- GNPs:
-
Gold nanoparticles
- HbA0:
-
Haemoglobin A0
- SPR:
-
Surface plasmon resonance
纳米材料