掺杂 Fe 的可生物降解氧化锌纳米颗粒作为向活体输送铁的潜在形式的初步研究
摘要
铁是生物体的关键元素,它的缺乏被描述为全世界最常见的营养障碍。如今,对人类和动物更有效和更安全的铁补充策略已成为治疗营养缺乏症的最重要挑战之一。我们之前的体内研究证实了内部制造的基于氧化锌的纳米粒子的安全性和生物降解性,以及它们在体内大多数器官和组织的快速分布。对 Caco-2 细胞系(一种胃肠道上皮细胞模型)进行的体外检查显示,所研究的纳米材料具有低毒性。在目前的研究中,我们研究了掺杂 Fe(III) 的可生物降解氧化锌纳米粒子作为缺铁的一种前瞻性补充策略。将可生物降解的 ZnO:Fe 纳米颗粒对成年小鼠进行胃内给药,24 小时后处死动物并收集内部器官用于进一步分析。用原子吸收光谱法和组织学染色(Perl 方法)测量的铁浓度显示铁掺杂纳米粒子快速分布到与铁稳态相关的组织。在肝细胞内和脾脏血管周围也可见铁的积累,这可能表明掺铁的纳米颗粒从血流转移到组织中。假设,目前研究中获得的初步结果表明,掺杂有 Fe 的可生物降解的 ZnO 纳米粒子可能是生物体内外源铁的良好载体。因此,后续研究的重点是确定与组织中铁沉积相关的确切机制以及纳米颗粒载体对铁代谢的影响。
介绍
铁是在地球上构建生物体的最重要成分之一,包括人类。该元素的重要性与铁在体内发生的许多结构和过程中的重要作用有关,例如在整个身体组织和细胞(血红蛋白和肌红蛋白的一部分)周围运输氧气,产生更多能量(线粒体呼吸链蛋白的组成部分)[1, 2]。因此,铁参与细胞和整个生物体的关键生命过程,其缺乏可能导致生物体功能的显着紊乱。
世界范围内的报道显示,缺铁已成为人类严重的公共卫生问题。据世界卫生组织称,1993-2005 年间,全世界近 30% 的社会受到贫血症的影响。幼儿和孕妇的贫血患病率仍然更高[3]。孕期补铁的重要性与缺铁是早产、新生儿低出生体重及其一般健康状况恶化的危险因素有关 [4]。对于婴幼儿、学龄前儿童,缺铁的危险因素是全身快速生长和随后消耗铁储备;因此,也建议 0 至 5 岁的儿童补充铁 [5]。有趣的是,有科学报告表明儿童体内适当的铁水平与其情绪和神经心理发育之间存在相关性 [6]。此外,缺铁也是其他哺乳动物物种的严重营养障碍,尤其是在哺乳仔猪中,这种元素在宫内的供应有限,加上母猪乳中的铁含量低 [7, 8]。此外,仔猪的快速生长会迅速消耗剩余的铁储备,因为在几天内新生仔猪的体重会翻倍,从而增加红细胞的数量和总体血容量。因此,目前对人类和动物群体更有效、更安全的补铁策略成为预防和治疗营养缺乏的最重要挑战之一。
有三种主要的方式将额外的铁输送到活生物体:静脉内、肌肉内和口服。它们中的每一个都展示了该方法的优点和缺点。口服给药对身体来说似乎更简单、更自然,涉及铁吸收和运输系统的生理途径。此外,这种方法保留了一个基于铁调素控制体内循环铁量的自然系统 [9]。然而,口服补充途径往往存在吸收差和最终效率低的问题。科学研究还报告说,标准的可溶性口服补铁策略强烈影响动物肠道微生物组的组成和代谢活动 [10]。另一方面,向机体输送铁的静脉内或肌肉内方式受到毒副作用风险的阻碍[11]。此外,在工业化养猪业中,基于个体、肌肉注射单剂量右旋糖酐铁的标准补铁方法给动物带来压力,给饲养员带来麻烦。此外,注射不当会导致动物出现炎症和其他并发症,并在未观察到不育条件的情况下在畜群中传播各种疾病。
上面提到的外源性铁对生物体的毒副作用与体内游离和未结合铁元素的发生有关,铁元素作为过渡金属(通过价态变化)会导致有害自由基(即活性氧)的产生物种-ROS)通过芬顿反应[12]。科学报告表明,生物体内自由基水平升高的风险是导致各种疾病的一个因素,包括:细菌感染、肿瘤或肝脏疾病 [13]。防止游离铁产生 ROS 的自然生理策略是基于在体内吸收和运输途径的每个步骤中将铁元素与特定蛋白质结合。在口服铁超负荷及其静脉/肌肉给药的情况下,铁循环的生理途径普遍不堪重负,导致ROS迅速增加[14]。
铁基纳米粒子(Fe 基 NPs)已被广泛研究为用于生物医学目的的有前途的工具。这些纳米结构最广泛的用途与其磁性有关;因此,它们主要被测试为临床诊断技术的造影剂,旨在肿瘤成像或在特定组织中的积累 [15]。最近,纳米结构也被研究作为一种新的、更有效的将生物活性物质输送到生物体的形式。与散装形式的物质相比,NP 载体可能会显着提高生物体的生物利用度,这主要与其较小的尺寸有关 [16, 17]。在其中一项研究中,将含铁的 NPs 与硫酸亚铁(治疗贫血的标准补充剂)在贫血大鼠中的有效性进行了比较。根据血液分析,获得的结果显示在贫血动物中口服单剂量含铁的 NPs 后铁的生物利用度增加。在多剂量纳米颗粒和硫酸亚铁的情况下,获得的结果相似[18]。另一项研究描述了铁基纳米材料作为人类和啮齿动物模型替代铁递送剂的结果。作者特别强调了纳米铁与可溶性铁相比具有更高的安全性,这导致肠粘膜中铁积累不足并促进有益的微生物群 [19]。在用维生素 C 封端的基于 Fe 的 NPs 治疗的贫血大鼠的研究中也获得了类似的有希望的结果,这可以避免从胃肠道吸收 Fe 的标准途径。获得的结果表明,与缺铁治疗的标准方法相比,受试动物在短时间内从贫血病中恢复并改善了它们的血液参数[20]。
材料和方法
纳米粒子的合成
使用微波水热技术合成了掺杂铁的氧化锌纳米颗粒 (ZnO:Fe NPs)。该方法是成熟的、相对便宜的、生物友好的和工业可扩展的,能够将不同的外来离子作为功能性掺杂剂 [21,22,23,24,25] 引入各种氧化物 [26,27,28]。现有纳米颗粒中的铁浓度设置为 5% 摩尔。从硝酸盐 (V) 开始合成:Zn(NO3)2·6H2O(99%,Sigma-Aldrich)和 Fe(NO3)3·9H2O(96%,Carl Roth)。我们测试了不同的供应商,并选择了那些提供最统一的产品,其中不包含可测量水平的不可溶残留物。将化合物溶解在蒸馏水中:17.63 g硝酸锌(V)和1.25 g硝酸铁(III)(V)。然后使用 25% 氨水溶液 (Carl Roth) 将澄清溶液碱化至 pH 值为 8。使用布氏漏斗洗涤所得红色残留物,用约 1 升蒸馏水并抽滤。将沉淀物置于 100 毫升特氟龙容器中,用水填充至体积的 80%,然后在 Ertec Magnum II 反应器室中封闭。微波水热工艺在4 MPa下进行20 min。反应后收集淡红色产物,60 ℃干燥过夜。
纳米粒子的表征
使用扫描电子显微镜 (SEM)、光致发光 (PL) 和阴极发光 (CL) 光谱以及能量色散元素分析 (EDX) 对产品进行表征。 SEM 测量是用高分辨率(1 nm)日立 SU-70 显微镜进行的,显微镜配备了特征辐射探测器和阴极发光系统 GATAN Mono CL3 二次电子(SE)和透射(TE)模式。使用配备氙灯作为激发源和 Hamamatsu R928P 光电倍增管的 Horiba/Jobin-Yvon Fluorolog-3 荧光分光光度计记录光致发光发射和激发光谱。使用 DelsaMax Pro 颗粒表征系统测量动态光散射 (DLS) 和 zeta 电位。使用 NETZSCH STA 449 F1 Jupiter 热重仪在氩气流下进行热重分析 (TGA)。 SEM 测量是在纳米粒子沉积在琼脂聚碳酸酯涂层的 400 铜网上之后进行的。使用Sonics VCX500超声波处理器将样品悬浮在蒸馏水中,然后将一滴悬浮液置于筛网上,然后干燥。样品在较重的颗粒沉降2小时后制备。
体外模型
对于当前的研究,使用 Caco-2 细胞系(高加索结肠腺癌细胞)作为胃肠道上皮细胞的模型。细胞系获自 The European Collection of Authenticated Cell Cultures-ECACC(Sigma-Aldrich,货号 86010202)。细胞以 0.5 × 10 6 接种 细胞/ml 到 6 孔或 96 孔板上并维持在 Dulbecco's Modification of Eagle's 培养基 - DMEM (Gibco),补充有 10% 胎牛血清 - FBS (Gibco),1% 非必需氨基酸 - NEAA (Gibco) )、1% 青霉素-链霉素-新霉素-PSN (Gibco) 和 0.2% 碳酸氢钠 (Gibco),温度为 37 °C 和 5% CO2。当观察到 95-100% 汇合时,去除细胞培养基并添加 ZnO:Fe NPs 在新鲜生长培养基中的悬浮液。将细胞与四种不同浓度的 ZnO:Fe NPs(1.0;0.1;0.01;和 0.001 mg ZnO:Fe NPs/ml)在 37 °C 和 5% CO2 下孵育 24 小时。
细胞活力分析
使用两种方法评估 Caco-2 的细胞活力:XTT 测定和台盼蓝染色。 XTT 测定所需的所有试剂均由商业试剂盒(细胞增殖试剂盒 II,Roche)提供。将在 96 孔板上生长的细胞与不同浓度的 ZnO:Fe NPs 孵育后,将细胞与 50 μl XTT 标记混合物在 37 °C 和 5% CO2 下孵育 4 小时。随后,用分光光度法评估甲臜浓度。在 470/650 nm 处测量吸光度,结果与活细胞数相关。在以下实验中,最高吸光度结果假定为 100% 的细胞活力。最终结果是基于四个独立的重复实验 (n =4).
为了进行台盼蓝染色,将在 6 孔板上生长的细胞与不同浓度的 ZnO:Fe NPs 孵育后,收集细胞(0.05%tripsin 和 0.2% EDTA 10 min),离心并重新沉淀沉淀。悬浮于 1 ml 生长培养基中。将 100 微升无菌 0.4% 台盼蓝溶液与 100 μl 细胞悬液混合。将 10 微升样品加载到计数载玻片上,并使用 JuLi Br Couting 软件 (NanoEnTek) 进行分析,以评估活细胞的数量。最终结果是基于三个独立的重复实验 (n =3).
动物模型
出于本初步研究的目的,成年雄性 Balb-c 小鼠 (n =6) 分别保持在标准和受控生活条件下(12/12 小时明暗期,25°C,湿度 30%),标准食物和水(随意提供)。所有程序均被当地伦理委员会接受,协议编号。 WAW2/59/2017。在适应 7 天后,通过口服管饲法 (IG) 向实验组 (n =4)。对照组小鼠通过 IG (n =2)。在实验过程中,我们没有注意到测试动物的任何行为变化,也没有发现任何组织异常。在 24 h 后,实验组和对照组的小鼠在 CO2-O2 室(CO2 Box,Bioscape,Merazet)中被处死,随后收集组织。一半新鲜收集的样品在 - 20 °C 下冷冻用于原子吸收光谱法 (AAS)。将下半部分组织固定在 4% 缓冲福尔马林中并转移到 70% 乙醇中(24 h 后)。随后,样品在浓度不断增加的乙醇中脱水,包埋在石蜡(Leica TP1020、Leica EG1150)中,并用切片机(Leica RM2255)制备 6 μm 薄(或 4 μm 薄用于组织病理学检查)的显微载玻片。
Perl 染色评估的铁积累
脾脏、肝脏和大脑的显微镜载玻片用 Perl 方法(普鲁士蓝)染色以确定铁的存在。将样品脱蜡并重新水化为蒸馏水,然后放入含有等份 5% 铁氰化钾 (Sigma-Aldrich) 和 5% 盐酸 (Sigma-Aldrich) 的工作溶液中,染色 30 分钟。随后,切片在水中冲洗并用核固红(Sigma-Aldrich)复染 5 分钟,用水冲洗,并用 Permount(Fisher Scientific)固定在盖玻片下。使用 Olympus BX60 显微镜和 Cell^P 图像采集软件拍摄染色样品的图像。根据家庭图像分析协议 [29],从每个测试样本中随机选择 16 张图像,用于使用 MicroImage v.4.0 (Olympus) 进行进一步检查。对于脾脏检查,在分析过程中只考虑了组织内的红髓。铁含量计算为图像中铁阳性染色(蓝色)的面积和强度与细胞核面积的比率。
用原子吸收光谱法测量的受试组织中的铁浓度
根据 [24] 之前详细描述的方案,先前收集、储存在 -20 °C 中并随后解冻的组织样品被制备用于使用原子吸收光谱法 (AAS) 方法进一步定量评估铁浓度。简而言之,将组织称重并在含有 5 ml 65% 硝酸和 1 ml 30% 过氧化氢 (Merck) 的溶液中保持> 16 小时。随后,样品在微波系统Ethos 900(Milestone,美国)中矿化成液体溶液,并用AAS(Perkin-Elmer)评估铁含量。
统计分析
研究中获得的数据表示为平均值±平均值的标准误差 (SEM)。为了对 Perl 染色获得的铁积累进行统计评估,对所有组进行单向方差分析和 Tukey-Kramer 多重比较事后检验。为了从 AAS 分析评估对照组和实验组之间的显着差异,未配对的 t 测试被使用。使用单向方差分析和 Dunnett 多重比较检验评估体外实验中对照组和实验组之间的显着差异。使用 GraphPad InStat 3.10 计算统计分析。对于所有测试,获得的结果被认为具有统计学意义,P ≤ 0.05 和 P ≤ 0.01 和 P ≤ 0.001 表示高度显着和极显着。
结果
纳米粒子的表征
在微波水热工艺中结晶的 ZnO:Fe 纳米晶体的扫描电子显微镜 (SEM) 图像如图 1 所示。合成后储存的干粉使用超声波浴分散在水中,然后沉积在 400 目铜上。将超声处理产生的悬浮液放置 2 h 以进行大颗粒的团聚和沉淀。在样品中通常可以看到呈细长六棱柱形状的 ZnO 晶体。晶体在 c 面生长的方向上被拉长 - [001]。在图像上可以清楚地看到棱镜的交叉点:六边形的大小为 50–100 nm。基本上,纳米颗粒聚集并形成更大的结构。在图 1b 中,显示了这种严重团聚和成群的 ZnO:Fe 晶体的结构。样品也由不均匀的晶粒组成,其尺寸分布在数百纳米和微米之间。除了 ZnO 相之外,没有明确的分离迹象,表明不存在铁基相(例如氧化铁)结晶 [30]。
<图片>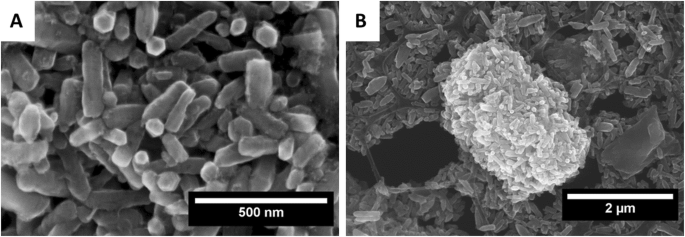
大型聚集体沉降后沉积在铜网上的 ZnO:Fe NPs 的扫描电子显微镜图像。放大倍数 100 kx (a ) 和 20 kx (b )。将每毫升水中含有 1 mg ZnO 纳米颗粒的悬浮液滴在聚碳酸酯涂层的铜网上,以实现高分辨率扫描显微镜观察
图>在氩气流下从室温到 800 °C 进行热重分析 (TGA) 和差示扫描量热法 (DSC),所得结果如图 2 所示。第一次质量损失发生在 200 °C 和它导致样品质量相对于其初始质量减少了 4.78%。第二个损失记录在 200 到 400 °C 之间,其值约为 7.36%。直到 800 °C,没有其他质量下降。第一个与表面吸附水分子的蒸发有关,第二个可能是由羟基减少引起的。 DSC曲线显示在118.3 °C处有吸热峰(-0.6175 mW/mg,水蒸发),在283.1 °C处有放热峰(0.4356 mW/mg,羟基分解)。与分解相关的小效应表明,ZnO:Fe纳米颗粒在微波水热过程中几乎完全结晶。
<图片>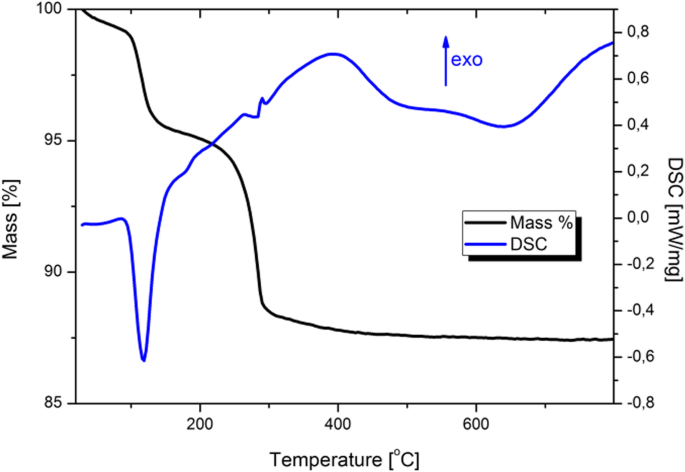
在氩气中加热的 ZnO:Fe NPs 的 TGA/DSC
图>图 3 显示了 ZnO:Fe NPs 的室温光致发光光谱。发射光谱(图 3a)由两个特征组成:低强度窄近带边缘 (NBE) 发光和非常强烈的宽深能级发射 (DLE) 发光。第一个在 ~ 380 nm 处达到峰值,而第二个在 600 nm 处达到峰值。 DLE 强度在光谱上的主导地位表明所获得的纳米晶体在晶体学水平上存在严重缺陷 [31,32,33]。激发光谱(图 3b)表明了 ZnO:Fe 带隙中深能级的发射机制。
<图片>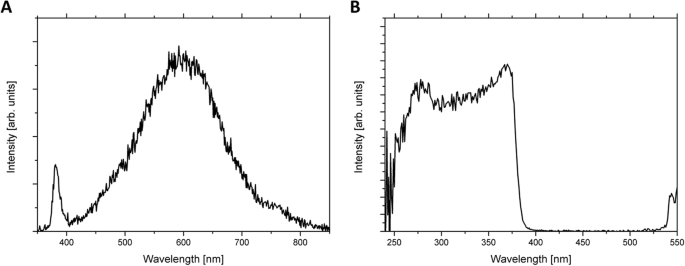
光致发光 (PL) 发射 (a , λexc =260 nm) 和激发 (b , λem =595 nm) ZnO:Fe NPs 的光谱。将样品松散地倒入铝基体中,然后重新平衡以进行 PL 测量。用光谱带通滤波器过滤激发波长的二阶和更高阶
图>ZnO:Fe NPs 的阴极发光光谱如图 4 所示; DLE/NBE 强度比 ≈ 25。 380 nm,DLE 波段包含四个分量,峰值在 ~ 570、625、653 nm,在 770 nm 处非常弱。 McCluskey 和 Jokela [34] 认为,570 nm 波段与氧空位有关,而其他波段则与纤锌矿型晶格中的锌空位有关 [35]。
<图片>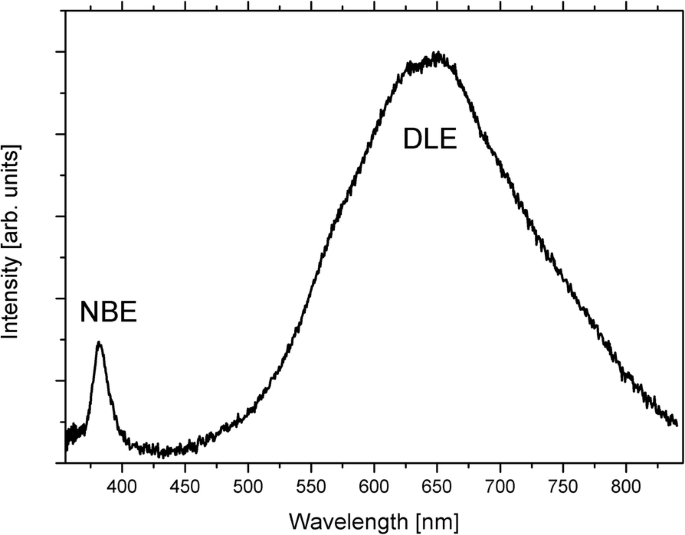
ZnO:Fe NPs 的阴极发光 (CL) 光谱。将干燥的纳米粉末造粒以获得源自大量样品的 CL 光谱。显示的条带说明了氧化锌纳米晶体中存在的晶体缺陷的数量和质量
图>使用两种技术测定样品中的铁含量:原子吸收光谱 (AAS) 和能量色散 X 射线光谱 (EDX)。在 AAS 的情况下,分析了与啮齿动物实验中使用的类似的悬浮液,表明 ZnO:Fe 中的 Fe 原子百分比为 3.98。通过EDX法,在铜网中分析干粉,发现铁的浓度为4.5%at。与锌离子有关。
使用 DLS 和 TEM 方法测量悬浮特性,纳米粒子直径的分布如图 5 所示。分布是双峰的:对于一个群体,最常见的直径在 20 到 100 nm (TEM) 之间以及在 50 到 50 纳米之间达到峰值200 nm (DLS)。第二个群体包含较低数量的较大物体 100–500 nm (TEM) 和约。 150–500 nm (DLS)。两种显示的方法都清楚地观察到纳米颗粒的双峰特征。较大群体中尺寸的高分散表明它对应于附聚的纳米粒子。直径大于 100 nm 的统计数据表明 ZnO:Fe 纳米颗粒有许多不同的凝聚方式。低于0 V的zeta电位表明,水悬浮液中的纳米颗粒带负电荷。大约-40 mV的值也表明最终的水悬浮液是稳定的,纳米颗粒对团聚没有亲和力。
<图片>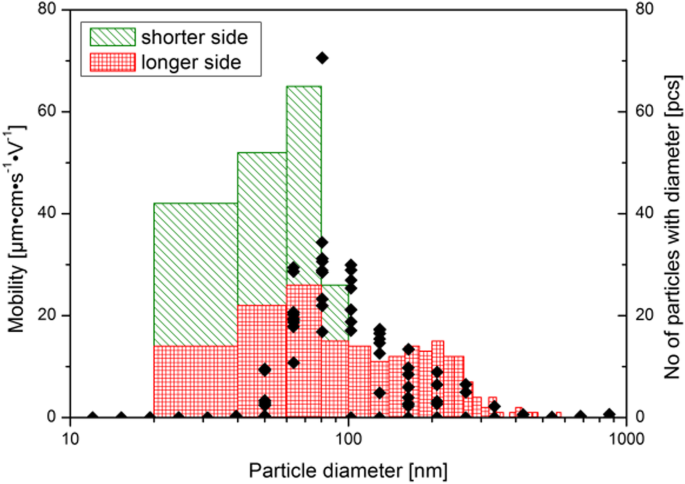
在水悬浮液中测量的纳米粒子流体动力学半径的分布(点),并直接取自沿纳米晶体短边和长边的 TEM 图像(见正文)。通过高功率超声波喇叭将纳米颗粒转移到水悬浮液中。干物质的浓度为每 1 ml 水 1 mg。沉降完成后,进行了十次迁移率测量,以获得可信的迁移率与纳米颗粒直径的关系图。 TEM 尺寸直接取自图像:沿 c 轴(ZnO 晶体的长边)和沿 m 和 a 轴(晶体的较短边)
图>ZnO:Fe 纳米颗粒的体外毒理学评价
两种细胞活力分析(XTT 和台盼蓝)都显示与 ZnO:Fe NPs 孵育的 Caco-2 细胞的吸光度降低,这与活细胞的数量直接相关。仅在孵育细胞暴露于最高浓度的 NPs(1.0 和 0.1 mg/ml)24 小时后才观察到统计学上的显着变化,而生理水平的 NPs 浓度(0.01 和 0.001 mg/ml)对细胞培养没有显着影响(图 6 和 7)。
<图片>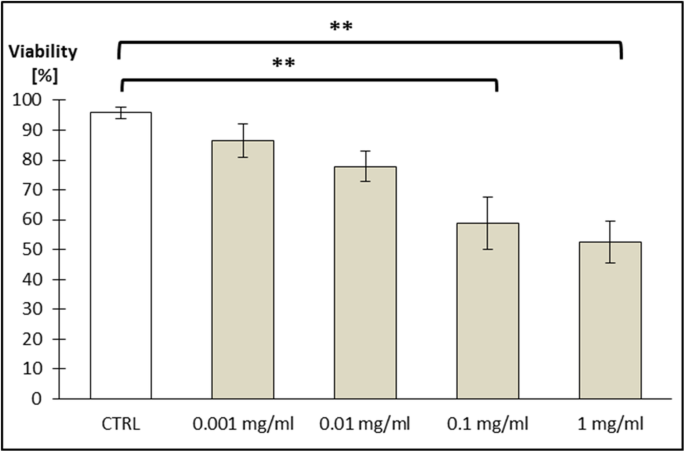
通过 XTT 方法测试细胞活力(Caco-2 系)。将细胞暴露于如下不同浓度的掺杂铁的 ZnO NPs 悬浮液 24 小时:0.001 mg/ml; 0.01 mg/ml; 0.1 mg/ml;和 1 mg/ml。结果代表对照组和实验组的平均活细胞百分比 (±SEM) (n =4)。 **P 的显着差异 ≤ 0.01 vs. 对照被标记在图表上
图> <图片>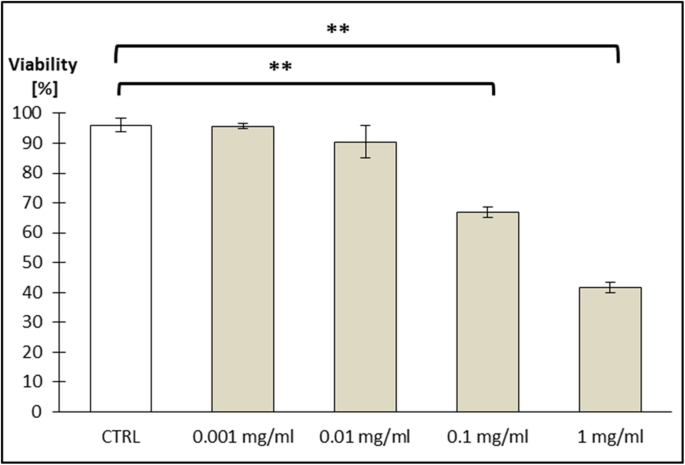
用台盼蓝染色测试细胞活力(Caco-2 系)。将细胞暴露于如下不同浓度的掺杂铁的 ZnO NPs 悬浮液 24 小时:0.001 mg/ml; 0.01 mg/ml; 0.1 mg/ml;和 1 mg/ml。结果代表对照组和实验组的平均活细胞百分比 (±SEM) (n =3)。 **P 的显着差异 ≤ 0.01 vs. 对照被标记在图表上
图>动物模型中的铁分布
为了评估 ZnO:Fe NPs 的体内铁可及性,小鼠 (n =4) 口服 ZnO:Fe NPs 的悬浮液,在 24 h 后,动物被划伤,进一步收集关键组织。使用 AAS 方法对所有收集的器官进行检查组织内铁含量的定量评估,并使用 Micro Image 软件(基于 Perl 染色)计算肝脏、脾脏和脑组织。如图 8 所示,数据显示在口服 ZnO:Fe NPs 后 24 小时,心脏、骨骼肌、脾脏和小肠组织中的铁含量(与对照相比)水平增加(无统计学显着性增加)。在骨骼的情况下,实验组内的平均铁水平仅略高于对照结果。肝、脑组织铁含量高显着升高(P ≤ 0.01) ZnO:Fe NPs IG 后 24 h,与对照组相比(图 8)。在肾脏、内脏和皮下脂肪组织以及肺中,施用 ZnO:Fe NPs 后 24 h 的 Fe 水平下降。
<图片>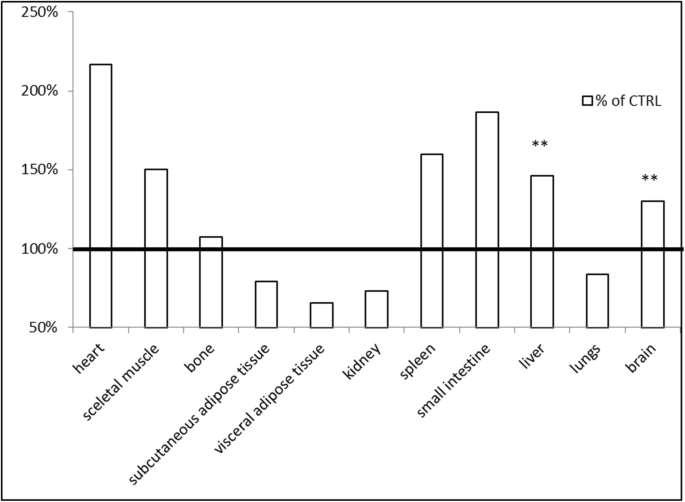
在 IG 施用 ZnO:Fe NPs 后 24 h 分析组织内的铁分布。数据代表实验组受试器官中 Fe 的平均百分比 (n = 4)与对照组比较(100%——横线)。铁含量用原子吸收光谱法分析。 **P 的显着差异 ≤ 0.01 与对照
图>用 Perl 法染色的肝脏内铁含量的评估显示极其显着 (P ≤ 0.001) 所有实验动物与对照动物的 Fe 水平增加(图 9b)。同样,脾组织红髓的结果显示出极其显着的(P ≤ 0.001) 所有实验动物与对照动物的铁含量增加(图 10b)。从脑组织分析获得的数据表明,在 ZnO:Fe NPs IG 后 24 h,实验组中 Fe 没有增加(图 11b)。
<图片>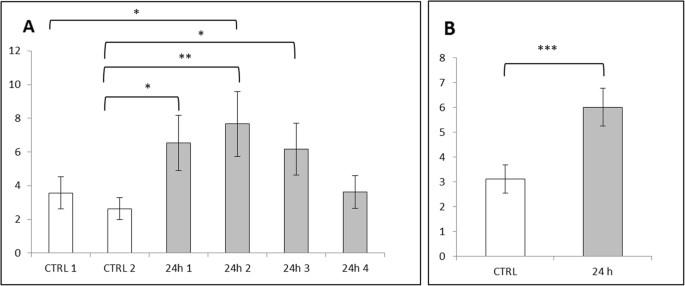
肝脏中的铁含量使用 Perl 染色和 MicroImage 软件计算。结果计算为铁阳性染色的面积和强度与细胞核面积的比率。数据表示为每个受检动物的平均值 (±SEM) 结果 (a ) 和作为对照组和实验组的平均 (±SEM) 值 (b )。 *P 的显着差异 ≤ 0.05, **P ≤ 0.01,且 ***P ≤ 0.001
图> <图片>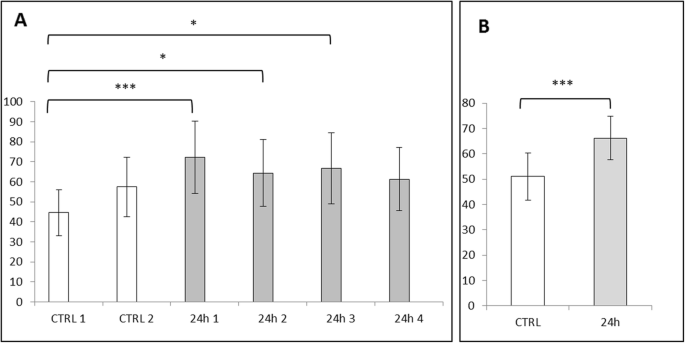
用 Perl 染色和 MicroImage 软件计算脾红髓中的铁含量。 Results calculated as a ratio of area and intensity of iron-positive stains to the area of cell nuclei. Data presented as mean (±SEM) result for each, examined animal (a ) and as mean (±SEM) value for both control and experimental groups (b )。 Significant difference of *P ≤ 0.05, **P ≤ 0.01, and ***P ≤ 0.001
图>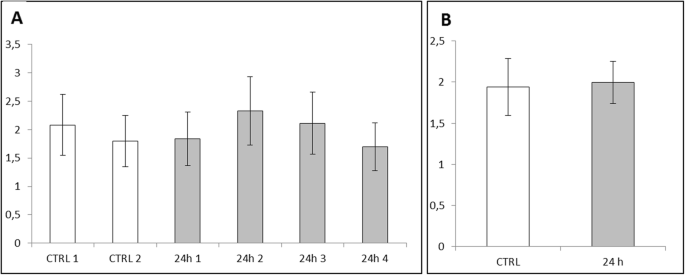
Iron content in the brain calculated with Perl’s staining and MicroImage software. Results calculated as a ratio of area and intensity of iron-positive stains to the area of cell nuclei. Data presented as mean (±SEM) result for each, examined animal (a ) and as mean (±SEM) value for both control and experimental groups (b )
图>In case of content of iron from each animal of experimental and control groups, results were collected at the Figs. 9a, 10a, and 11a. The highest and significantly different (P ≤ 0.05 and P ≤ 0.01) level of iron within liver tissue was detected for mice – 2 (24 h 2), comparing with results from control group (Fig. 9a). Levels of iron stains in red pulps of the spleen within the experimental group were similar; however, most of them were statistically higher (P ≤ 0.05 or P ≤ 0.01) than mice – 1 (CTRL 1) from the control group (Fig. 10a). Results obtained for brain tissue were similar between each animal from experimental and control groups (Fig. 11a).
Sections of liver and spleen tissues stained with Perl’s method were also qualitatively assessed. Increased number of iron depositions (light blue stains) was visible within hepatocytes from liver sections of experimental group (Fig. 12).
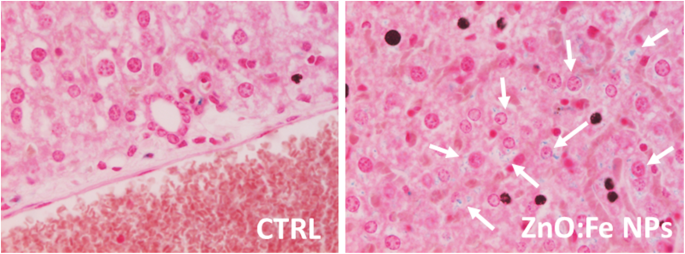
Representative microphotographs of liver sections showing staining with Perl’s method. Comparison of the presence of iron (light blue areas) in control and experimental groups of mice (with ZnO:Fe NPs administered 24 h before sacrifice). The arrows point iron deposits in hepatocytes. × 40 lens
图>General greater accumulation of iron (blue stains) within the red pulp than in white pulp of spleen was observed in both control and experimental groups (Fig. 13). After application of ZnO:Fe NPs, the higher level of iron was particularly visible within the red pulp, comparing with images from control group (Fig. 13c, d). In experimental group, an increased concentration of iron was also noticeable around blood vessels (Fig. 13b, d).
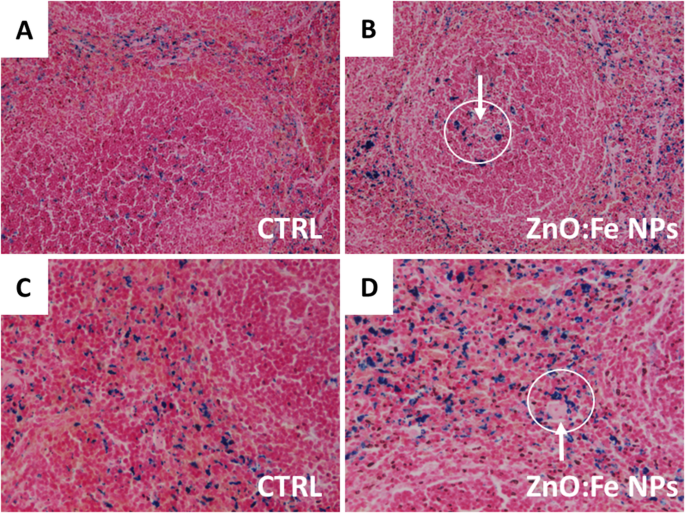
Representative spleen sections following staining with Perl’s method. Comparison of the presence of iron (blue areas ) in control and experimental group of mice (with administered 24 h before ZnO:Fe NPs). Separate presented areas of the tissue:white pulp (a , b ) and red pulp (c , d )。 The arrows point iron accumulated around blood vessels (circles). × 10 (a , b ) or × 20 (c , d ) lens
图>Discussion
As was mentioned in the “Introduction” section, the iron deficiency is a considerable nutritional disorder in human population, as well as in other mammalian species [3, 7, 8]. Consequently, an implementation of new, more efficient and safe iron supplementation strategy seems to be highly desirable. Currently presented results come from preliminary studies aimed at further, detailed investigations related with potential usage of biodegradable ZnO NPs doped with Fe as a novel strategy for iron supplementation. As was confirmed previously, in-house manufactured zinc-based NPs undergo very efficient absorption after their oral administration with further, rapid distribution to major organs and tissues in the living organism [22,23,24]. Moreover, we reported that these nanostructures demonstrated bio-safety and biodegradability within the body along with fast clearance from the organism [36]. Employed in the current study, the oral way of NP administration provides a highly efficient way of fast delivery of the exogenous nanostructures to the body, based on persorption process [37, 38]. Furthermore, this strategy decreases chance of eventual toxic effects related with administration of iron-doped substances, because of the existence physiological “security mechanisms” related to both Zn and Fe absorption from the gastrointestinal tract. This crucial, physiological pathways of iron distribution may be avoided in case of intravenous/intramuscular application iron-doped substances [14], which can result in increased possibility of occurrence iron-related toxic side effects. The examination of the internalization pathways of the nanoparticles, their dynamics, and cellular systems involved in the process is being currently studied in the frame of other publication. In the current paper, we decided to show overall effect of our nanoparticles on living organism, focusing on the effect of iron.
Toxicity of nanostructures may be related with various features of material, not only with chemical composition, but also with e.g. size, shape, or their ability to aggregate [39]. Nevertheless, it was confirmed that smaller NPs were better absorbed than bigger one and high surface-to-volume ratio makes the particles of some metals exceptionally reactive and cytotoxic [40, 41]. Nanostructures were able to enter cells and affect their components, which altered the viability of cells [42, 43]. In one of the previous investigations on cytotoxicity of ZnO or FeO NPs, authors did not observe toxic effects (based on ability of examined cells to grow and divide), even in high doses of NPs [44]. Presented here is a study performed on Caco-2 cell line, as a model of epithelial cells of the gastrointestinal tract, revealing a low toxicity of used nanomaterials. ZnO:Fe NPs in small/physiological doses (0.001 mg/ml and 0.001 mg/ml) did not alter the viability of cells (Figs. 6 and 7). Following the incubation of Caco-2 cells with high doses of ZnO:Fe NPs, a statistically significant decrease of viability was observed. However, IC50 (the half maximal inhibitory concentration), as a measure of the effectiveness of a substance in inhibiting a specific biological or biochemical function, still remained surprisingly high—around 1 mg/ml (Figs. 6 and 7). Observed drop of cell viability was probably associated with the physical deposition of nanoparticles on the surface and overstress of the cells rather than the cytotoxic effect related to the nanoparticles themselves. In another study, authors concluded decrease IC50 of cells from cancer cell line following incubation with ZnO and ZnO:Fe3O4 NPs and relatively low cytotoxicity effect (and non-dose-depended) of examined NPs on noncancerous cells [45]. Similar observations were also reported for ZnO and platinum NPs [46, 47]. Nevertheless, further examinations on chronic toxicity profile of such composites including antioxidant activity profiling, production of reactive oxygen species (ROS), and using another cells and cell line must be performed.
Obtained in the in vivo experiment, results indicated a rapid distribution of ZnO:Fe NPs to tissues mostly related with iron homeostasis (Figs. 8, 9, and 10). Similar studies, concerning the bioavailability of iron from “nano” form, were mostly performed on anemic animals or examined following the iron depletion period [18, 19, 48], where our preliminary studies were carried out on healthy, normally maintained mice. Comparison of data presented in the current work with similar studies may be misleading because of differences in employed NPs e.g. their size, shape and compound or route, dose, and frequency of their administration to animals. Results of iron level in the liver showed significantly increased level at 24 h after application of ZnO:Fe NPs, comparing with control (Figs. 8 and 9). Iron accumulation was visible in hepatocytes of liver tissue (Fig. 12), where the major storage site of the element takes place [49]. Similarly, previous studies with intraperitoneally injected iron oxide, magnetic NPs to rats also resulted in 55% accumulation of these nanomaterials in the liver at 6 h following injection [50]. Likewise, the spleen level of iron in the current study was clearly higher in experimental group, than within control (Figs. 8 and 10). Interestingly, tendency of iron accumulation was detected around blood vessels within the spleen, which might indicate the transfer of ZnO:Fe NPs from general bloodstream into the tissue (Fig. 13b, d). Primary accumulation of nanostructures within the liver and spleen after their application might also be attributed with clearance via mononuclear phagocytes in these tissues, as was also postulated previously [50,51,52].
In case of brain tissue, the measurement of total iron level (AAS method) showed significantly higher level of the element in animals with previously administered ZnO:Fe NPs (Fig. 8), which was not confirmed by analysis based on Perl’s staining (Fig. 11). Inconsistency between both, employed in the study methods, may be related with sensitivity of Perl’s staining. This histological method of iron visualization is dedicated to detect iron aggregates. Iron contained in the “nano” form, additionally distributed within the tissue without physiological deposits of these elements, might not be sensitive enough to stain these extremely small deposits of iron. Possibility of overcoming the blood-brain barrier with manufactured by us nanostructures was already described [23, 24, 53]. In another study, with intraperitoneally administered superparamagnetic maghemite iron oxide NPs to mice, no accumulation of iron in experimental group was detected, nor within brain or heart tissues, which is inconsistent with our results [52]. Another study, focused on a distribution pattern of iron oxide magnetic NPs within living organism, indicated similar circulation mechanism of nanostructures as in our study—increased level of Fe within the brain and heart after administration of NPs [51]. Increased level of iron in heart tissue may be caused by presence of ZnO:Fe NPs in the general blood stream and consequently its higher level within the tissue. Likewise, higher level of iron within a skeletal muscle detected in AAS analyses is probably related with intensive blood circulation within this muscle and in case of small intestine, the improved content of iron is caused by residues of orally administered NPs.
结论
In conclusion, we performed the preliminary study on the distribution of biodegradable ZnO:Fe NPs as a perspective, new supplementation strategy in iron deficiency. Deposition of iron in the body of mice following oral administration of the nanostructures was detected within the crucial tissues, where the major storage site of the element takes place. We assumed that obtained in the study results might indicate the biodegradable ZnO:Fe NPs as a good carriers of exogenous iron in the living body. However, further research is needed to completely understand the exact mechanisms of the deposition, elimination, and influence of ZnO:Fe NPs on the body.
数据和材料的可用性
The conclusions of the following study are based on the data presented in this manuscript.
缩写
- NP:
-
纳米粒子
- ZnO:Fe NPs:
-
Zinc oxide nanoparticles doped with iron
- IG:
-
Intra-gastric
- ROS:
-
活性氧
- SEM:
-
扫描电镜
- PL:
-
Photoluminescence spectroscopy
- CL:
-
Cathodoluminescence spectroscopy
- DLS:
-
动态光散射
- Caco-2 cells:
-
Caucasian colon adenocarcinoma cells
- DMEM:
-
Dulbecco’s modification of Eagle’s medium
- FBS:
-
胎牛血清
- NEAA:
-
Non-essential amino acids
- PSN:
-
Penicillin–streptomycin–neomycin
- EDTA:
-
乙二胺四乙酸
- AAS:
-
Atomic absorption spectrometry
- SEM (in statistical analysis):
-
Standard error of the mean
纳米材料
- 与铜原子的心脏协调一致
- 将视觉数据与物联网集成的潜力
- 氧化铜纳米颗粒对大肠杆菌的生物合成、表征和抗菌潜力评估
- 131I 追踪的 PLGA-脂质纳米颗粒作为靶向化疗治疗黑色素瘤的药物递送载体
- 二氧化钛纳米颗粒对小鼠的潜在肝脏、大脑和胚胎毒性
- 合成单分散二元 FePt-Fe3O4 纳米粒子的后处理方法
- 通过腹膜内和静脉内给药途径对大鼠生物合成铜和氧化锌纳米颗粒的体内比较检查
- 金属和金属氧化物纳米粒子的绿色合成及其对单细胞藻类莱茵衣藻的影响
- 氧化锌纳米粒子的特性及其对微生物的活性
- 嵌入TiO2致密层的不同尺寸和浓度的Ag纳米颗粒对钙钛矿太阳能电池转换效率的影响
- 使用极浓缩锌源在水/甘油中制备纳米级氧化锌的简便方法
- 基于紫杉醇的靶向脂质纳米颗粒的抗增殖和细胞凋亡触发潜力,通过转铁蛋白受体增强细胞内化——白血病细胞研究