用贵金属纳米粒子装饰的电纺聚合物纳米纤维用于化学传感
摘要
不同的贵金属纳米结构表现出理想的等离子体和/或电催化性能,与电纺聚合物纳米纤维的整合,表现出独特的机械和热力学性能,产生具有协同性能和功能的新型混合纳米级系统。本综述总结了如何将贵金属纳米粒子掺入电纺聚合物纳米纤维的最新进展,并说明了这种集成如何为提高灵敏度、稳定性、灵活性、兼容性和选择性的化学传感应用铺平道路。预计该领域的进一步发展最终将对许多研究领域产生广泛影响。
评论
背景
纳米科学和纳米技术的快速发展导致了广泛的实际应用,包括空气过滤、伤口敷料、药物输送、检测、能源生产和食品包装 [1,2,3,4,5,6,7, 8,9,10]。纳米材料的物理和化学性质往往与更大规模的相同材料有很大不同。已经开发了许多不同的策略来合成和构建纳米结构材料 [11,12,13]。根据维度,纳米材料可以分为四类:零维(0D)、一维(1D)、二维(2D)和三维(3D)。一维纳米材料,如纳米线、纳米棒和纳米管,在过去的几十年里得到了广泛的研究。在上述材料中,一维纳米纤维因其独特的结构和物理特性而引起了极大的关注,例如小直径、大单位质量表面积、小孔径和表面功能的灵活性[14, 15]。有许多加工技术已被用于生产一维纳米纤维,例如模板合成 [16]、自组装 [17] 和静电纺丝 [18, 19]。在这些方法中,静电纺丝似乎是制备纳米纤维最通用和最简单的一种 [15]。值得注意的是,通过调整聚合物溶液或静电纺丝装置的参数,大多数已知聚合物如聚丙烯腈 (PAN) [20, 21]、聚偏二氟乙烯 (PVdF) [18] 和聚乙烯醇 (PVA) [22 ] 可以成功地静电纺成超细纤维。因此,由于静电纺丝具有显着的简单性和多功能性,近年来静电纺聚合物纳米纤维受到了广泛关注,尤其是在化学传感器领域。
处理纳米级吸附分子和贵金属结构之间光-物质相互作用的等离子体领域最近成为一个快速增长的兴趣领域,包括表面增强拉曼散射 (SERS) 在内的各个领域的爆炸性增长证明了这一点[23,24,25],表面增强红外吸收光谱 [26, 27],表面增强荧光光谱 [28,29,30],表面等离子体共振光谱 [31,32,33,34] 和等离子体比色法[35]。等离子纳米结构迷人的光学特性由称为表面等离子激元的贵金属(例如金、银和铂)纳米结构中导带电子的集体振荡支配。对制造可重复等离子体纳米结构的简单方法的探索激发了对各种科学学科的浓厚兴趣。然而,在基板上的预定义位点分层组装具有理想长程有序的单个贵金属纳米结构仍然是一个巨大的挑战。纳米级等离子体构建块的模板合成和组装以形成合理设计的架构已成为解决这一挑战的总体策略 [36,37,38]。电纺聚合物纳米纤维已被证明是最有前途的模板之一,可以高精度地填充贵金属纳米结构。将具有所需等离子体特性的贵金属纳米结构可控地掺入到电纺聚合物纳米纤维中,为提高灵敏度、稳定性、灵活性、兼容性和选择性的传感应用铺平了道路。
本综述重点介绍了将电纺聚合物纳米纤维与贵金属纳米颗粒相结合的最新进展及其在化学传感中的应用。我们总结了以下内容:(1) 静电纺丝的基本设置和工艺参数,(2) 合成 Au 或 Ag 纳米结构的不同策略,(3) 用 Au 或 Ag 纳米粒子装饰的电纺聚合物纳米纤维的制备,以及 (4) Au或Ag纳米粒子修饰的电纺聚合物纳米纤维的化学传感应用实例。
电纺:基本设置和工艺参数
静电纺丝系统一般由四个主要部分组成:高压直流电源、装有聚合物溶液的注射器、带有钝头的金属针和接地的导电集电极,如图 1 所示。过程中,注射器中的聚合物溶液将以特定速率通过金属针尖泵出。施加高电压在聚合物液滴表面产生电荷形成泰勒锥,当排斥静电力足够强以克服聚合物液滴的表面张力时,聚合物液滴会被拉长成圆锥形。 39]。随后,聚合物射流将经历一个伸长过程,在此期间聚合物将被拉伸,聚合物溶液溶剂将蒸发,留下长而细的聚合物纳米纤维收集在接地的导电集电器上。
<图片>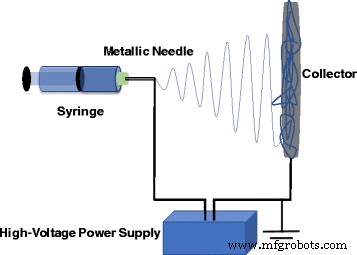
静电纺丝的基本实验室设置
图>静电纺丝的一大优点是通过改变静电纺丝过程中的参数,可以轻松控制静电纺丝纳米纤维的形态。这些参数包括聚合物浓度、溶液粘度、溶液电导率、流速、施加的电压、收集器和针尖之间的工作距离以及空气湿度 [12]。聚合物浓度是一个重要参数,因为它决定了电纺纳米纤维的形态,因为随着聚合物浓度的降低,表面张力可能占主导地位,这将导致聚合物珠的形成 [39]。此外,溶液粘度是另一个关键参数,它决定了聚合物是否可以电纺成纳米纤维。溶液粘度高度依赖于聚合物浓度和用于静电纺丝的聚合物的分子量。原则上,分子量较高的聚合物平均具有较长的分子链,并且会形成更多的缠结,从而导致聚合物溶液的粘度较高。因此,对于由高分子量聚合物制成的溶液,即使聚合物浓度较低,但由于溶液粘度足够高,仍然可以产生均匀的射流。相反,如果分子量太低,即使聚合物浓度高也不能保证合适的聚合物溶液粘度,并且聚合物倾向于在集电体上形成珠状结构[40]。相比之下,施加电压和流速等加工条件在静电纺丝过程中对纳米纤维的形成也起着重要作用。对于外加电压,已经证明不同的外加电压不会显着改变纳米纤维的形态。根据过去的工作,当施加更高的电压时,可以获得更大和更小的纤维直径[40]。
金或银纳米结构的合成与组装
在过去的几十年中,在合成不同尺寸和形状的 Ag 和 Au 纳米结构方面取得了很大进展。值得注意的是,不同的纳米结构可以产生显着不同的光学、电子、磁性或化学性质,这可能适用于不同的应用。通常,根据不同的机理,还原金或银纳米结构的方法可以大致分为化学方法和物理方法。通常,获得Au或Ag纳米结构的方法是将Au或Ag前驱体与还原剂和/或胶体稳定剂混合,在特定条件下可以生成不同尺寸和形状的纳米结构Au或Ag。 AgNO3和HAuCl4是Ag和Au纳米结构合成最常用的前驱体,硼氢化钠、醇类、柠檬酸钠和聚乙烯吡咯烷酮(PVP)等各种还原剂可以将Ag/Au离子还原成Ag/Au原子对它们的大小和形状有特殊的控制。已经证明,Au 或Ag 纳米粒子的等离子体共振频率取决于它们的尺寸。例如,Xia 及其同事合成了 60 到 100 nm 的银纳米立方体,并比较了它们的 SERS 的大小和形状(尖锐与截断)[39]。它表明,发现较大的颗粒(90 和 100 纳米)具有更高的 SERS 效率(90 和 100 纳米),这主要归因于激光源和等离子体共振带之间的重叠。此外,与截断的对应物相比,具有成形角的粒子也提供了更强烈的 SERS 信号。
金纳米结构的合成
基于 Turkevich 在 1951 年的研究,HAuCl4 可以在柠檬酸盐存在的水溶液中被还原,这是合成 Au 纳米颗粒最常用的方法之一 [41]。通过改变柠檬酸盐的量,可以轻松控制 Au 纳米颗粒的平均尺寸,并且柠檬酸盐同时起到成核剂和生长剂的作用 [41]。已经证明柠檬酸盐还原法可以产生相对较窄的Au纳米颗粒尺寸分布。随后的研究表明,不同的Au纳米颗粒尺寸对柠檬酸盐含量的控制机制与pH值密切相关,因为不同的pH值将决定Au纳米颗粒的形成过程[42]。
1994 年,Brust 和 Schiffrin 通过发明两相合成策略,为 Au 纳米结构的合成做出了巨大贡献。在这种方法中,AuCl4 − 使用四辛基溴化铵作为相转移试剂将其从水溶液转移到甲苯中,并利用硫醇 - 金相互作用来保护具有硫醇配体的 AuNP。在十二烷硫醇的存在下,通过硼氢化钠 (NaBH4) 的还原反应获得尺寸范围在 1 到 3 nm 之间的 Au 簇(图 2)[41]。随着有机相中加入NaBH4,溶液的颜色立即变成深棕色。可以改变包括金/硫醇比、温度和还原率在内的几个参数来控制所得金纳米粒子的尺寸。例如,较大的硫醇/金摩尔比导致 Au 纳米粒子具有较小的平均核尺寸 [43]。利用不同的配体形成单层保护的金簇和硫醇与AuCl4的比例 - 可以在合成中进行调整以控制 AuNPs 的大小。种子介导的生长,由 Jana 等人开发。还显示出生成具有受控和单分散粒径的 Au 纳米粒子的巨大希望 [44,45,46,47]。在一个典型的过程中,需要高质量的种子,然后在多个步骤中生长圆柱形 Au 纳米结构。在种子介导的生长方法中,Au纳米结构的产量相对较低,需要高质量的种子[48]。
<图片>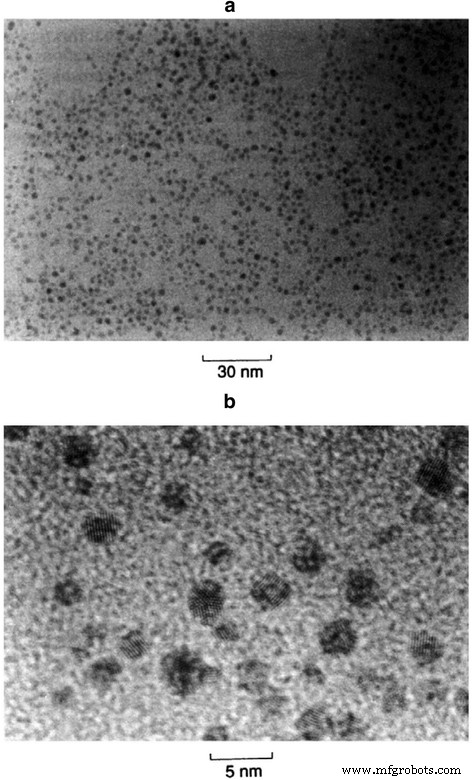
a处硫醇衍生的金纳米粒子的TEM照片 低和b 高倍率 [147]。经[147]许可转载。版权所有 {2010} 英国皇家化学会
图>各种聚合物已被报道用于稳定 Au 纳米粒子,包括 PVP、聚(乙二醇)(PEG)、PVA、聚(乙烯基甲基醚)(PVME)、壳聚糖和聚乙烯亚胺(PEI)[49,50,51 ,52,53,54,55,56,57]。不同的聚合物呈现出不同的金纳米结构形成过程;例如,金离子和 PVP 之间的还原可能涉及固-液 (S-L) 机制,氮和氧原子杂环有助于 PVP 的还原能力 [56]。在还原反应中,PVP既是还原剂又是空间稳定剂;因此,通过改变PVP和Au离子的浓度或比例,可以获得不同形状和尺寸的不同Au纳米结构(如图3所示)。
<图片>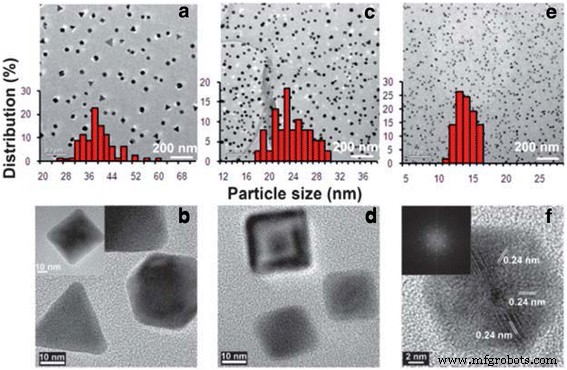
来自 AuNPs-PVP 纳米复合薄膜的 AuNPs TEM 图像和直方图,HAuCl4 与 PVP 的重量比为 [HAuCl4/PVP] =1:1.5 (a , b ), 1:2 (c , d ) 和 1:4 (e , f ) [88]。经参考许可转载。 [67]。版权所有 {2010} 英国皇家化学会
图>除了 Au 纳米结构的化学合成策略外,还使用了几种物理方法来提高 Au 纳米结构的质量,包括光化学(UV、近红外)、声化学、辐射分解、热解和微波辐射 [58,59 ,60,61,62,63,64,65]。在微波辐射合成过程中,添加不同量的油酸不仅提高了生长速度,而且还控制了所得金纳米结构的形貌,如图 4 所示 [65]。此外,还可以加入油胺作为还原剂,成核的金作为催化剂引发油酸和油胺反应形成二油酰胺,二油酰胺作为制备的金纳米粒子的封端剂。
<图片>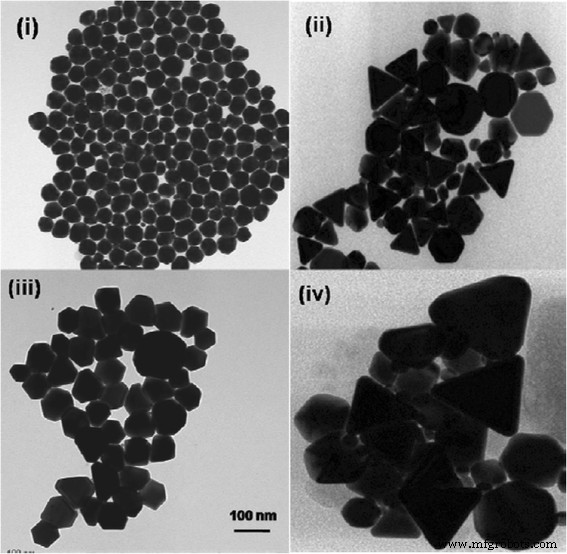
i制备的金纳米粒子的TEM图像 60、二 70、iii 80 和 iv 90% 油酸 [65]。经参考许可转载。 [65]。版权所有 {2010} 美国化学会
图>银纳米结构的合成
几十年来,人们已经发现使用柠檬酸盐作为水溶液中银胶体合成的还原剂。通常,将一定量的柠檬酸钠溶液加入沸腾的 AgNO3 水溶液中,保持系统沸腾 1 小时后将获得 Ag 纳米晶体。在反应过程中,柠檬酸根离子既是还原剂又是稳定剂,它们可以与银种子络合,从而影响颗粒生长,导致形成更大的银簇[66]。此外,通过改变溶液的pH值或柠檬酸根离子的浓度,可以实现与柠檬酸根离子相关的不同质子化状态,从而导致不同的Ag簇生长机制和形态[13]。
另一种合成银纳米结构的常用方法是多元醇法,它可以形成具有多种尺寸和形状的银纳米结构 [67,68,69,70,71]。在多元醇还原过程中,成核、生长过程和所得的 Ag 纳米结构形态对反应条件敏感,例如温度、试剂浓度和痕量离子的存在 [13]。在典型的多元醇还原过程中,将带有封端剂的 Ag 前体注入预热的多元醇中,例如乙二醇; 1,2-丙二醇;或 1,5-戊二醇,它具有作为溶剂和还原剂的双重作用 [13, 66]。由于其复杂的性质,多元醇还原过程的确切机制仍然在很大程度上未知,可能的反应之一如下:
$$ 2\mathrm{H}\mathrm{O}\mathrm{C}{\mathrm{H}}_2\mathrm{C}{\mathrm{H}}_2\mathrm{O}\mathrm{H}\ kern0.5em +\kern0.5em {\mathrm{O}}_2\to\2\mathrm{H}\mathrm{O}\mathrm{C}{\mathrm{H}}_2\mathrm{C}\mathrm {H}\mathrm{O} + 2{\mathrm{H}}_2\mathrm{O} $$ (1)通过光谱方法,乙醇醛(GA)的形成已被证实,它是乙二醇的中间产物和更强的还原剂,可以有效地将AgNO3还原为Ag[72]。这也可以解释为什么多元醇过程高度依赖于反应温度 [13]。在多元醇反应过程中,Ag 原子最初形成小簇,然后成长为稳定且更大的簇。最后,经过不断的生长,会形成不同形状和尺寸的Ag纳米结构。
此外,硝酸银在含醛化合物(或糖,如葡萄糖)的存在下,可以形成托伦试剂,随后通过还原反应转化为元素银:
$$ \mathrm{RCHO} + 2\ {\left[\mathrm{Ag}{\left(\mathrm{N}{\mathrm{H}}_3\right)}_2\right]}^{+} + 2\ \mathrm{O}{\mathrm{H}}^{\hbox{-}}\to \mathrm{RCOOH} + 2\ \mathrm{A}\mathrm{g} + 4\ \mathrm{N} {\mathrm{H}}_3 + 2\ {\mathrm{H}}_2\mathrm{O} $$ (2)该反应也称为银镜反应,会在反应容器的内表面产生闪亮的镜面涂层[13]。然而,该反应无法实现形状控制,限制了其在合成银纳米结构中的应用。
使用纳米晶体作为种子进一步生长的种子介导生长引起了很多关注,并成为另一种流行的银纳米结构合成方法。基本上,涉及两个主要步骤:种子成核和纳米结构的生长。这两个步骤基本上是分开的,可以很好地控制 Ag 纳米结构的最终形态 [13, 67]。对于通过这种方法合成的 Ag 纳米结构,纳米结构的最终形状不仅取决于初始种子,还受不同晶面的生长速率控制 [13]。已经发现,特定面的生长速率受封端剂的显着影响。例如,当用作封端剂时,柠檬酸盐与 {111} 晶面的结合比 {100} 晶面更牢固,倾向于形成纳米片。然而,对于 PVP,它与 {100} 晶面的结合比 {111} 晶面更牢固,因此可以降低沿 [73] 方向的生长速率,导致不同的形态形成。这些研究表明,通过改变反应条件,包括封端剂和种子类型,。可以简单地操纵不同面的结合强度,从而精确控制Ag纳米结构的形貌[13]。
很久以前,人们发现银前体(例如 AgNO3)可以与光相互作用,从而形成元素银。因此,在适当的化学物质存在的情况下,可以在银胶体样品的激光照射下形成银纳米结构 [13]。早期研究发现,当超快(飞秒或纳秒)激光脉冲应用于银纳米结构时,这些纳米结构会熔化并由于这种形状的低表面能和热力学稳定性而倾向于形成粗糙的球体 [13, 74, 75]。受这些早期研究的启发,后来的研究表明,光激发也可用于以可控方式生长或修改纳米结构,并且发现所得纳米结构的大小和形状取决于所施加的激光波长和功率 [76,77, 78,79]。最近的研究表明,柠檬酸盐、氧气和光是反应所必需的。光介导合成背后的机制如下:吸收/散射光的Ag种子弱还原分子氧并释放Ag + 成溶液;在有光和 Ag + , 柠檬酸盐会降解为乙酰乙酸盐,产生的电子转移到银纳米结构中,加速银在表面的沉积速度 [13, 78, 80]。通过增加照射光强度,可以显着增强光化学过程,从而提高光反应速率和Ag纳米棱柱的产率[78]。
用贵金属纳米粒子装饰的电纺聚合物纳米纤维
银和金纳米结构已被证明是用于各种应用的多功能平台,例如等离子体、生物医学研究、传感和催化 [81,82,83,84,85,86]。利用电纺聚合物纳米纤维的柔韧性、均匀分布、可控形态和自支撑特性,金或银纳米结构与聚合物纳米纤维的结合具有提高可重复使用性和拓宽当前应用的巨大潜力。例如,值得注意的是,将银纳米颗粒封装到聚合物纤维基质中可以有效地防止银纳米颗粒表面的硫化。将 Au 或 Ag 纳米结构添加到电纺纳米纤维中也可以改变纳米纤维的形态。 Kim 及其同事合成了 Au NP/PEO 复合材料,发现将 Au NP 添加到 PEO(聚(环氧乙烷))中后,纤维直径增加了 50 nm [87]。
用银纳米粒子装饰的电纺聚合物纳米纤维的制备
基于Ag + 还原过程的顺序 对于银纳米结构,用银纳米粒子装饰的电纺聚合物纳米纤维的制备可分为两种不同的方法。在第一种方法中,要么首先制备具有不同形态的银纳米结构,要么在聚合物前体溶液中将银前体还原成银纳米结构。如果还原反应在单独的溶液中进行,那么制备的 Ag 纳米结构将被分离并随后添加到聚合物前体溶液中。这样,由于银纳米结构的还原发生在电纺纳米纤维形成之前,因此不需要可以溶解和稳定银前体的溶剂。此外,聚合物不必能够还原Ag前体,这意味着可以不受限制地将合适的聚合物用于复合材料。此外,由于在依赖还原过程中更容易控制Ag纳米结构的形态,单独的合成过程允许具有更多Ag纳米结构形态的Ag纳米结构/电纺纳米纤维复合材料。
另一种制备用 Ag 纳米粒子装饰的电纺聚合物纳米纤维的方法包括首先将 Ag 前体溶解到聚合物前体溶液中或将其附着在电纺聚合物纳米纤维的表面上, 然后通过还原过程将 Ag 前体转化为 Ag 纳米结构。这种方法也称为等离子体纳米粒子的原位生长。通常,为了进行还原反应,其中一种方法是利用还原聚合物或包含还原聚合物的混合聚合物作为电纺纳米纤维前体,例如壳聚糖和 PVP [88, 89,90,91]。 PVP 如何将 Ag 前体还原为 Ag 纳米粒子的确切机制仍不完全清楚,并且已经假设由羟基端基氧化产生的醛官能团可能以类似的方式还原金属离子托伦试剂[92]。此外,值得指出的是,当使用相同质量的聚合物时,金属形成能力高度依赖于 PVP 的分子量 [88]。其他降低 Ag + 的方法 聚合物纳米纤维内部或外部的处理包括加热、紫外线照射、微波照射或氢还原 [93,94,95,96,97]。伦纳德等人。制备了电气石纳米颗粒/聚氨酯纳米纤维复合材料,并在表面装饰了亚硝酸银 [98]。辐照处理4 h后,硝酸银被还原成Ag纳米颗粒,在复合材料表面呈现线状结构。
用金纳米粒子装饰的电纺聚合物纳米纤维的制备
与封装 Ag 纳米结构的策略类似,大多数研究人员表明,可以首先使用常规的 Au 纳米结构方法(例如柠檬酸盐还原和种子介导的方法)合成用 Au 纳米粒子装饰的电纺聚合物纳米纤维,然后将制备的 Au 纳米结构分散到静电纺丝聚合物前体溶液 [73, 99,100,101,102]。对于某些特定应用,需要在纳米纤维表面装饰 Au 纳米结构,并且发现 Au 纳米结构会被聚合物上的某些特定官能团所吸引。通过调整 Ag 或 Au 溶液的 pH 值,Dong 等人。发现来自NPs表面结合柠檬酸盐的三个COONa基团之一会变成COOH,它可以通过两个分子间氢键桥接尼龙6纤维表面的酰胺基团,并结合表面的Ag或Au NPs如图 5 所示的尼龙 6 纳米纤维 [103]。
<图片>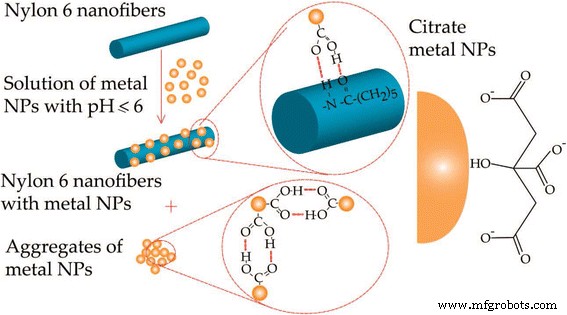
pH 诱导金属纳米粒子在尼龙 6 纳米纤维表面组装的假定机制 [103]。经参考许可转载。 [103]。版权所有 {2008} 美国化学会
图>一些聚合物在其主链上含有官能团,这些官能团可以很容易地用其他材料如 3-巯基丙基三甲氧基硅烷 (MPTES) 进行改性,以提供更强的结合位点来吸引 Au 纳米粒子 [99]。此外,一些聚合物可用于稳定Au纳米结构并在Au 3+ 的还原过程中作为电子供体 到 Au 0 .普奇等人。发现在辐照下,PVA 中具有可用 α 氢原子的 RCH2OH 可以转化为 RCHO,同时释放 H + 和 e − [52]。随后,产生的 e − 可能被 Au 3+ 困住 产生 Au 0 使PVA添加剂更有效的光还原反应[104]。
贵金属纳米粒子装饰的电纺聚合物纳米纤维的传感应用
表 1 中说明了基于电纺聚合物纳米纤维装饰的贵金属纳米粒子(例如,Au 和 Ag NP)的传感应用的一些最新示例。从表 1 中可以看出,金属粒子/纳米纤维复合材料具有许多优点,例如简单、高灵敏度、高选择性检测各种生化标本。通过静电纺丝,金属颗粒/纳米纤维复合材料可以很容易地制造出高表面积,这可以为检测分子提供方便的途径,从而导致 SERS 的优异活性。因此,许多金属颗粒/纳米纤维复合材料显示出低检测限。此外,基于表 1 中的结果,可以得出结论,金属颗粒的密度和尺寸对 SERS 活性/灵敏度有重要影响,因为拉曼信号的增强是由于金属颗粒之间/之间存在热点.
图>用贵金属纳米粒子修饰的电纺聚合物纳米纤维用于基于 SERS 的化学传感
表面增强拉曼散射 (SERS) 已成为探测单分子、离子、生物分子和细胞研究的最有前途和最强大的分析工具之一 [105,106,107,108,109,110,111]。自 1980 年代中期以来,更多的研究人员开始专注于探索有前景的 SERS 分析应用,而不是对该现象的基本理解 [112]。有组织的 Au 或 Ag 纳米结构因其作为 SERS 基底的信号放大功能而引起了极大的关注,这归因于金属纳米结构引起的局部电磁场增强。 SERS 增强因子(存在和不存在纳米结构时来自给定数量分子的拉曼信号之间的比率)与产生效应的纳米结构的大小和形状密切相关 [113]。通常,将金、银或金银混合纳米结构排列在刚性材料上作为 SERS 基底,这些方法要么合成过程复杂耗时,要么需要严格的合成条件。
Recently, a flexible substrate fabricated by combining electrospun nanofibers with Au, Ag, or AuAg-mixed nanostructures has become popular due to their excellent SERS performance and, compared with the rigid substrate, these flexible structures are adaptable to a rough substrate in terms of wrapping and bending [106, 114]. These metal/nanofiber composites demonstrated a 3D structure, which can provide high density of “hot spots”, which refers to the regions of highly enhanced local electromagnetic field [115]. In addition, the polymer outside the nanostructures can protect them from the surrounding environment especially for Ag nanostructures, which gives the composite long lifetime and high sensitivity [116].
Different polymers or ceramic nanofibers, such as PVA [100, 116, 117], cellulose [118], poly(methyl methacrylate) (PMMA) [119], chitosan [106], poly (acrylic acid) (PAA)/PVA [120], and silica [121] have been utilized to combine with different Ag or Au nanostructures to fabricate the flexible substrate for SERS. PVA is a nontoxic, biocompatible polymer, which has good electrospinability, and it is a popular material for electrospinning. When it is used as the supporting material for Ag nanostructures for SERS, it functions not only as the host matrix but also as an organic additive inducing the aggregation of individual Ag nanostructures [116]. In a typical process, the Au or Ag nanostructures are produced in specific morphologies first and these nanostructures are added into the polymer solution as the precursor solution.他等人。 synthesized nearly monodispersed Ag NPs via a microwave-assisted method and then these as-prepared silver dimers and aggregates were mixed into a 7% aqueous PVA solution for electrospinning [116]. In order to reduce the specific surface area and the surface Gibbs free energy of individual nanofibers, Ag NPs were self-assembled inside the PVA nanofibers. The assembly of Ag dimers or aligned aggregates within PVA nanofibers was confirmed using transmission electron microscopy (TEM) and X-ray photoelectron spectroscopy (XPS) analyses. Moreover, Ag NPs tended to form a linear chain-like structure along the axial direction of fibers (Fig. 6) because when a high voltage was applied to the solution, Ag NPs became positively charged on one side and negatively charged on the other, leading to a self-alignment by electrostatic attraction in the direction of the electric field [116].
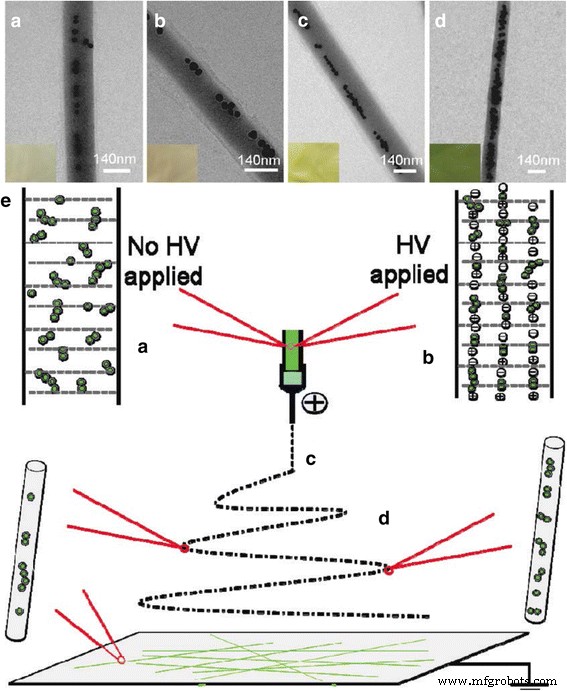
一 –d Typical TEM image of Ag/PVA nanofibers with the molar ratio of PVA/Ag 530:1 (a ), 530:2 (b ), 530:3 (c ), and 530:4 (d )。 The increase of the molar ratio of Ag/PVA in the Ag/PVA solution led to stronger aggregation state and a larger distribution in the sizes of the aggregated Ag NPs. e Schematic representation of the formation of chain-like arrays of Ag NP aggregates within PVA nanofibers [116]. Reprinted with permission from ref. [116]. Copyright {2009} American Chemical Society
图>As the amount of Ag NPs increased in the PVA nanofibers, the enhancement factor did not increase accordingly, which indicated that different morphology of Ag NP aggregation had a great influence on the enhancement effects of SERS [116]. When 4-mercaptobenzoic acid (4-MBA) was used as a probing molecule to study the Raman enhancement effects, the Ag/PVA nanofibers showed excellent detection reproducibility (i.e., the average relative standard deviation values of the major Raman peak were less than 0.07). Taking advantage of the same nanoparticle alignment in the polymer nanofibers, Ag nanowires (NWs) were also synthesized and electrospun into PVA nanofibers [122]. The Ag NW/PVA nanofibers showed similar morphology and the NWs were “frozen-up” within the polymer fibers. In addition, the electrospun Ag NW/PVA nanofibers were arranged into different structures and stronger SERS intensities were obtained from the arranged samples [122]. Besides Ag nanostructures, Au nanostructures were also encapsulated into the PVA electrospun nanofibers as SERS substrates [100].张等人。 used a seed-mediated surfactant-directed approach to synthesize Au nanorods (AuNRs), and these Au NRs exhibited good alignment along the axial direction of the nanofibers, which demonstrates that electrospinning is a powerful tool to assemble anisotropic nanorods on a large scale [100]. Ag and Au nanostructures can be co-assembled into the PVA nanofibers [117, 123]. Different SERS effects can be obtained by varying the Au/Ag ratio and the excitation wavelength due to the different activities of Au and Ag nanostructures under different wavelengths [124]. In spite of the different morphologies of Ag and Au nanostructures, both Au/PVA and Ag/PVA composites showed excellent SERS performance.
Electrospun Polymer Nanofibers Decorated with Noble Metal Nanoparticles for Chemical Sensing Based on Electrochemical Techniques
Nowadays, metal nanoparticles (such as Au, Ag, Cu, and Ni) have become widely utilized in electrochemical sensing applications, which can be attributed to their rich electronic properties, high surface area, and excellent chemical stability [125, 126]. Au NPs can decrease the overpotentials of many electroanalytical reactions and maintain the reversibility of redox reactions [41, 127]. The Au NP platform can be used for detection of different kinds of analytes including small molecules such as glucose [128, 129], dopamine [130,131,132,133], bisphenol A [134], toxic chemicals and drugs such as mercury [135,136,137,138], antimony [139], and hydrogen peroxide [140]. Au NPs hold great promise as substrates for designing electrochemical biosensors, which benefit from their ability to provide a stable immobilization of biomolecules retaining their bioactivity, ease of use in chemical synthesis, narrow size distribution, and their convenient labeling of biomolecules [141,142,143]. Furthermore, both Ag and Au NPs have good biocompatibility and large surface area which can help adsorb biomolecules strongly and play an important role in the immobilization of biomolecules [144]. Accordingly, combining Au or Ag NPs with large-surface-area polymer nanofibers, which provide a large loading capacity for nanoparticles, can further enhance the sensitivity of the sensors [145].
Sapountzi et al. decorated the PVA/poly(ethyleneimine) (PEI)/glucose oxidase nanofibers with Au NPs to further improve the conductivity of the mat and used these composites as electrochemical biosensors [146]. However, both PVA and PEI are water soluble polymers and it may weaken the stability of the composite. Therefore, the researchers conducted a post-electrospinning cross-linking step by exposing the NFs to glutaraldehyde (GA) vapors and the morphology of the fibers was still well retained, suggesting a successful chemical cross-linking reaction induced by GA vapors [146]. The same treatment was also performed by other researchers and the cross-linked PVA nanofiber mat maintained its morphology even after being soaked in water for 15 days [99]. After obtaining the water soluble PVA nanofiber mat, 3-mercaptopropyltrimethoxysilanes (MPTES) were first modified on the surface of electrospun PVA nanofibers. Then, the modified PVA nanofiber mat was immersed into the as-prepared Au NPs aqueous solutions and Au NPs were strongly bonded onto the surface of the modified PVA nanofibers due to the strong affinity between the thiol groups and Au NPs [99]. Au NPs were homogenously decorated on the surface of the modified PVA nanofibers for different Au NP concentrations, leading to highly sensitive detection of H2O2 and the Au NPs/modified PVA also showed more advantages such as fast response, broad linear range, and low detection limit [99].
Conclusions
Extensive research has been carried out to study the properties and applications of both Au or Ag nanostructures and electrospun nanofiber materials in recent years. Taking advantage of the flexibility, large surface area, ease of production, and surface modification of the electrospun polymer nanofibers, the combination of Au/Ag nanostructures with nanofibers makes these composites versatile platforms for various applications in optics, antibacterial coatings, photovoltaics, and chemical and biological sensors etc. The adaptable functionalization of both electrospun nanofibers and Au or Ag nanostructures can lead to unique morphologies and structures for Au or Ag nanostructure/electrospun nanofiber composites, followed by more applications with enhanced performance.
Despite the increasing number of publications using electrospun polymer nanofibers decorated with noble metal nanoparticles for sensing applications, the field is in its infancy. The rational integration of noble metal nanoparticles to nanofiber matrices to achieve desirable plasmonic properties will bring unprecedented strategies for sensor development. Further investigations are required to better understand the morphology control, formation mechanism, and applications to specific applications. It is expected that further development of this field will eventually make a wide impact on many areas of research.
缩写
- GA:
-
Glutaraldehyde
- MPTES:
-
3-Mercaptopropyltrimethoxysilane
- NWs:
-
Nanowires
- PAA:
-
Poly (acrylic acid)
- PAN:
-
Polyacrylonitrile
- PEG:
-
Poly(ethylene glycol)
- PEI:
-
Polyethyleneimine
- PEO:
-
Poly (ethylene oxide)
- PVA:
-
Polyvinyl alcohol
- PVdF:
-
Polyvinylidene fluoride
- PVME:
-
Poly(vinyl methyl ether)
- PVP:
-
Polyvinylpropelene
- SERS:
-
Surface-enhanced Raman scattering
- TEM:
-
透射电子显微镜
- XPS:
-
X射线光电子能谱
纳米材料
- 金属 3D 打印的支撑结构——更简单的方法
- ADI:用于生物和化学传感的新型阻抗和恒电位仪 AFE
- ADI:用于生物和化学传感的阻抗和恒电位仪 AFE
- 用于化学传感器的金纳米粒子
- 用于增强药物递送的纳米纤维和细丝
- 用银纳米粒子作为抗菌剂装饰的基于氧化石墨烯的纳米复合材料
- 用于磁热疗的电纺 γ-Fe2O3/聚氨酯纳米纤维的电场辅助原位精确沉积
- 用 MnFe2O4 纳米颗粒装饰的还原氧化石墨烯对四环素的吸附
- 带有 Ag 纳米颗粒的对齐良好的 TiO2 纳米管阵列,用于高效检测 Fe3+ 离子
- 用于生物医学、药物输送和成像应用的磁性功能化纳米粒子
- 具有氧化还原不稳定聚合物壳的聚多巴胺复合纳米颗粒,用于控制药物释放和增强化学光热疗法
- 钣金化学薄膜转化涂层