用于增强维拉帕米细胞摄取的冻干混合纳米结构脂质载体:统计优化和体外评估
摘要
维拉帕米是一种钙通道阻滞剂,对治疗高血压、心绞痛等疾病非常有效。然而,由于首过效应,该药物的生物利用度低,仅为 20% 至 35%。本研究的主要目的是开发混合维拉帕米-葡聚糖纳米结构脂质载体 (HVD-NLC),以尝试增加维拉帕米细胞摄取。采用高剪切均质法成功制备制剂,并采用2 4 进行统计学优化 全析因设计。使用海藻糖作为冷冻保护剂冷冻干燥 HVD-NLC 制剂。结果表明,优化后的配方(VER-9)的粒径(PS)、多分散指数(PDI)和包封率(%EE)分别为192.29 ± 2.98、0.553 ± 0.075和93.26 ±6 % , 分别。在制剂中掺入硫酸葡聚糖延长了维拉帕米在模拟胃液 (pH 1.2) 和模拟肠液 (pH 6.8) 中的释放(48 小时内~ 85%)。差示扫描量热分析显示维拉帕米和制剂中的赋形剂之间没有化学相互作用。虽然广角 X 射线散射研究表明药物在掺入 NLC 后呈无定形形式。透射电子显微镜和扫描电子显微镜图像显示纳米颗粒具有球形形状。使用 Caco-2 细胞系的细胞摄取研究表明,与维拉帕米溶液和维拉帕米-葡聚糖复合物相比,来自 HVD-NLC 的维拉帕米摄取更高。在冷藏条件 (5°C ± 3°C) 中储存的优化配方 (VER-9) 可稳定保存 6 个月。总之,HVD-NLCs是维拉帕米的潜在载体,因为它们显着增强了药物的细胞摄取。
背景
维拉帕米是苯烷基胺类的 L 型钙通道阻断剂,是 α-肾上腺素能受体的拮抗剂。它广泛用于治疗高血压、室上性快速性心律失常、心绞痛和丛集性头痛。根据生物药剂学分类体系,维拉帕米属于I类药物。已知该类药物口服后经肠膜吸收良好(≤ 90%)。然而,由于通过门脉循环进行快速首过代谢,只有 20% 至 35% 的维拉帕米口服剂量进入血液循环 [1]。因此,开发合适的载体以增强维拉帕米的细胞摄取并可能提高其生物利用度具有重要意义。
第二代基于脂质的纳米制剂,如纳米结构脂质载体 (NLC),在治疗药物的递送方面具有巨大的潜力 [2, 3]。与固体脂质纳米颗粒 (SLN) 相比,NLC 具有更高的化学和物理稳定性,并且它们还具有控释特性。此外,NLCs 通过形成较少有序的脂质基质晶格,对药物分子表现出高负载能力,这可以最大限度地减少储存期间药物的排出。 NLC 由脂质制备,可加速肠内乳糜微粒的形成并促进 NLC 的吸收,从而提高药物的生物利用度 [4]。因此,本研究开发了混合维拉帕米-葡聚糖纳米结构脂质载体(HVD-NLCs)以提高药物的细胞摄取,从而提高其生物利用度。
大多数亲脂性药物可以很容易地结合到 NLC 中,但很难将维拉帕米等亲水性药物包裹在基于脂质的纳米制剂中。因此,在本研究中,制备了使用抗衡离子葡聚糖硫酸钠的混合 NLC,以增强维拉帕米的包封并延长其从 NLC 中的释放(图 1)。 HVD-NLCs 配方采用热和高剪切均质法制备,并使用 2 4 进行统计学优化 全析因设计。研究中使用了两种脂质:固体脂质 Compritol 888 ATO® 和液体脂质油酸。制备的 HVD-NLC 具有粒径 (PS)、zeta 电位 (ZP)、多分散指数 (PDI) 和药物包封率 (%EE) 的特征。使用海藻糖作为冷冻保护剂将优化的 HVD-NLC 冻干。体外释放曲线在碱性和酸性环境中进行。使用 Caco-2 细胞系对所选 HVD-NLC 中的维拉帕米进行体外细胞摄取研究。在三种不同条件下(5°C ± 3°C、25°C ± 2°C/60% RH ± 5% RH和40°C ± 2°C/ 75% RH ± 5% RH)。
<图片>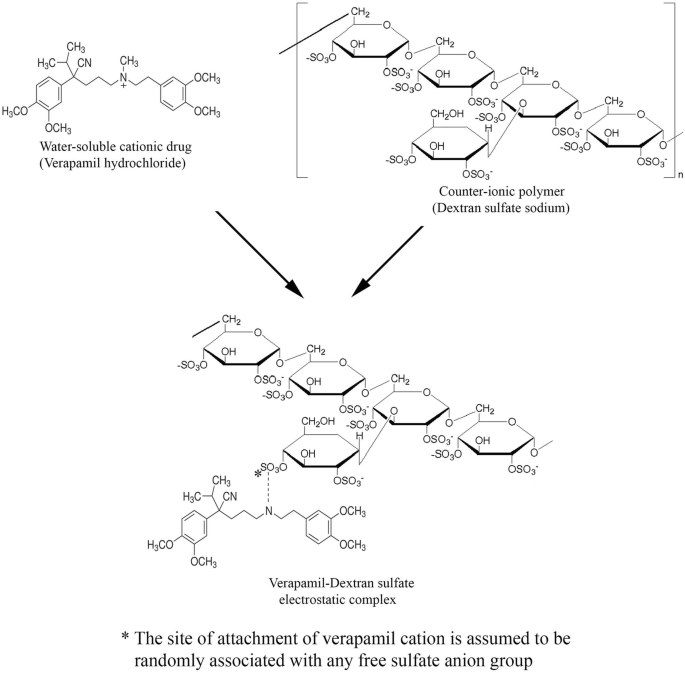
水溶性阳离子药物盐酸维拉帕米与抗衡离子聚合物葡聚糖硫酸钠的静电络合物的形成
图>方法
材料
盐酸维拉帕米购自温州制药厂(中国温州)。 Compritol 888 ATO® (Glycerol dibehenate EP–Glyceryl behenate NF) 购自 Gattefosse (Saint-Priest, France)。油酸购自 R&M 化学品(英国埃塞克斯)。 Tween 80®(聚山梨醇酯 80)购自 Euro chemo-pharma Sdn。 Bhd.(马来西亚槟城)。泊洛沙姆 188 (Pluronic® F-68) 购自 Molekula (Dorset, UK)。海藻糖、Sephadex® G-25 和胎牛血清 (FBS) 购自 Sigma-Aldrich(美国密苏里州圣路易斯)。 Caco-2 细胞系获自 ATCC (Virginia, USA)。青霉素-链霉素溶液 100 X 购自 Biowest (Nuaillé, France)。胰蛋白酶 0.25% 和 Dulbecco 改良 Eagles 培养基 (DMEM) 购自 GE 医疗保健生命科学公司 Hyclone 实验室(美国犹他州)。 Passive Lysis Buffer, 5X 购自 Promega (Wisconsin, USA)。
方法
HVD-NLC 的制备
HVD-NLCs 是通过热高剪切均质法制备的。脂质相由 Compritol ATO 888® 组成,油酸通过在 85°C(比固体脂质的熔点高 10°C)加热而熔化。称取所需量的维拉帕米并溶解在含有 Tween 80® 和泊洛沙姆 188 的混合物的水相中,其比例为 1:1 w /w .将水相加热至与脂质相相同的温度。将水相倒入脂质相中,并使用数字 T25 Ultra-Turrax® 均质器 IKA®(Staufen,德国)在 24,000 rpm、85°C 下均质化。随后,在均质过程中将硫酸葡聚糖水溶液(与维拉帕米的比例为 1:1)缓慢加入混合物中。将制备的 HVD-NLC 在室温(25 ± 2°C)下冷却。
使用2 4 对配方参数进行统计优化 全因子设计和合意性函数方法
执行具有两个水平和四个变量的全因子设计以优化和评估配方自变量(因子)对特征(因变量或响应)的影响(表 1)。在初步研究的基础上选择了每个自变量的真实高低水平。
图>从因变量获得的实验响应是四个自变量的单独和组合效应的结果。这种两水平的实验设计提供了足够的数据来拟合以下多项式方程。
$$ X={\upbeta}_0+{\upbeta}_1A+{\upbeta}_2B+{\upbeta}_2B+{\upbeta}_3C+\upbeta 4D+{\upbeta}_{12} AB+{\upbeta}_{13}\mathrm{AC}+ {\upbeta}_{14}\ \mathrm{AD}+{\upbeta}_{23}\mathrm{BC}+{\upbeta}_{24}\mathrm{BD}+{\upbeta}_{34 }\mathrm{CD} $$ (1)其中 X 是一个因变量; β0 是截距; β1、β2、β3、β4是自变量A的系数 , B , C , 和 D 分别。而 β12、β13、β14、β23、β24 和 β34 是各自的相互作用(即 AB、AC、AD、BC、BD 和 CD)。实验结果通过 Design-Expert® 软件 6.0.10 版(Stat-Ease, Inc., Minneapolis, MN, USA)进行分析,然后进行方差分析 (ANOVA),以确定因素及其相互作用的显着性。当 p 值<0.05。
最终配方是根据合意性函数方法选择的。合意性函数将所有响应组合为一个变量,以预测所研究因素的最佳水平。因此,确定需要选择具有最低粒度 (PS) 和多分散指数 (PDI) 以及最高 zeta 电位 (ZP) 和百分比截留率 (%EE) 值的优化配方。合意性函数将所有响应转换为一个共同的尺度 (0, 1) 并通过几何平均将它们组合起来以优化整体度量。合意性值 1 表示响应的可接受值(最期望值),而合意性值 0 表示不可接受值。
粒度、多分散指数和 Zeta 电位的测量
使用光子相关光谱仪(Zetasizer 1000HS/3000HS,Malvern Instrument,Malvern,UK)测量制备的纳米颗粒的 PS 和 PDI。 ZP 使用 zeta 电位分析仪(Zetasizer nano 系列,Nano Z-red 徽章,Malvern Instrument,Malvern,UK)测定。使用过滤的蒸馏水(0.45 μm 尼龙过滤器)稀释所有样品,并以一式三份的方式进行测量。
捕获效率百分比的确定
使用 Sephadex® G25 通过微型柱离心方法分离含有游离维拉帕米-葡聚糖复合物和 HVD-NLC 的制备样品。将 1 mL 样品置于柱顶,然后以 2000 rpm 离心 2 分钟。收集含有 HVD-NLC 的洗脱液并使用冷冻干燥机(Labconco,Free Zone Freeze Dry Systems, Kansas City, USA)冻干。
将冻干的 HVD-NLC(10 毫克)溶解在氯仿中并在 40°C 的氮气下蒸发。然后将干燥的样品溶解在 1 mL 蒸馏水中,涡旋 2 分钟,并以 12,000 rpm 离心(Eppendorf,德国)5 分钟。收集上清液并使用经过验证的高效液相色谱 (HPLC) 方法测定维拉帕米的含量。 %EE 使用以下公式计算:
$$ \%\mathrm{EE}=\frac{\mathrm{Weight}\ \mathrm{of}\ \mathrm{encapsulated}\ \mathrm{verapamil}}{\mathrm{Initial}\ \mathrm{weight}\ \mathrm{of}\ \mathrm{维拉帕米尔}}\kern0.5em \times \kern0.75em 100 $$ (2)使用配备有 LC 20AD 输送泵(日本京都)和 Phenomenex C18 柱(250 × 4.6 毫米)的岛津色谱系统进行维拉帕米定量的 HPLC 分析。流动相由比例为 40:60 (v /v )。用冰醋酸将流动相的 pH 值调至 6.5。进样体积为 20 μL,维拉帕米的检测波长设置为 278 nm,流速固定为 1.5 mL/min。
冻干研究
使用 Labconco,FreeZone Freeze Dry Systems(美国堪萨斯城)在 - 40°C 下进行冻干 24 小时。筛选了五种常用的糖类,包括甘露醇、果糖、蔗糖、乳糖和海藻糖,脂质与冷冻保护剂的比例为 1:1,以选择合适的冷冻保护剂用于 HVD-NLC 的冷冻干燥。选择在蒸馏水中复溶后冻干制剂产生最低平均 PS 和 PDI 的冷冻保护剂进行进一步研究。
在接下来的研究中,使用两种方法将 HVD-NLC 制剂与选定的冷冻保护剂混合。第一种方法是在制备 HVD-NLCs 制剂后加入冷冻保护剂,称为“均质后处理”。而第二种方法中,冷冻保护剂是在制备HVD-NLCs制剂的过程中加入的,称为“均质过程中”。在第一种方法中,制备并冻干了几种比例为 1:1、1:2、1:4、1:6 和 1:8 的脂质和冷冻保护剂。将冻干的 HVD-NLC 制剂重新分散在过滤的蒸馏水(0.45 μm 尼龙过滤器)中,并评估样品的平均 PS、PDI 和 %EE。选择产生最低 PS 和 PDI 以及最高 %EE 的脂质:冷冻保护剂比率用于第二种混合方法的进一步研究。
体外释放研究
维拉帕米从 NLC 的体外释放研究是使用置于模拟肠液 (pH 6.8) 或模拟胃液 (pH 1.2) 介质中的透析袋进行的。在这项研究中,将 2 mL HVD-NLCs 分散体装入透析袋(12,000 Da MW 截止值)。将袋子密封,然后浸入 150 mL 预热的释放介质中。释放研究在设置为 100 rpm 搅拌速度和 37°C 的热板磁力搅拌器上进行。在 0.08、0.25、0.5、1、2、4、6、8、10、12、24、48 和 72 小时的预定时间间隔内,抽取 1 mL 样品并更换为相同体积的新鲜培养基。 HPLC测定释放的维拉帕米浓度。
差示扫描量热法
使用 Perkin-Elmer Pyris 6 DSC (Perkin-Elmer, Beaconsfield, UK) 进行差示扫描量热法 (DSC) 分析。将样品称重(5-7 毫克)放入铝盘中并使用标准样品盘压接机(Perkin-Elmer,Beaconsfield,UK)密封。从 0 到 260°C,以 10°C/min 的速率记录 DSC 曲线。
广角 X 射线散射
广角 X 射线散射 (WAXS) θ/2θ 分析是在室温下使用帕纳科 X’Pert PRO MRD PW3040(荷兰阿尔梅洛)应用 Cu Kα 辐射进行的。样品在 2-60°C 的温度范围内以 5°C/min 的扫描速率运行。
透射电子显微镜和扫描电子显微镜
使用(Philips CM12,Eindhoven,Netherlands)通过透射电子显微镜(TEM)观察 HVD-NLC 的形态(即形状和大小)。将一滴稀释的 HVD-NLC 分散体放在 400 目铜网上,然后用 2% 磷钨酸进行负染色。制备的样品在进行透射电镜检测前风干。
使用扫描电子显微镜 (SEM) (Leo Supra 50 VP field Emission SEM, Oberkochen, Germany) 观察冻干 HVD-NLC 的形态。将冻干的 HVD-NLC 样品固定在铝棒上,然后在溅射装置中以 15 mA 的电流涂覆金(10 nm 厚)15 分钟。使用牛津INCA能量色散X射线微量分析系统以10 kV的加速电压对样品进行可视化。
HVD-NLC 的细胞摄取
使用 Caco-2 细胞系研究了来自 HVD-NLC 制剂的维拉帕米的体外细胞摄取研究。细胞在 T-25 组织培养瓶中在补充有 1% 青霉素-链霉素溶液和 10% FBS 的 DMEM 生长培养基中培养。然后,收集 Caco-2 细胞并接种在 48 孔板中,每孔 60,000 个细胞密度,使用 DMEM 完全培养基,直到细胞融合并在孔板底部形成单层。通过仅用 DMEM 培养基稀释 HVD-NLC、维拉帕米溶液和维拉帕米-葡聚糖复合物,制备了几种含有相同浓度维拉帕米的溶液。维拉帕米溶液和维拉帕米-葡聚糖复合物用作对照。随后,将 48 孔板中 Caco-2 细胞单层的 DMEM 完全培养基与不同稀释度的 HVD-NLC、维拉帕米溶液和维拉帕米-葡聚糖复合物交换,并在 37°C 下孵育 6 小时。孵育期结束后,从孔中取出 HVD-NLC、维拉帕米溶液和维拉帕米-葡聚糖复合物样品。通过用磷酸盐缓冲盐水 (PBS) 洗涤 3 次,将测试样品的残留物和死细胞从单层中除去。通过向每个样品孔中加入被动裂解缓冲液 (200 μL) 来裂解单层细胞。移出样品并以 12,000 rpm 离心 10 分钟以从细胞中提取维拉帕米。收集上清液,采用高效液相色谱法测定维拉帕米浓度。
短期稳定性研究
根据国际协调人用药物技术要求委员会 (ICH) 指南 Q1A (R2) 进行了优化 HVD-NLC 配方的短期稳定性研究。将新鲜制备的冻干 HVD-NLC 制剂 (VER-9) 置于三种不同条件下(5 ± 3°C、25 ± 2°C/60 ± 5% 相对湿度 (RH) 和 40 ± 2°C/75 ± 5%RH) 6 个月。通过在 0、1、3 和 6 个月时测量 PS、PDI、ZP 和总药物含量来检查优化的 HVD-NLC 制剂的稳定性。
统计分析
使用单向方差分析 (ANOVA) 分析结果,然后使用事后 Tukey-HSD。使用 IBM® SPSS® Statistical 软件(第 22 版,纽约,美国)进行统计分析。所有值均表示为平均值和标准偏差(平均值 ± SD)。当 p 时,差异具有统计学意义 <0.05.
结果与讨论
使用2 4 分析 全因子设计
所选自变量的值,即均质化时间 (A)、固体脂质浓度 (B)、液体脂质浓度 (C) 和表面活性剂浓度 (D) 以及所选响应的所得结果,即 PS、PDI 、ZP 和 %EE 显示在表 2 中。使用方差分析对每个自变量及其相互作用进行统计评估。每个因变量的多项式系数由 Design-Expert® 软件生成,以量化每个自变量的影响,如方程式 1 所示。 3, 4, 5, 6。方程只代表了显着因素及其相互作用。
图> $$ \mathrm{PS}=185.73-7.64A-2.13C-12.03D-2.15\mathrm{AC}+2.16\mathrm{BD}-3.53\mathrm{CD} $$ (3) $$ \mathrm{PDI }=0.48-0.060C-0.029\mathrm{AC}+0.039\mathrm{CD} $$ (4) $$ \mathrm{ZP}=-45.94-2.06C-1.41D+0.91\mathrm{BD}-1.09 \mathrm{CD} $$ (5) $$ \%\mathrm{EE}=81.40-4.31A+8.45D-2.13\mathrm{AD}+1.90\mathrm{BD} $$ (6)其中 A 是均质化时间(分钟),B 是固体脂质浓度(mg),C 是液体脂质浓度(mg),D 是表面活性剂浓度(mg)。方程中每个因素或相互作用因素前的正负号分别代表协同效应或拮抗效应。生成响应面图以观察各因子或因子交互作用对响应参数的并发影响。
变量对平均粒径的影响
如方程式所示。 3、因素A , C , 和 D 对粒径 (PS) 有拮抗作用。可以得出结论,增加均质时间(因子A ) 同时与 NLC 的 PS 显着降低相关 (p <0.05)。这可能是由于均质的高剪切力有效地将脂质球破碎成更小的颗粒。同样,增加液体脂质浓度(C ) 被发现同时降低 NLC 的 PS。这可能归因于油酸在与 Compritol 888 ATO® 结合时降低系统粘度和表面张力的能力,从而产生更小粒径的 NLC。 Jenning 等人也报道了液体脂质在与固体脂质结合时降低系统粘度的能力。当他们制备 SLN 分散体时 [5]。作者观察到,将脂质基质组合物中的液体脂质浓度从 0% 增加到 38% 会减小粒径。此外,增加表面活性剂浓度 (D ) 会降低 PS,因为系统中存在较高量的表面活性剂很容易乳化制剂中的总脂质含量,从而形成更小的纳米颗粒。
图 2 显示了显着 (p <0.05) 因素(AC、BD 和 CD)相互作用对 NLC 的 PS 的影响。因子 (BD) 的积极影响和因子 (AC 和 CD 相互作用) 的负面影响对 NLC 粒径有显着影响。因子 (AC) 的负面影响表明,在较长的均质时间和较高的液体脂质浓度下,PS 会降低。 NLC 制剂中较高浓度的固体脂质和表面活性剂之间的相互作用(BD 相互作用)显示出对粒径的显着积极影响,从而导致更大的粒径。相比之下,较高浓度的液体脂质和表面活性剂之间的相互作用(CD 相互作用)对产生较小尺寸的颗粒尺寸有显着的负面影响。
<图片>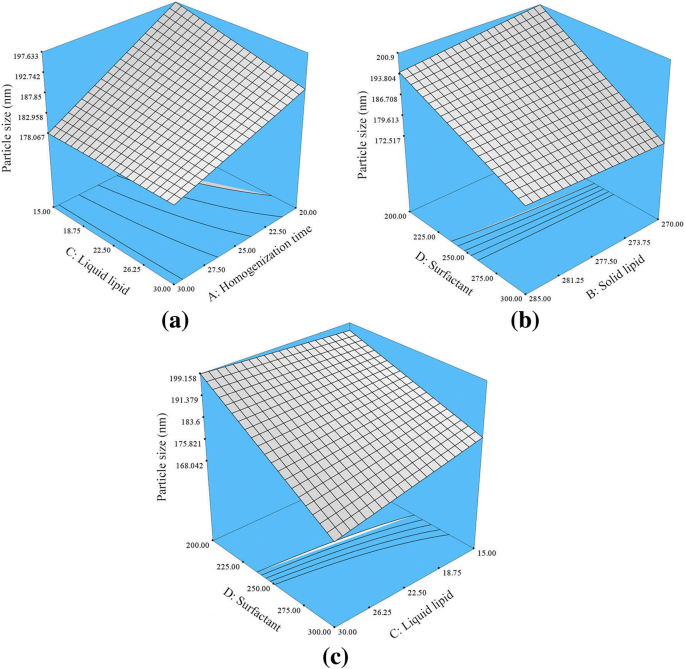
描述显着性的响应面图 (p <0.05) 均质化时间、液体脂质浓度、表面活性剂浓度以及a之间相互作用的影响 交流电,b BD 和 c HVD-NLC 的 PS 上的 CD。 A 均质化时间,B 固体脂质,C 液体脂质,D 表面活性剂
图>变量对 PDI 的影响
如方程式所示。如图 4 所示,油酸对 PDI 有负面影响,这意味着增加油酸浓度会降低 PDI 值并导致 NLC 颗粒的更好分布。一些研究也观察到油酸对 NLC 的类似作用 [6]。图 3 描绘了匀浆时间和液体脂质浓度之间的相互作用(AC 相互作用)。这种相互作用对 PDI 显示出显着的负面影响。这意味着更长的均质时间和更高的液体脂质浓度将导致 PDI 值的降低。此外,液体脂质和表面活性剂浓度之间的相互作用(CD 相互作用)对 PDI 值显示出协同作用。因此,同时增加液体脂质和表面活性剂的浓度会增加 PDI 值并产生较不均匀的 HVD-NLC 颗粒。这可能是由于表面活性剂浓度进一步增加超过优化值可能导致表面活性剂在纳米粒子的外表面积累,从而增加 PDI 值。 Shamma 等人也观察到了相同的模式,即增加表面活性剂浓度会导致 NLC 的 PDI 值增加 [7]。
<图片>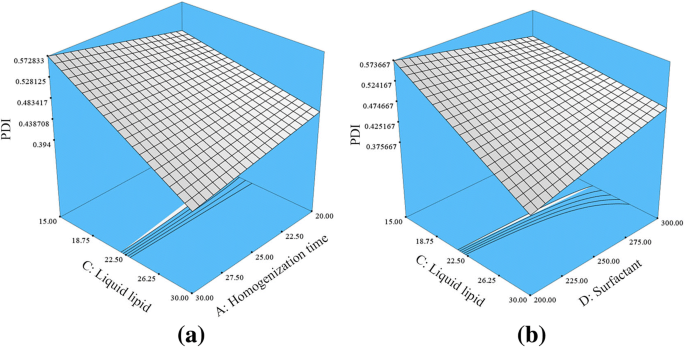
描述显着性 (p <0.05) 液体脂质浓度的影响和a之间的相互作用 AC和b HVD-NLC 的 PDI 上的 CD。 A 均质化时间,C 液体脂质,D 表面活性剂
图>变量对 ZP 的影响
由于山嵛酸甘油酯(Compritol 888 ATO® 中的一种脂肪酸)、油酸和硫酸葡聚糖残留物的电离,HVD-NLC 制剂带有负电荷。基于方程。 5,增加油酸的量对纳米颗粒有负面影响,导致纳米颗粒的负电荷增加,从而增加 HVD-NLC 的 ZP。这可能是由于油酸(液体脂质)中丰富的羧基过度电离 [8]。此外,山嵛酸甘油酯(固体脂质)也对 ZP 值有负面影响,增加其浓度会增加纳米颗粒的 ZP。这是由于二十二酸甘油酯中羧酸的电离度较高,导致纳米颗粒外表面带较高的负电荷。
BD 相互作用对 NLC 的 ZP 具有显着的积极影响,而 CD 相互作用则显示出负面影响(图 4)。增加固体脂质的浓度和降低表面活性剂的浓度会降低纳米颗粒的负电荷并降低 ZP 值。可能在这个阶段,较低的表面活性剂浓度将无法电离固体脂质的总量,导致纳米颗粒表面的负电荷减少,从而降低 HVD-NLC 的 ZP。此外,同时增加液体脂质和表面活性剂(CD 相互作用)的浓度会显着增加 HVD-NLC 的 ZP 值。这是因为配方中表面活性剂浓度越高,就会有越多的羧酸被电离。
<图片>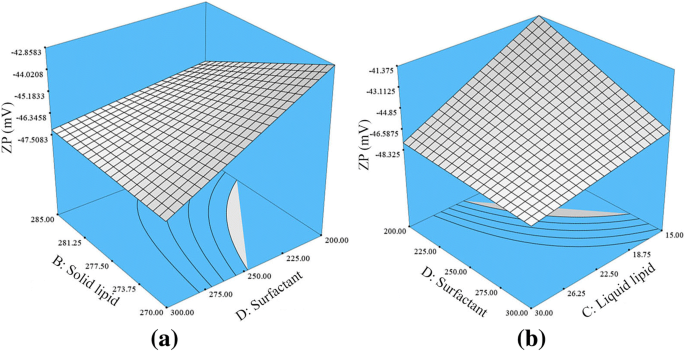
描述显着性 (p <0.05) 液体脂质浓度、表面活性剂浓度以及a之间相互作用的影响 BD 和 b HVD-NLC 的 ZP 上的 CD。 B 固体脂质,C 液体脂质,D 表面活性剂
图>变量对 %EE 的影响
方程 6 表明,增加均质时间会显着 (p <0.05) 降低药物的 %EE。更长的匀浆时间可以剥离或剥离附着在纳米颗粒外表面的药物分子,这导致 HVD-NLC 中药物的 %EE 较低。相反,增加表面活性剂浓度会导致 HVD-NLC 中药物的 EE 百分比升高。这可能是因为较高的表面活性剂浓度会增加 NLC 的外表面涂层,部分药物会被包裹在其中。庄等人。和达斯等人。表明足够浓度的表面活性剂对于脂质晶格内部和纳米颗粒外表面的药物分子的溶解至关重要[9, 10]。
如图 5 所示,AD 交互作用显着 (p <0.05) 负面影响,由此增加均质化时间和减少表面活性剂的量会降低 HVD-NLC 中药物的 %EE。相比之下,固体脂质和表面活性剂浓度之间的相互作用(BD 相互作用)显示出对药物 %EE 的积极影响。因此,增加固体脂质和表面活性剂的浓度将增加 HVD-NLC 中药物的 %EE。这可能是由于较高的表面活性剂浓度会溶解过量的药物分子,同时系统中存在的较高量的固体脂质容纳了过量的药物分子,因此增加了药物在 HVD 中的 %EE-国家图书馆。
<图片>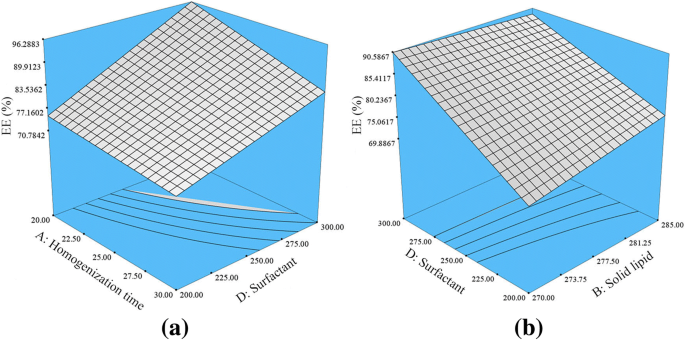
描述显着性 (p <0.05) 均质化时间、液体脂质浓度、表面活性剂浓度以及a之间相互作用的影响 AD和b BD 在 HVD-NLC 中维拉帕米的 EE。 A 均质化时间,B 固体脂质,D 表面活性剂
图>使用合意性函数选择优化的 HVD-NLC
代码编号为 VER-9、VER-11、VER-13 和 VER-15 的 HVD-NLC 公式分别具有更高的合意性值,分别为 0.863、0.852、0.811 和 0.840。选择这些配方进行进一步研究,因为合意性值更接近 1。所选配方的所有响应的个体和组合合意性函数如图 6 所示。这些的平均 PS、PDI、ZP 和 %EE制剂为在173.46±0.757的范围内以±190.3 8.22,0.451±0.033至0.501±0.019, - 46.73±1.13到 - 49.16±1.55,和93.95±4.10 98.81到1.01±分别
。 <图片>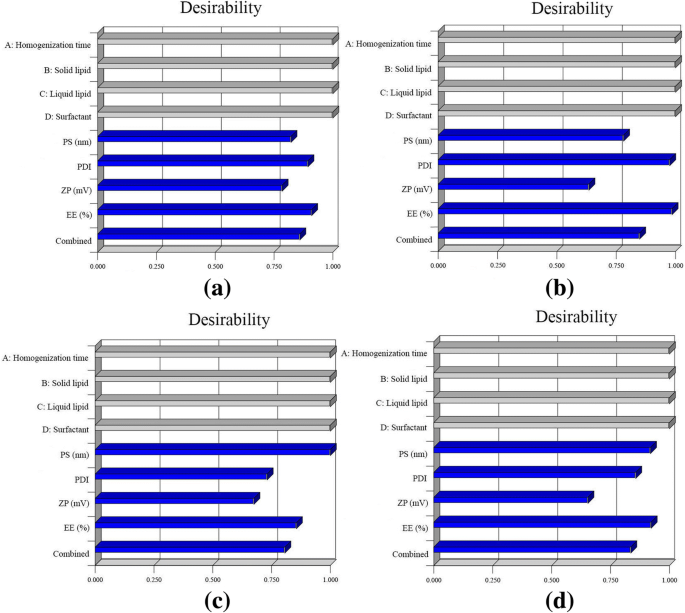
Bar graph displaying the individual and combined desirability of several responses on selected HVD-NLCs formulations. 一 VER-9, b VER-11, c VER-13, and d VER-15
图>Lyophilization Study
Lyophilization is one of the commonly used methods in the pharmaceutical field to change aqueous formulation into the dried form to prolong the physical and chemical stability of the nanoparticle during storage [11, 12]. However, the lyophilization step produces various stresses to the sample during the process. So, the need of cryoprotectant is essential to avoid the stress condition and protect the sample. Among the screened cryoprotectants, trehalose was found to be the most suitable one for protecting the HVD-NLCs formulations from aggregation after the lyophilization process. The HVD-NLCs formulations protected with trehalose had the smallest particle size and lowest PDI (Table 3). Trehalose offers many advantages over other cryoprotectants including less chemical interactions and exhibit higher glass transition temperature which may help to prolong the stability of nanoparticles. Furthermore, trehalose has been demonstrated as an efficient cryoprotectant for freeze drying of Compritol 888 ATO® (Glyceryl behenate) in the lipid-based nanoparticles [13, 14]. Thus, in the present study, trehalose was selected as cryoprotectant for the HVD-NLCs formulations.
图>In the next study, different ratios of trehalose were used in the HVD-NLCs formulations after or during the homogenization process. Table 4 shows the effects of different ratios of trehalose added into the formulations after homogenization on PS, PDI, and %EE.
图>The mean PS was found in the nano size range, while PDI was < 1 in all ratios of lipids to trehalose used in the study. However, the %EE of verapamil was decreased significantly with increasing the ratio of trehalose to lipids. The %EE of lipid:trehalose at the ratio of 1:1 was significantly higher than the lipid:trehalose ratio of> 1:1. Increasing the amount of trehalose beyond the lipid trehalose ratio of 1:1 enhanced the removal of verapamil from the verapamil-dextran sulfate complex. This is possibly due to the interaction between trehalose and dextran at the outer surface of nanoparticles, which promotes the displacement of verapamil from the verapamil-dextran sulfate complex and hence, lowers the %EE of drug in the HVD-NLCs. The interaction between trehalose and dextran takes place due to the formation of sugar-salt complex (trehalose-dextran complex), in that the trehalose (sugar) competes with cationic verapamil and forms complex with polyanionic dextran [15]. Miller et al. measured the viscosity and glass transition temperature of trehalose with boric acid and proposed the formation of chemical complex between trehalose and borate ion (B(OH)4 − ) [15]. Therefore, based on the obtained results, the lipid to trehalose ratios of 1:1 and 1:2 were selected for the next study of trehalose addition during homogenization. Table 5 shows that the results of PS, PDI, and %EE were significantly better when trehalose were added during homogenization. This could be due to better mixing of trehalose in the HVD-NLCs formulation by the homogenization process. It is evident from the data that there was no significant difference in the %EE of lipid:trehalose ratios of 1:1 and 1:2. Therefore, formulation VER-9 and VER-11 at lipid:trehalose ratio of 1:1 was selected for further studies.
图>In Vitro Release of HVD-NLCs
The release profile of verapamil solution from the selected HVD-NLCs formulations (VER-9 and VER-11) in SGF and SIF are shown in Fig. 7. The release profile of verapamil from the VER-9 and VER-11 formulations was significantly longer than verapamil solution in both SGF and SIF media. Verapamil was loaded as an ionic complex with dextran sulfate in the HVD-NLCs formulation. This could be the reason for the sustained release of verapamil from the HVD-NLCS. The formulations released about ~ 85% of verapamil after 48 h and the drug had a prolonged release profile up to 72 h. On the other hand, the verapamil solution released approximately 99% of verapamil within 4 h. The release profiles of the selected formulations VER-9 and VER-11 were found almost similar in SGF and SIF release media. However, the release of verapamil from VER-9 was found slightly better as compared to VER-11 in the SGF release medium. The percentage cumulative release of verapamil at 72 h from VER-9 (95.91 ± 1.40) was found significantly higher as compared to VER-11 (90.67 ± 1.16) in the SGF medium. Therefore, VER-9 was selected as a final formulation and proceeded for further investigations.
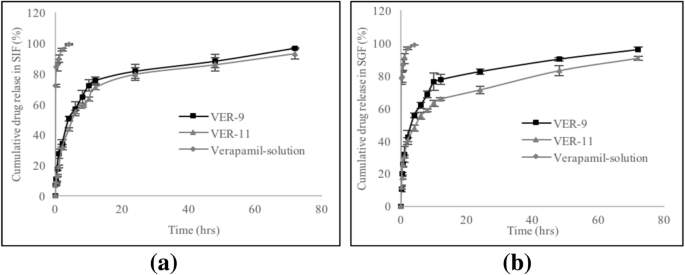
Cumulative percentage of verapamil released in a SIF (pH 6.8) and b SGF (pH 1.2) release media for 72 h
图>DSC Study
The DSC analysis of verapamil, dextran sulfate, Compritol 888 ATO®, poloxamer 188, trehalose, physical mixture (including Compritol 888 ATO®, poloxamer 188, trehalose, verapamil, and dextran sulfate), lyophilized blank formulation (Placebo), and lyophilized HVD-NLCs formulation (VER-9) are shown in Fig. 8. Verapamil, dextran sulfate, Compritol 888 ATO®, poloxamer 188, and trehalose had the melting peak at 144.96 °C, 220.41 °C, 72.46 °C, 50.80 °C, and 100.13 °C, respectively. The thermograms showed that there was no significant shift in the peaks of physical mixture, lyophilized blank formulation, and HVD-NLCs formulation (VER-9) when compared to the individual peak components such as Compritol 888 ATO®, poloxamer 188, trehalose, verapamil, and dextran sulfate. This indicated the physical compatibility between the components. The endothermic peak of verapamil at 144.96 °C was no longer seen in the thermogram of HVD-NLCs formulation (VER-9) (Fig. 8f), which could be due to verapamil that had changed to amorphous form when incorporated in the HVD-NLCs matrix [16].
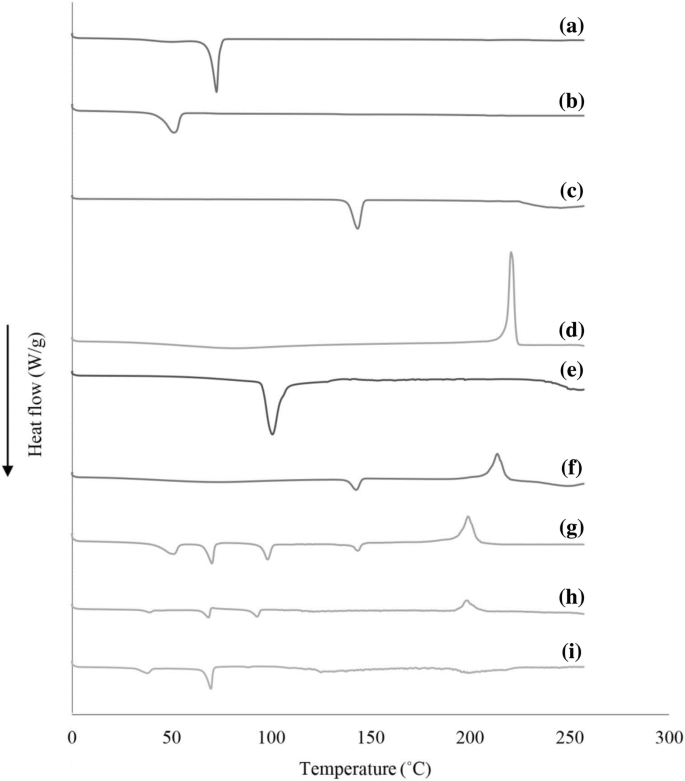
DSC thermograms of a Compritol 888 ATO, b Polomxamer 188, c verapamil HCl, d dextran sulfate, e trehalose, f verapamil HCl and dextran sulfate physical mixture, g physical mixture (including Compritol 888 ATO, poloxamer 188, trehalose, verapamil, and dextran sulfate), h placebo, and i HVD-NLCs formulation (VER-9)
图>WAXS Study
The WAXS/2θ scans of pure verapamil, dextran sulfate, bulk Compritol 888 ATO®, trehalose, lyophilized blank formulation (containing Compritol 888 ATO®, oleic acid, poloxamer 188, Tween 80®, trehalose, and dextran sulfate), and lyophilized HVD-NLCs formulation (VER-9) are shown in Fig. 9. The diffraction peaks of pure verapamil showed specific crystalline nature of the drug at 2θ scattered angles 14.42°, 16.67°, 17.07°, 18.07°, 18.82°, 20.22°, 23.77°, and 26.27° [17]. However, the WAXS/2θ scans of HVD-NLCs formulation (VER-9) showed that the peaks of verapamil diminished indicating a decrease in the crystallinity of the drug when incorporated in the lipids of HVD-NLCs. The scan also showed the predominant peaks at 2θ scattered angles 12.47°, 15.17°, 16.87°, 21.07°, and 23.82° which possibly attributed to the crystalline structure of Compritol 888 ATO® and trehalose because none of these peaks showed similarity with any of the pure verapamil peaks [10]. The results suggested that verapamil was in the amorphous form when incorporated in the HVD-NLCs formulation. The Compritol 888 ATO® revealed polymorphic crystalline transformation upon heating, whereby it changed to a more stable form, and showed reduced intensity peaks at 21.1° and 23.3° in the HVD-NLCs and blank formulations. The WAXS pattern of pure dextran sulfate indicated its amorphous form.
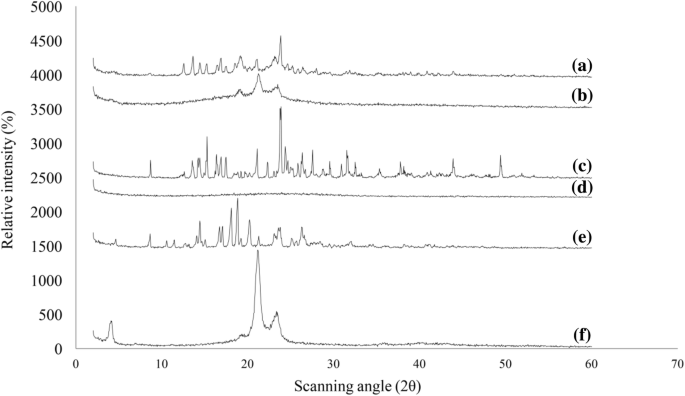
X-ray patterns of a lyophilized HVD-NLCs formulation (VER-9), b lyophilized blank formulation, c trehalose, d dextran sulfate, e verapamil HCl, and f Compritol 888 ATO
图>Electron Microscopic Examinations
The particle shape and size of the HVD-NLCs formulation (VER-9) were examined using the electron microscope. The liquid preparation of HVD-NLCs formulation (VER-9) observed under transmission electron microscope (TEM) showed that the particles had a spherical shape with the size less than 200 nm (Fig. 10). In contrast, observation under scanning electron microscope (SEM) revealed that the nanoparticles no longer had a spherical shape following lyophilization of the formulation without trehalose. However, the cryoprotective effect of trehalose could be seen when it was incorporated in the HVD-NLCs formulation (VER-9). The SEM image indicated that the trehalose helped to protect the structure of the nanoparticle to such extent when compared to the lyophilized formulation without the cryoprotectant (Fig. 11).
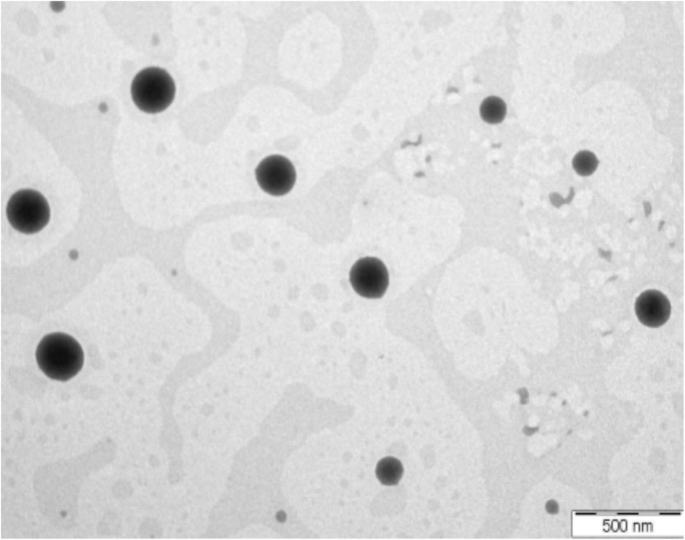
TEM image of VER-9 before lyophilization
图>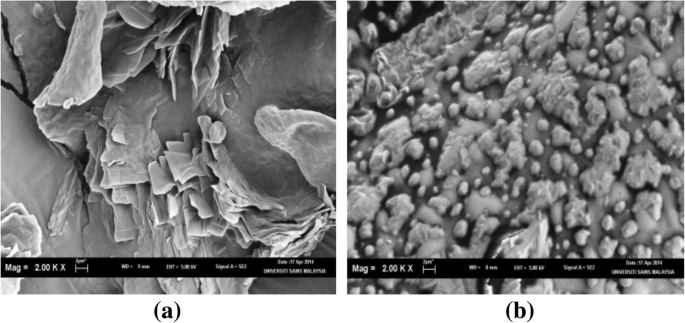
SEM images of VER-9 formulation a without trehalose; b with trehalose
图>Cellular Uptake Study of HVD-NLCs
Caco-2 cells have been extensively used to study drugs intestinal cellular uptake [18]. The Caco-2 cells lines is spontaneously differentiating to form monolayer which has significant morphological and biological resemblance to the intestinal epithelium [19]. Several researchers have reported the in vitro cytotoxicity studies of Compritol ATO 888® [20], oleic acid [21, 22], Tween 80® [23, 24], and poloxamer 188 [25]. These substances were used as excipients in the optimized formulation of HVD-NLCs (VER-9). Based on their finding, these excipients were safe within the concentration used, the cell line type, and cell density that have been employed in the present study. The concentration of verapamil in the samples of VER-9, verapamil solution, and verapamil-dextran complex used in the cellular uptake study was in the range of 10.03 μg/200 μL to 30.1 μg/200 μL. It was found that the verapamil cellular uptake was increased with increasing the verapamil concentration in the samples. The cellular uptake values of verapamil from VER-9, verapamil solution, and verapamil-dextran complex were increased from 6.45 ± 2.62 to 15.92 ± 4.78 μg, 0.89 ± 0.28 to 1.46 ± 0.65 μg, and 0.064 ± 0.019 to 0.118 ± 0.003 μg, respectively (Fig. 12). The verapamil cellular uptake from the VER-9 formulation was 10.90- and 134.91-fold higher than the verapamil solution and verapamil-dextran complex, respectively. The results indicated that verapamil was passively transported from HVD-NLCs, verapamil solution, and verapamil-dextran complex in the caco2-cells. A similar finding of higher cellular uptake of drugs from a lipid-based nanoformulation was reported [26].
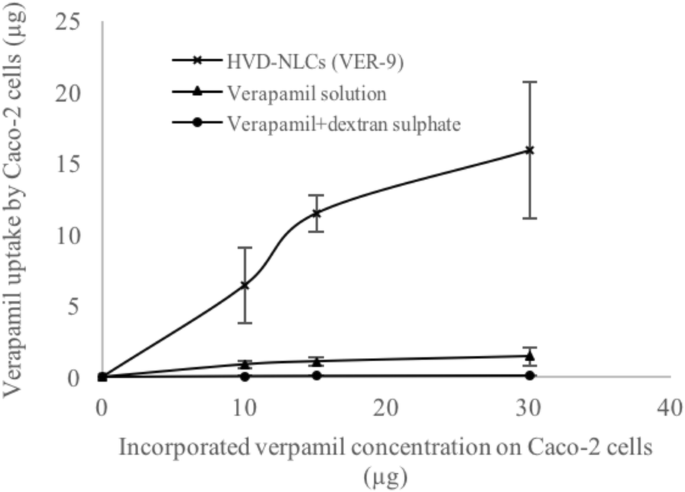
In vitro model of Caco-2 cell line showing cellular uptake of HVD-NLCs (VER-9), verapamil solution, and verapamil-dextran complex (mean ± SD, n = 3)
图>Caco-2 cells act similarly as cell in animals when stimulated by fatty acid. van Greevenbroek et al. suggested that the incorporation of long-chain unsaturated fatty acids (e.g., oleic acid and linoleic acid) into the Caco-2 cells stimulate the secretion of very low-density lipoproteins (VLDL) and chylomicrons. In contrast, administration of saturated fatty acids primarily secretes intermediate- and low-density lipoproteins (IDL and LDL) [27]. Similar findings of increasing chylomicron secretion by the long-chain fatty acids were also reported by Caliph et al. in animal model [28]. In the present investigation, the long-chain fatty acids (Compritol 888 ATO® and oleic acid) used in the preparation of the HVD-NLCs may also stimulate the secretion of chylomicron, and hence increase the cellular uptake of the model drug.
Stability Study
The lyophilized VER-9 formulation was found stable for 6 months at refrigerated condition (5 ± 3 °C) (Table 6). There were no significant differences in the PS, PDI, ZP, and %EE after 6 months of stability study at storage temperature of 5 ± 3 °C. However, the VER-9 formulation was not stable after 1-month storage at the temperatures of 25 ± 2 °C/60 ± 5%RH and 40 ± 2 °C/75 ± 5% RH. The PS and PDI were increased significantly, while the total drug content decreased significantly. After 1 month of stability study, the samples were not measurable due to aggregation and sticky behavior of the nanoparticles.
图>Conclusion
The lyophilized HVD-NLCs formulation was successfully developed and optimized. The lipid carriers had prolonged the release of verapamil from the HVD-NLCs formulation. The formulation was in the nano size range and stable for 6 months at 5 ± 3 °C. The cellular uptake of verapamil lipid formulation (NLCs) was found higher than the non-lipid formulations. This suggested that the NLCs as a lipid carrier for verapamil may play an important role in improving the absorption of the drug.
缩写
- %EE:
-
Percentage of entrapment efficiency
- DMEM:
-
Dulbecco’s modified Eagles medium
- DSC:
-
差示扫描量热法
- FBS:
-
胎牛血清
- HVD-NLCs:
-
Hybrid verapamil-dextran nanostructured lipid carriers
- ICH:
-
The international council for harmonization of technical requirements for pharmaceuticals for human
- IDL:
-
Intermediate density lipoproteins
- LDL:
-
Low density lipoproteins
- NLCs:
-
Nanostructured lipid carriers
- PBS:
-
磷酸盐缓冲盐水
- PDI:
-
Polydispersity index
- 附注:
-
Particle size
- RH:
-
Relative humidity
- SEM:
-
扫描电子显微镜
- TEM:
-
透射电子显微镜
- VLDL:
-
Very low-density lipoproteins
- WAXS:
-
Wide-angle X-ray scattering
- ZP:
-
Zeta potential
纳米材料
- 混合云环境:最佳应用、工作负载和策略指南
- 蜂窝物联网和蓝牙 LE 的协同作用
- 结合蜂窝和 Wi-Fi 以提高成本、连接性和可靠性——网络研讨会
- 通过溶胶-凝胶工艺制备的纳米结构二氧化硅/金-纤维素-键合氨基-POSS 混合复合材料
- 石墨烯和氧化石墨烯的体外和体内生物安全和抗菌能力
- 通过多元醇介导工艺制备和表征 ZnO 纳米夹
- 用于新型隐球菌诊断和光热治疗的抗体偶联二氧化硅修饰金纳米棒:体外实验
- 生长用于形状选择性细胞摄取的金纳米结构
- 制备聚酰胺 6/66 纳米纤维束的溶胶-凝胶静电纺丝工艺条件的统计优化
- 碳纳米管及其衍生物对体外肿瘤细胞和生化参数、体内细胞血液成分的影响
- 告别点点滴滴,增强光存储介质
- 了解混合制造的优势和挑战