深入了解纳米颗粒的细胞摄取和细胞内运输
摘要
纳米粒子科学正在迅速改变各个科学领域的格局并定义新的技术平台。这在纳米医学领域可能更为明显,纳米粒子已被用作治疗和诊断许多疾病的工具。然而,尽管赋予了巨大的好处,但这项技术的常见缺陷是它对人体的潜在短期和长期影响。为了理解这些问题,进行了许多科学研究。本综述试图阐明其中一些研究及其结果。本综述中研究的主题包括纳米颗粒的不同可能摄取途径和细胞内运输途径。此外,还讨论了纳米粒子的物理化学性质,如大小、形状、电荷和表面化学对决定纳米粒子吸收机制和生物学功能的影响。
介绍
纳米粒子 (NPs) 是纳米材料的一个子类别,由于其独特的性质和巨大的适用性,目前处于几乎所有可以想象的领域的前沿研究的前沿 [1,2,3,4]。在 RNCOS 题为《2020 年全球 NP 市场展望》的技术市场研究报告中,据报道,2015-2020 年期间,NP 市场将以 16% 的复合年增长率(CAGR)增长。 NP 技术在生物医学和生物技术领域找到了独特的利基,其应用范围迅速扩大 [5, 6]。例如,NPs 已被应用于药物和基因传递 [7, 8] 病原体的生物检测 [9]、蛋白质检测 [10]、组织工程 [11、12]、肿瘤成像和靶向 [13]、肿瘤破坏通过热疗 [14] 和 MRI 对比增强 [15]。
由于其体积小,NPs 可以很容易地进入细胞以及跨细胞、组织和器官转移。纳米颗粒在生物医学应用中被广泛使用,因为它们能够穿过生物屏障进入细胞发挥其功能。然而,就像一把双刃剑,NP 的潜在风险(即不利影响)也源于这种能力 [16, 17]。尽管它们的“小”尺寸,作为极性分子的 NPs 不能通过细胞膜 (CM) 扩散。由于 CM 主要可渗透小分子和非极性分子,因此 NPs 采用内吞途径进入细胞 [18, 19]。 NPs 进入细胞的方式是决定其生物医学功能、生物分布和毒性的关键因素。在纳米医学中,纳米颗粒安全进入细胞是获得高疗效的关键步骤。此外,考虑到这些载体旨在靶向特定的亚细胞区室并传递特定的生物分子,如造影剂、基因和药物,NPs 的细胞内运输和命运是 NPs 成功的重要过程 [18, 20,21,22 ]。更重要的是,NPs 对细胞毒性的诱导是由其进入途径和细胞内定位决定的。因此,了解纳米颗粒的细胞摄取和细胞内运输对于设计安全有效的纳米药物至关重要[23]。
纳米颗粒的细胞摄取、靶向和细胞内运输可以通过调整纳米颗粒的物理化学特性(如大小、形状和表面特性)进行优化 [24]。因此,了解细胞摄取所涉及的潜在机制对于评估 NPs 的命运及其毒性至关重要。本综述重点介绍了 NPs 的不同可能摄取途径及其细胞内运输途径。此外,还讨论了 NP 的物理化学特性,如大小、形状、电荷和表面化学对其被细胞内化的影响。了解 NPs 与其细胞摄取机制相关的理化特性将使我们能够设计出在生物医学应用中至关重要的功能性 NPs,例如以受控方式在目标作用部位递送药物有效载荷,对周围健康组织的毒性影响最小和器官。
纳米颗粒的细胞摄取途径
CM,也称为质膜,通过将细胞内液与细胞外液分离来包围细胞质。 CM 非常重要,因为它可以保护细胞内成分、维持细胞稳态、提供结构支持并保留细胞的组成 [25,26,27,28,29]。 CM 由排列在双层中的磷脂组成,其中嵌入了蛋白质。这些具有亲水头和疏水尾的磷脂双层允许小生物分子进入。更具体地说,CM 是一种选择性渗透屏障,可控制物质进入细胞 [30, 31]。 CM采用不同的机制来交换物质,主要分为两类:被动运输和主动运输。氧气和二氧化碳等气体、苯等疏水性分子和水和乙醇等不带电分子从高浓度区域向低浓度区域扩散穿过膜。这种沿浓度梯度不借助能量而发生的输运称为被动输运。相反,主动转运是通过使用三磷酸腺苷 (ATP) 提供的能量来逆浓度梯度发生的 [32,33,34,35,36]。
不能通过疏水质膜的极性或带电生物分子通过称为内吞作用的主动运输形式被内化。在这个过程中,细胞通过 CM 的内陷吞没细胞外液内的物质,并在细胞内萌芽,形成一个称为内体的膜囊泡 [37]。胞吞作用基本上可以分为两大类:吞噬作用和胞饮作用。吞噬作用(细胞吞噬)是被称为吞噬细胞的特殊哺乳动物细胞(即单核细胞、巨噬细胞和中性粒细胞)吸收碎片、细菌或其他大尺寸溶质的过程[38, 39]。
吞噬作用不可或缺的是一个称为调理作用的过程,通过该过程,诸如免疫球蛋白和补体蛋白之类的调理素覆盖目标材料以触发吞噬细胞的存在并启动吞噬活性 [40]。当吞噬细胞开始摄取目标物质时,它会同时刺激称为吞噬体的膜结合囊泡的形成,其中摄取的物质在吞噬细胞内被分隔。在该过程的后期,吞噬体将与溶酶体融合,材料在酸性 pH 下被溶酶体腔内的水解酶消化 [41,42,43]。
在所有细胞类型中,纳米范围内的小颗粒通过胞饮作用被内化 [44]。在胞饮作用中,“细胞饮用”质膜形成内陷以吸收一小滴细胞外液,其中包括溶解的分子。胞饮作用不是一个区分过程,它以连续的方式发生在几乎所有细胞中,而不管细胞的需要。被抓取的物质被挤压成称为松香体的小囊泡,它与溶酶体融合以水解或分解内容物 [45, 46]。吞噬作用和胞饮作用可以通过它们的内吞囊泡的大小来区分;前者包括大小为 250 nm 的大囊泡对大颗粒的吸收,后者包括通过几纳米到数百纳米范围内的小囊泡对流体的吸收 [42, 47]。胞饮作用可细分为网格蛋白介导的内吞作用、小窝介导的内吞作用、网格蛋白和小窝细胞独立的内吞作用和巨胞饮作用[48, 49]。
网格蛋白介导的内吞作用是将特定分子内化到细胞中的细胞进入机制。这种进入途径有助于细胞通过低密度脂蛋白受体吸收质膜成分和营养物质,包括胆固醇,通过转铁蛋白受体吸收铁 [50,51,52,53,54,55,56]。在这个过程中,细胞外液中的特定配体与 CM 表面的受体结合,形成配体-受体复合物。这种配体-受体复合物移动到 CM 中富含网格蛋白的特定区域,从而通过形成网格蛋白包被的囊泡将它们吞没。一旦进入细胞,囊泡外部的网格蛋白涂层在与早期内体融合之前被排出。早期内体中的货物最终将通过内溶酶体途径到达溶酶体 [40, 57,58,59,60]。每种类型的 NP 都通过优先摄取途径被细胞内化。例如,由聚(乳酸-乙醇酸共聚物)、D,L-聚丙交酯和聚(乙二醇-丙交酯)和二氧化硅(SiO2)基纳米材料组成的纳米颗粒通过网格蛋白介导的内吞途径被内化[61]。基于香豆素的固体脂质 NPs 通过非能量依赖途径被细胞内化,因为这些 NPs 的结构类似于 CM。所有基于脂质的 NPs 都利用网格蛋白介导的内吞作用途径 [62]。赫赛汀包覆的金纳米颗粒通过膜ErbB2受体介导的内吞作用进入细胞[63]。
Caveolae 介导的内吞作用是细胞进入的途径,涉及称为小窝(小洞穴)的烧瓶状膜内陷。小窝存在于内皮细胞、上皮细胞、脂肪细胞、肌肉和成纤维细胞中 [64,65,66,67]。小窝的大小通常在 50 到 80 纳米之间,由膜蛋白小窝蛋白-1 组成,赋予它们烧瓶状结构 [68,69,70,71]。细胞膜蛋白、脂质和脂肪酸的细胞信号传导和调节涉及细胞膜内陷依赖的内吞作用 [61, 64, 67]。一旦小窝从质膜上分离,它们就会与一个称为小窝的细胞室融合,该小室在中性 pH 值下存在。 Caveosomes 能够绕过溶酶体,因此保护内容物免受水解酶和溶酶体降解。因此,包括病毒和细菌在内的病原体使用这种进入途径来防止降解。由于通过caveolin依赖性机制内化到细胞中的货物不会最终进入溶酶体,因此该途径被用于纳米医学[54, 72,73,74]。
网格蛋白和小窝蛋白独立的内吞作用发生在缺乏网格蛋白和小窝蛋白的细胞中。生长激素、细胞外液、糖基磷脂酰肌醇 (GPI) 连接蛋白和白细胞介素 2 利用该途径进入细胞。例如,叶酸采用网格蛋白和小窝独立途径进入细胞 [58, 72, 75,76,77,78,79] 与用于药物递送系统和作为显像剂的 NPs 和聚合物结合 [53 , 80, 81]。巨胞饮作用是一种胞饮作用机制,其中细胞通过形成称为巨胞饮体 [82,83,84,85] 的大囊泡 (0.5-10 μm) 来吸收大量细胞外液。巨胞饮作用是一种内化凋亡和坏死细胞、细菌和病毒以及抗原呈递的途径。该途径可以内化微米级纳米颗粒,而大多数其他途径不可能将这些纳米颗粒带入细胞。除脑微血管内皮细胞外,几乎所有细胞均可发生巨胞饮作用 [86,87,88,89]。 NPs 通过这些内吞途径之一进入细胞,如图 1 所示。
<图片>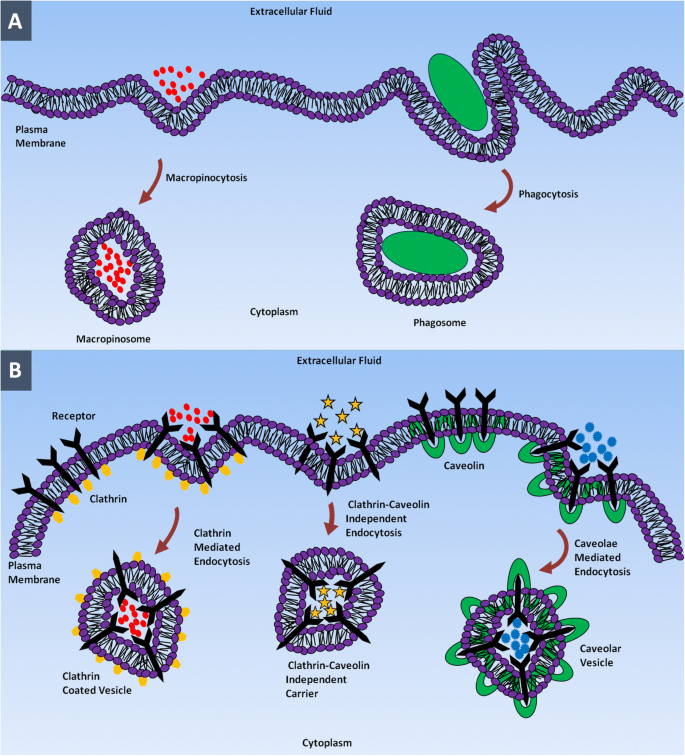
NPs 使用不同的内吞途径进入细胞。 一 巨胞饮作用和吞噬作用。 b 网格蛋白介导的内吞作用、网格蛋白-小窝蛋白非依赖性内吞作用和小窝细胞介导的内吞作用
图>NP 理化性质对细胞摄取的影响
研究纳米颗粒的物理化学性质(如大小、形状、表面电荷、表面疏水性/亲水性和表面功能化)对细胞吸收的影响至关重要,因为这些参数直接影响纳米颗粒的吸收水平、内吞途径和细胞毒性。 [90, 91]。影响纳米颗粒细胞摄取的理化因素如图 2 所示。下一节将讨论这些参数对细胞-纳米颗粒相互作用的影响。
<图片>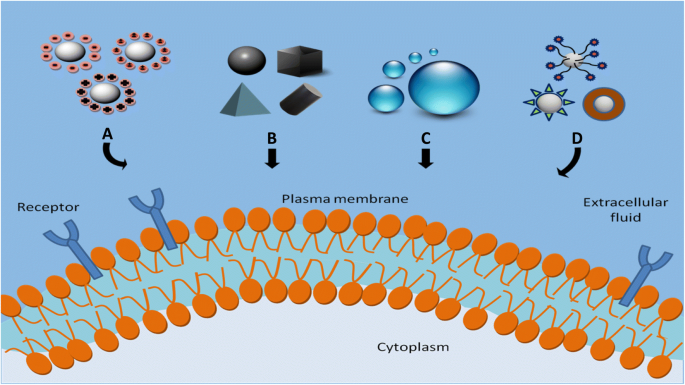
影响细胞对 NP 摄取的物理化学因素。 一 表面电荷,b 形状,c 大小和 d 表面化学
图>尺寸的影响
NP 的大小是决定细胞摄取效率 [92] 及其对活细胞的潜在毒性 [24] 的关键因素。此外,还发现 NP 的大小在决定摄取途径方面也起着重要作用。大小从几纳米到几百纳米不等的小纳米颗粒通过胞饮作用或巨胞饮作用进入细胞。尺寸范围为 250 nm 至 3 μm 的 NPs 已被证明具有最佳的体外吞噬作用,而尺寸范围为 120-150 nm 的 NPs 通过网格蛋白或小窝蛋白介导的内吞作用被内化,并且 NPs 的最大尺寸据报道,采用这种途径的波长为 200 nm [47, 93]。在小窝介导的通路中,小窝的大小阻碍了较大的 NPs 的吸收 [16, 17]。特定类型的 NP 可根据其大小利用多种内吞途径。
几项研究表明,对于 NPs 的细胞摄取,有一个 50 nm 的最佳大小,NPs 可以更有效地内化并具有更高的摄取率。对于较小的颗粒(约 15-30 nm)或较大的颗粒(约 70-240 nm),NP 吸收显示出减少 [94,95,96,97,98,99]。此外,30-50 nm 大小的 NPs 与 CM 受体有效相互作用,随后通过受体介导的内吞作用被内化 [97]。在纳米颗粒的给药应用中,主要关注的是防止纳米颗粒被网状内皮系统清除并延长其在血液中的循环时间,从而提高靶点的生物利用度。在这方面,增加 NPs 的大小将导致清除率的增加 [100,101,102,103,104,105]。因此,了解纳米颗粒大小在细胞摄取中的作用对于设计有效且安全的纳米颗粒用于医学应用至关重要。
尽管不同的研究调查了 NP 大小与摄取途径之间的关系,但揭示的结果始终不一致 [93, 106,107,108,109]。这些矛盾可能与在控制大小的过程中控制 NP 其他参数的复杂性有关。除此之外,在体外和体内研究期间,合成后测量的 NP 的大小可能会发生变化,原因是团聚和聚集,这反过来又会影响细胞内化途径 [110, 111]。通过在 50-1000 nm 范围内使用不同尺寸的荧光乳胶珠,研究了粒径对非吞噬 B16 细胞中细胞摄取途径的影响 [93]。结果表明,这些珠子的内化机制在很大程度上依赖于粒径。特别是,尺寸为 200 nm 或更小的珠子被网格蛋白包被的小坑吸收,而较大的珠子被小窝介导的内吞作用内化。 Lai 及其同事 [16] 发现尺寸小于 25 nm 的小聚合物 NP 采用一种新机制通过内/溶酶体途径外的非降解囊泡到达细胞的核周区域。该通路是非网格蛋白和非小窝介导的,不依赖胆固醇的。
SK-BR-3 细胞对与赫赛汀-AuNPs 结合的不同尺寸(2 到 100 nm)的金 (Au) NPs 的吸收显示出尺寸依赖性。对于 25-50 nm 大小范围内的 NP,观察到最高的细胞内化[63]。在这个进入途径中,发现 NP 的大小是膜受体结合和激活以及蛋白质最终表达的决定因素。评估了胶体 AuNP 大小和形状的变化对细胞内摄取的影响 [112]。 14-、50- 和 74-nm 大小的球形和棒状 AuNPs 与 HeLa 细胞一起孵育。发现 NP 的吸收很大程度上取决于其大小和形状,50 nm 大小的颗粒显示出最高的吸收率。此外,球形 AuNPs 的吸收比类似尺寸的棒状 NPs 高 500%。山等人。 [113] 研究了 HeLa 细胞内吞直径为 4、12 和 17 nm 的 AuNP 的大小依赖性力。结果表明,AuNPs 的大小增加了吸收力和解离力值。 A549 细胞(肺上皮细胞)对不同尺寸(50、100 和 300 nm)的 SiO2 NPs 的吸收已通过流式细胞术、荧光和电子显微镜的组合进行了研究。这些研究人员已经表明,SiO2 NPs 的吸收随着尺寸的增加而减少[114]。
形状效果
除了大小之外,NP 的形状在 NP 的吸收途径和运输中也起着举足轻重的作用。奇特拉尼等人。 [112] 研究了胶体金纳米粒子的形状对 HeLa 细胞摄取的影响。结果表明,球形 AuNPs 的吸收率是棒状 AuNPs 的五倍。在另一项工作中,同样的研究人员研究了球形和棒状转铁蛋白包覆的 AuNP 在三种不同细胞系上的摄取水平; STO 细胞、HeLa 细胞和 SNB19 细胞 [94]。他们观察到球形AuNPs被所有细胞系内化的速率高于棒状AuNPs。
为了确定体内形状的影响,Geng 及其同事 [115] 使用 filomicelles 来评估啮齿动物中带有球体的柔性细丝在运输和运输方面的差异。结果表明,丝束在循环中的保留量是球形对应物的十倍左右。此外,球状丝束比较长的细丝更容易被细胞内化。 Gratton 及其同事 [106] 证明了单分散水凝胶颗粒的形状对 HeLa 细胞吸收的影响。他们发现,与球体、圆柱体和立方体相比,棒状纳米颗粒具有最高的内化率。在另一项研究中,通过在 Caco-2 细胞上使用圆盘形、球形和棒状聚苯乙烯 (PS) NPs 来研究 NPs 形状对细胞摄取的影响。结果表明,杆状和盘状 NPs 的内化程度是球形 NPs 的两倍。他们得出结论,通过考虑纳米颗粒的形状可以促进纳米颗粒介导的药物递送[116]。
Xu 及其同事 [117] 通过制备具有不同形态的异硫氰酸荧光素 (FITC) 的层状双氢氧化物 (LDH) NPs 研究形状对细胞摄取的影响,例如六边形片状(横向宽 50-150 nm 和 10-20 nm厚)和棒(30-60 nm 宽和 100-200 nm 长)。所有形态均通过网格蛋白介导的内吞作用获得。 LDH-FITC 纳米球保留在细胞质中,而 LDH-FITC 纳米棒通过微管向细胞核移动。达斯古普塔等人。应用 [118] 模拟来探测 NPs 形状对细胞摄取的作用。他们模拟了纳米棒状和纳米立方体状 NP 的膜包裹。对于棒状颗粒,他们发现了稳定的内吞状态,具有小而高的包裹率;纵横比的增加对于完整的包裹来说是不可取的。 Nangia 和 Sureshkumar [119] 通过应用先进的分子动力学模拟技术,计算机化了形状对 NPs 易位率的影响。该研究的一个主要启示是锥状、立方体、杆状、水稻状、金字塔状和球状纳米颗粒的易位率发生显着变化。
表面电荷的影响
影响纳米颗粒细胞摄取的另一个关键因素是表面电荷。在最近十年中,纳米表面改性已被用于将 NPs 的表面电荷设计为阳离子或阴离子[92]。带负电的 CM 增强了带正电的 NPs 的吸收。特别是,带正电的 NPs 比中性和带负电的 NPs 具有更高的内化 [47, 120]。然而,带正电的 NPs 的摄取可能会破坏 CM 的完整性并导致毒性增加 [121, 122]。一般来说,带正电荷的 NP 会诱导细胞死亡 [123, 124]。有趣的是,与带负电荷的 NPs 相比,带中性电荷的 NPs 会降低细胞摄取 [110, 125,126,127]。此外,带负电荷的 NPs 的内化导致膜凝胶化,而带正电荷的 NPs 导致 CM 的流动性 [128, 129]。除了 NP 的吸收率外,表面电荷也会影响吸收机制。更具体地说,带正电荷的 NPs 主要通过巨胞饮作用被细胞内化,而网格蛋白/小窝蛋白独立的内吞作用是吸收带负电荷的 NP [130] 的机制。当 AuNPs 的表面被有机分子覆盖时,细胞摄取途径会发生变化。例如,带正电荷的普通 AuNP 通过巨胞饮作用和网格蛋白和小窝蛋白介导的内吞作用被内化,而带负电荷的聚乙二醇 (PEG) 包被的 AuNP 主要通过小窝蛋白和/或网格蛋白介导的内吞作用被内化 [131]。 /P>
Li 和 Gu [132] 通过分子动力学模拟研究了带电和中性 NPs 与 CM 的相互作用。发现与中性 NPs 相比,带电 NPs 对 CM 的粘附性更好。此外,通过增加 NPs 的电荷密度,它们可以被膜完全包裹。另一个研究小组采用分子动力学模拟来研究阳离子和阴离子 AuNP 与 CM 的相互作用。结果表明,随着 AuNPs 电荷密度的增加,AuNPs 渗透对 CM 的破坏增加 [133]。这些发现提出了一种通过操纵 AuNPs 的表面电荷密度来控制细胞和 AuNP 之间相互作用的方法,以优化其吸收,同时最大限度地减少细胞毒性,这是任何被考虑用于生物医学应用的 NPs 的基本特征。
Li 和 Malmstadt [134] 研究了带正电荷和负电荷的 PS-NPs 与生物膜的相互作用。结果表明,阳离子纳米颗粒与膜的磷酸基团之间的强静电相互作用导致纳米颗粒与膜的结合和膜表面张力增强,进而导致孔的形成。据报道,SK-BR-3 细胞中带正电的 AuNP 的吸收率是带负电的 AuNP 的五倍。这些研究人员还探索了带正电荷的AuNPs通过非内吞作用途径被内化,而带负电荷的AuNPs通过内吞作用途径被细胞吸收[135]。
豪克等人。 [107] 探测了 HeLa 细胞对尺寸范围为 18 至 40 nm 和表面电荷范围为 + 37 mV 至 - 69 mV 的金纳米棒(AuNRs)的吸收。结果表明,对于所有浓度的 AuNR,HeLa 细胞的最高内化是表面电荷 + 37 mV,最低内化是 - 69 mV。 Huhn 及其同事 [136] 评估了胶体 AuNP 与不同细胞系(如 3T3 成纤维细胞、鼠 C17.2 神经祖细胞和人脐静脉内皮细胞)的电荷依赖性相互作用。结果表明,对于所有细胞系,阳离子 AuNPs 的吸收率高于阴离子对应物。他们得出结论,细胞摄取高度依赖于电荷的符号。此外,细胞毒性研究表明,由于对带正电荷的 NPs 更高的摄取,它们表现出比带负电荷的更高的毒性。
疏水性的影响
NP 的疏水性是它们与 CM 相互作用的决定因素 [92, 137]。几项研究证明了 NP 的疏水性对其与 CM 相互作用的影响。李等人。 [138] 通过采用分子动力学模拟研究了 NPs 的疏水性/亲水性对与 CM 相互作用的影响。结果表明,疏水性 NPs 在 CM 中产生了内含物,而亲水性 NPs 被发现吸附在 CM 上。在另一项研究中,模拟方法被用于研究疏水性对 NP-细胞相互作用的影响。观察到亲水性纳米颗粒被包裹,而疏水性纳米颗粒通过直接穿透膜嵌入双层的内部疏水核内[139]。
通过改变 NPs 的疏水性表面来评估 QDNPs 与混合脂质/聚合物膜的相互作用。据观察,疏水性 NPs 位于膜的混合脂质/聚合物单层中的聚合物域内,而亲水性 QDNPs 吸附在单层上并遍布整个区域,表明对空气/水界面处的分子堆积有更高的影响 [140] .研究了将具有混合疏水性和亲水性配体的功能化 AuNP 掺入脂质体壁中。结果表明疏水配体与双层疏水核相互作用,而亲水配体与水溶液相互作用[141]。
表面修饰效果
在纳米颗粒的生物医学应用中,纳米颗粒的表面化学修饰是用于降低毒性、增加稳定性以及控制和调节纳米颗粒细胞内化的关键步骤,因此它们的生物学命运 [142]。 NPs 的表面功能化主要由 PEG、负羧基 (-COOH) 基团、中性官能团如羟基 (-OH) 基团和正胺 (-NH2) 基团组成。 (-NH2) 量的增加导致表面正电荷增强,从而增加了纳米颗粒进入细胞的吸收 [143,144,145,146]。同样,-COOH官能团增加了NPs的负电荷,从而增强了其吸收[144]。
陶等人。 [147] 设计了用于肿瘤靶向的聚多巴胺功能化 NP-适配体生物偶联物。他们报告说,与非功能化 NPs 相比,功能化 NPs 具有更好的靶向功效,表明功能化 NPs 具有更高的细胞摄取率,这转化为增强的治疗效果。在另一项研究中,叶酸功能化 NPs 在靶向宫颈癌细胞方面表现出比非功能化 NPs 更高的功效 [148]。 Qiu 及其同事研究了表面涂层对 AuNP 毒性和细胞吸收的影响 [90]。他们发现表面包被是决定细胞摄取率的关键因素,因为聚(二烯丙基二甲基氯化铵)包被的AuNRs显示出更高的细胞内化效率。
姜和同事研究了原始聚苯乙烯 (PS-NPs) 和氨基官能化聚苯乙烯 NPs 的细胞摄取差异 [149]。结果表明,氨基官能化聚苯乙烯 NPs 比 PS-NPs 具有更高的吸收率,前者主要通过网格蛋白介导的途径内化,后者通过网格蛋白非依赖性内吞作用。这种显着差异突出了表面化学修饰在与纳米颗粒的细胞相互作用中的关键作用。表面改性富勒烯,C60( C( COOH) 2) 2 NPs 主要通过内吞作用以时间、温度和能量依赖性方式被细胞内化。发现网格蛋白介导的内吞作用是 C60( C( COOH) 2) 2 个 NP [150]。
弹性的影响
NPs 的弹性是影响其被细胞内化的内在因素。 NPs 的弹性可以用它在施加力时对变化的抵抗力来解释。刚度、硬度和刚性是描述 NP 弹性的同义词。用于衡量 NP 弹性的测量指标是杨氏模量,测量单位是帕斯卡 (Pa)。基于此测量,较高的杨氏模量值表示较高的 NPs 弹性,反之亦然。用于测量 NP 值的分析设备或仪器的例子是原子力显微镜、流变仪和纳米压痕仪。具有较高弹性值的 NP 称为硬 NP,例如金 NP、量子点和磁性 NP。弹性值较低的纳米颗粒称为软纳米颗粒,例如水凝胶、脂质体和可生物降解聚合物。
许多关注 NP 与细胞摄取相关的参数的研究报告称,与较软的 NPs 相比,细胞更倾向于更有效地内化较硬的 NPs [151, 152]。显然,这一观察结果归因于与较软的 NPs 相比,包裹更硬的 NPs 的膜的总能量消耗较少,即使包裹 NPs 所需的变形能量在整个内化过程中发生变化。此外,使用粗粒分子动力学 (CGMD) 模拟进行的具有不同弹性的 NP 的膜包裹的计算建模与关于内化刚性和软 NP 所涉及的变形能量变化的实验观察一致 [153]。 However, there are also other studies that have reported on softer NPs being internalized more efficiently than stiffer NPs [154, 155] and intermediate elastic NPs internalized more efficiently compared to either stiff or soft NPs [156]. Hence, tuning the elasticity of NPs for better cellular internalization could be a valuable tool in biomedical applications such as drug delivery. A potential application was demonstrated by Guo and coworkers, whereby accumulation of nanolipogels in tumour cells were enhanced primarily by controlling this parameter of NP [157].
Intracellular Trafficking of NPs
In the previous sections, different possible uptake pathways of NPs and the parameters that affect the efficacy of uptake has been discussed. Following uptake, the next crucial matter is the intracellular trafficking of NPs which determines its final destination within cellular compartments, its cytotoxicity and its therapeutic efficacy [158, 159]. After NPs are internalized by the cells, they will first encounter membrane-bound intracellular vesicles called early endosomes. Endosomes formed at the plasma membrane are categorized into three types; early endosomes, late endosomes and recycling endosomes [106, 160,161,162,163].
Early endosome ferries the cargo to the desired cellular destination. Part of the cargo is recycled to the plasma membrane via recycling endosomes. Early endosomes transform into late endosomes via maturation and differentiation process. The late endosomes will then integrate with lysosomes to form endolysosomal vesicles and hydrolytic enzymes contained within these vesicles degrade the trapped NPs [18, 164,165,166]. However, some NPs are able to escape this pathway and are released into the cytoplasm therefore bypassing the lysosomal degradation process [167,168,169]. Another intracellular degradation pathway which plays important role in the intracellular fate of NPs is an intracellular process called autophagy [170,171,172]. In this process, cytoplasmic contents will be surrounded by autophagosome and delivered to the lysosome to be broken down and recycled [173]. In addition, aggregated proteins and dysfunctional organelles are degraded by autophagy to maintain cellular homeostasis. It is necessary to consider this pathway since recent studies demonstrated that several NPs are capable of inducing autophagy [174,175,176,177,178].
The intracellular trafficking of Tat peptide-conjugated quantum dots (Tat-QDs) in live cells was studied by Ruan and co-workers [179]. Dynamic confocal imaging showed that Tat-QDs interacted with negatively charged CMs leading to its internalization by macropinocytosis. The QD containing vesicles were observed to be actively transported by molecular motors towards the perinuclear region known as the microtubule-organizing center (MTOC). Tat-QDs bind to cellular membrane structures such as filopodia and vesicle shedding results in releasing QD-containing vesicles from the tips of filopodia.
The uptake and intracellular fate of fluorescent carboxylated polystyrene particles (20 nm and 200 nm in diameter) were evaluated by applying it on hepatocyte [180]. It was found that the particles were internalized by hepatocytes in size, time and serum-dependent manner. The fate of the particles was studied and they were not observed in early endosomes or lysosomes, but only in the mitochondria of the hepatocyte. Particles accumulated inside bile canaliculi show that NPs can be eliminated within bile. A study on the uptake and intracellular fate of silver NPs into human mesenchymal stem cells demonstrated that they agglomerate in the perinuclear region [181]. It was observed by using fluorescent probes that particles are contained within endo-lysosomal structures but not in the cell nucleus, endoplasmic reticulum or Golgi complex. Confocal imaging of FITC conjugated titania nanotubes in mouse neural stem cells revealed that they have crossed the karyotheca entering the cell nucleus [182]. Single-walled carbon nanotubes were observed to enter the cytoplasm and localize in the cell nucleus leading to cell mortality [183]. Translocation of AuNRs towards the nucleus has also been reported [184].
结论
The application of NPs in the modern world is growing at an exponential rate as the scientific enterprise is looking for novel ways to address current problems. NPs can be found as active ingredients in many formulations intended for human consumption, from cosmetics to processed foods. As its application increases in consumer products, so does human exposure to NPs. Hence, more research should be carried out to understand its potential hazards to humans and other living beings. In this review, we have looked at the current knowledge on the effects of NPs at a cellular level. Some of the topics discussed include cellular pathways of NPs and the influences of physiochemical properties of NPs on the uptake rate and uptake mechanism.
缩写
- ATP:
-
Adenosine triphosphate
- CAGR:
-
Compound annual growth rate
- CM:
-
Cell membrane
- FITC:
-
Fluorescein isothiocyanate
- GPI:
-
Glycosylphosphatidylinositol
- LDH:
-
Layered double hydroxide
- MTOC:
-
Microtubule-organizing center
- NP:
-
Nanoparticle
- PEG:
-
Polyethylene glycol
纳米材料
- 用于癌症治疗的纳米粒子:当前的进展和挑战
- 生物相容性 FePO4 纳米颗粒:药物递送、RNA 稳定和功能活性
- 钴掺杂 FeMn2O4 尖晶石纳米粒子的制备和磁性
- Au@TiO2 蛋黄-壳纳米结构的制备及其在亚甲基蓝降解和检测中的应用
- 改性超支化聚甘油作为分散剂,用于控制和稳定碳氢化合物中的金纳米粒子
- 用于光热疗法和光声成像的聚吡咯涂层铁铂纳米粒子的合成和体外性能
- 通过腹膜内和静脉内给药途径对大鼠生物合成铜和氧化锌纳米颗粒的体内比较检查
- 分层多孔 Silicalite-1 封装的 Ag NP 的制备及其对 4-硝基苯酚还原的催化性能
- 金属和金属氧化物纳米粒子的绿色合成及其对单细胞藻类莱茵衣藻的影响
- 纳米粒子和超声波控制的水过冷
- 基于紫杉醇的靶向脂质纳米颗粒的抗增殖和细胞凋亡触发潜力,通过转铁蛋白受体增强细胞内化——白血病细胞研究
- PEG-PCCL 纳米颗粒的毒性评估及其负载紫杉醇抗肿瘤作用的初步研究