黑磷纳米颗粒通过上调 TG2 表达促进 EMSCs 的成骨分化
摘要
在生物安全浓度下,黑磷纳米粒子激活TG2,促进ECM的表达,进一步促进了EMCs的成骨分化。从这些结果,我们可以得出结论,黑磷纳米粒子适合作为骨组织工程中的生物因子。黑磷纳米粒子 (BPs) 表现出优异的生物相容性和良好的生物降解性,这已经过严格的研究和证明。然而,其在骨组织工程领域的应用仍处于起步阶段。因此,本研究的主要目的是研究 BPs 对体外外胚层间充质干细胞 (EMSC) 成骨分化的影响。用简单有效的超声技术制备了具有高产率的生物相容性 BPs。从成年大鼠鼻呼吸道粘膜中分离出 EMSCs。然后,我们在体外用不同浓度的 BPs 处理 EMSCs,并检查了 BPs 对 EMSCs 成骨分化的影响。此外,转谷氨酰胺酶2抑制剂(TG2)和蛋白质印迹被用来阐明BPs促进成骨作用的机制。我们的结果表明,BPs 可以显着增强体外 EMSCs 的成骨分化。尽管如此,BPs 对 EMSCs 增殖没有影响。从机制上讲,BPs 通过上调 TG2 表达促进 EMSCs 的成骨分化。这些结果突出了使用化学材料对这些极具前景的小分子进行骨组织再生的新型工程策略的优势。
介绍
在临床环境中,即使是普通骨折,骨再生不足也可能导致预后不良,因此迫切需要骨再生材料[1]。随后,许多治疗策略,如自体移植、同种异体移植和人工骨支架已被用作再生材料。近年来,生物材料已被成功证明有助于骨再生,这表明生物材料在多样化应用方面取得了令人瞩目的进展[2]。然而,仍然迫切需要开发一种具有优良骨传导、骨诱导和骨整合的人造骨替代品。生物活性聚合物 [3,4,5] 和陶瓷 [6] 已被制成用于骨工程的支架,通过释放离子 [7] 来增强成骨的支架尤其受到关注。值得注意的是,特异性作用于靶细胞或组织的无机磷酸盐可以为研究矿化相关的生物过程和推进仿生矿化引导的医学工程提供一条有前途的途径[8]。
2014 年,Li 及其同事报告说,黑磷 (BP) 纳米片可以从大块 BP 上剥离,并证明了 BP 纳米片作为一种用于纳米电子器件的新型二维 (2D) 材料的潜力[9]。由于 BP 的优越特性,例如层中独特的褶皱结构、可调节的直接带隙、高载流子迁移率和许多有趣的层内各向异性,BP 目前正在研究其潜在的生物医学应用 [10]。由于这些优点,在过去的 2 年中,基于 BP 纳米材料的支架刺激骨再生得到了广泛的研究。先前的研究表明,磷基生物材料可以通过增加磷酸根离子的局部浓度来增强矿化和骨再生[11]。虽然 BP 可以被认为是促进骨再生的理想候选者,但它通常被封装在聚合物中或通过浸入将其引入生物材料支架上,以供骨组织工程师使用。迄今为止,BP调节骨再生的分子机制在很大程度上仍不清楚,从而阻碍了基于BP的骨修复疗法在临床上的进一步发展。
间充质干细胞 (MSC) 是多能基质细胞,具有进行自我更新和多向分化的能力 [12]。骨折后,间充质干细胞在体内骨修复过程中发挥关键作用 [13,14,15]。多年来,人们对骨髓干细胞 (BMSC) 及其成骨分化潜力进行了广泛的研究。然而,BMSCs 的收获过程对提供者来说可能是痛苦的,并且存在感染风险。外胚层间充质干细胞 (EMSC) 起源于胚胎发育过程中的颅神经嵴,可以很容易地从成人鼻腔的呼吸道粘膜中获取。此外,EMSCs 具有多向分化潜能的自我更新能力,这在我们之前的研究中已经得到了彻底的表征 [16, 17]。我们已经证明 EMSCs 可以分化为多种细胞谱系,包括脂肪细胞、神经细胞、软骨细胞和骨细胞 [18, 19]。这些特殊的特性使 EMSCs 成为探索通过化学信号(包括 BPs)调节 EMSCs 成骨分化的潜在分子机制的有前途的工具。然而,成骨分化是一个复杂的过程,涉及不同细胞增殖和分化、细胞外基质 (ECM) 的合成和矿化的紧密协调 [20]。以往的研究报道,转谷氨酰胺酶2(TG2)能够通过影响成骨细胞增殖、分化、细胞外基质产生和矿化来刺激成骨细胞成骨[21,22,23]。
上面引用的报告表明,BPs 具有巨大的生物医学应用潜力,可以成为 EMSCs 的优良成骨分化诱导剂。到目前为止,关于 BPs 对 EMSC 分化影响的研究尚未见报道。本研究的主要目的是研究 BPs 对 EMSC 相关体外成骨分化和增殖的影响。在那次调查之后,还仔细研究了 BPs 对 EMSC 增殖和成骨分化的潜在分子机制。总体而言,我们的数据表明,BPs 可能是组织工程支架的潜在有用化学试剂。
材料和方法
黑磷纳米颗粒
散装 BP 购自南京 XFNANO 材料科技有限公司。 二甲基亚砜 (DMSO)、氢氧化钠 (NaOH) 和 N -甲基-2吡咯烷酮(NMP)由阿拉丁实业有限公司提供。磷酸盐缓冲盐水(PBS)由北京阳光生物科技有限公司提供。Dulbecco改良的Eagle培养基/营养混合物F12(DMEM/F12; Hyclone;GE Healthcare Life Sciences)、链霉素、青霉素、胎牛血清(FBS),购自BI科技有限公司
BP 合成与之前的报告中的相似,只是稍作修改 [24]。首先,将 20 毫克散装 BP 浸入饱和的 NaOH/NMP 溶液中,并用研磨机械方法精制。然后将混合物以 1500 rpm 离心 10 分钟,然后丢弃沉淀物。然后,将混合物在冰/水浴中超声处理 6 小时,然后将混合物通过 100 μm BD Falcon 过滤器(Becton Dickinson,Sunnyvale,CA)过滤。最后,进一步离心悬浮液(10 分钟,13,000 rpm,4°C),收集沉淀。
鼻粘膜 EMSC 的免疫荧光染色
为了在体内显示 EMSCs,解剖鼻中隔的呼吸道粘膜,然后在 4% PFA 中固定过夜进行免疫荧光染色。组织用PBS洗涤,然后用梯度蔗糖溶液脱水。将组织包埋在 OCT(Sakura Finetek,日本)中进行冷冻切片,并使用 Leica 冷冻切片机以 25 毫米的厚度将组织切成冠状连续切片。这些切片在 PBS 中洗涤 10 分钟,并用 1% 牛血清白蛋白和 0.1% Triton-X 100 的 PBS 进行透化。鼻粘膜中的 EMSC 用抗巢蛋白的一抗和 Cy3 结合的二抗进行免疫荧光染色。平行阴性对照在没有初级抗体的情况下进行相同的程序。染色后的组织在免疫荧光显微镜(AxioObserver,ZEISS,Germany)下观察。
细胞培养
所有动物程序均经江南大学动物护理与伦理委员会批准,本研究严格遵守国际动物研究指南。根据我们之前的研究,从大鼠呼吸道黏膜中分离出原代外源间充质干细胞 [17, 25]。简而言之,通过腹膜内注射戊巴比妥钠 (0.05 g/kg) 麻醉 100 g 成年 Sprague Dawley (SD) 大鼠。将鼻中隔的中间三分之一切碎,然后在 0.25% 胰蛋白酶溶液(Hyclone;GE Healthcare Life Sciences)中在 37°C 下孵育 25 分钟,然后小心地剥离鼻中隔粘膜。最后,将鼻中隔粘膜组织切成碎片。所得组织悬浮液通过 100 微米尼龙网筛,以 1000 克离心 5 分钟,然后用磷酸盐缓冲盐水 (PBS) 洗涤两次。将组织悬浮液置于生长培养基(DMEM/12 含有 10% FBS、100 U/ml 青霉素和 100 μg/ml 链霉素)中的细胞培养瓶中,并在 37°C、5% CO2 和 95% 空气中培养饱和湿度。每三天更换一次培养基,每周传代细胞一次。第三代细胞用于所有表征研究。使用针对波形蛋白和巢蛋白等干细胞标志物的抗体对培养的细胞进行免疫荧光表征[26]。
EMCs多向分化能力的鉴定
将 EMSC 接种在 6 孔板中并用 DEME/F12 培养基培养 24 小时至 70% 汇合。然后用成骨分化培养基(补充有 10% FBS、0.1 mM 地塞米松、10 mM β-甘油磷酸二钠和 0.2 mM l-抗坏血酸的 DMEM)更换培养基以诱导成骨。每 7 天更换一次培养基,在第 28 天用 0.5% 茜素红 S (Sigma-Aldrich) 进行茜素红 S 染色。将长至 90% 汇合的 EMSC 在成脂分化培养基中培养以诱导脂肪生成 21 天。油红染色按照厂家说明书使用油红染色试剂盒(Solarbio)进行。
BP 的特征
扫描电子显微镜 (SEM) 用于表征 BP 的形态和大小。通过将一滴浓度为 1mg/ml 的去离子水中的 BP 溶液置于涂有 formvar 的铜网上,然后风干来制备样品。通过扫描电子显微镜(H-7500;Hitachi,Tokyo,Japan)对样品进行拍照。使用图像 Pro Plus 软件(Media Cybernetics Inc.,MD,USA)分析 BP 的平均大小。 zeta 电位的评估由 Malvern zeta sizer (ZEN3600) 证实。 X 射线衍射 (XRD) 使用 Bruker D8 Discover 衍射仪在 Bragg-Brentano 对位聚焦几何结构和 Cu Kα 辐射中进行。在 2θ 的 10° 和 80° 之间收集衍射图案 步长为 0.01° 2θ 和每步 0.2 秒的采集时间。使用 HighScore Plus 3.0e 软件评估所得数据。具有 514 nm 激光激发的拉曼光谱仪 (LabRam HR800) 用于测量样品的拉曼光谱。通过X射线光电子能谱([XPS] Omicron NanoTechnology GmbH,Germany)测量样品的表面成分。
细胞计数套件-8 (CCK-8) 分析
使用细胞计数试剂盒 (CCK-8) 测定法评估 BPs 对细胞增殖的影响。简而言之,将 EMSC(3000 个细胞/孔)接种在 96 孔板中并在 37 小时用 BP(0、1、2、4、8、16、32、64、128 和 512 μg/ml)处理 24 ℃。对于 CCK8 检测,在分析前 4 小时将 10 μl CCK8 试剂添加到培养基中。根据制造商的说明,使用酶标仪(Thermo Fisher Scientific,马德里,西班牙)测量 450 nm 处的光密度(OD)。用于进一步研究的 BPs 浓度是根据 CCK-8 测定的结果选择的。对于 Ki-67 染色,EMSCs (1 × 10 4 ) 在 24 孔板中培养,并通过 Ki-67 的免疫荧光染色(兔多克隆;目录号 ab15580;abcam;1:300)。 DAPI 用于染色细胞核。图像由荧光显微镜(放大倍数,200 倍;eclipse Ti;nikon 公司)捕获,并用 Image Pro Plus 软件进行分析。
成骨分化
对于成骨分化,以 3,000 个细胞/cm 2 的密度接种 EMSCs 并在生长培养基中培养至 70% 汇合。然后用成骨分化培养基诱导细胞2周。值得注意的是,为了避免 β-甘油磷酸钠的影响,成骨分化培养基补充有 10% FBS、0.1 mM 地塞米松和 0.2 mM l-抗坏血酸(Sigma-Aldrich(St. Louis, MO, USA),但不与β-甘油磷酸钠。诱导分化后,碱性磷酸酶(ALP)和茜素红染色和RT-qPCR用于评估BPs对EMSCs成骨分化的任何影响。EMSCs以2 × 10<的密度接种sup> 5 细胞/孔在 6 孔板中,并与成骨培养基一起孵育。根据制造商的说明,在第 14 天使用 ALP 染色试剂盒(南京建成生物工程研究所)评估 ALP 染色。应用茜素红 S 染色观察磷酸钙的沉积。简而言之,将固定的细胞再次用去离子水洗涤,并与 1 ml/孔的茜素红染色溶液(0.5% (w/v) 茜素红 S (Sigma-Aldrich) 在 PBS 中)在 37°C 下孵育 10 分钟。除去溶液,再次用去离子水清洗样品,用Image-Pro plus软件对每场显示钙化结节的阳性染色面积进行计数,并归一化为各自的对照。
RT-qPCR
如前所述进行 RT-qPCR。简而言之,根据制造商的说明,使用 RNA 纯化试剂盒(TaKaRa,日本)从单一培养物中分离或分选总 RNA。使用 cDNA 合成试剂盒 (Fermentas) 将两微克 mRNA 逆转录为 cDNA。用于 RT-PCR 的引物由南京金斯瑞生物工程技术服务有限公司(中国南京)设计和构建,如表 1 所示。 RT-PCR 使用 SYBR Green/荧光素 qPCR Master Mix 试剂盒(Fermentas)进行操作使用 ABI Prism 7500 系统。将数据标准化为甘油醛 3-磷酸脱氢酶 (GAPDH) 以指示相对表达水平。所有测量一式三份进行。
图>蛋白质印迹
培养的细胞用 PBS 洗涤两次,然后用含有磷酸酶和蛋白酶抑制剂混合物的 RIPA 裂解缓冲液在冰上裂解 30 分钟。为了收集总 TG2,用细胞刮刀收集 6 孔板中的细胞裂解物和 ECM。用PBS洗涤板,并收集细胞洗涤液。通过以 12,000 × g 离心从上清液中收集裂解物 5 分钟,与等体积的 SDS 上样缓冲液混合,然后煮沸 5 分钟。使用 BCA 蛋白质检测试剂盒 (Beyotime, Shanghai, China, P0012) 测定蛋白质浓度。在 RIPA 裂解缓冲液中提取蛋白质,在十二烷基硫酸钠 (SDS) 聚丙烯酰胺凝胶上分离,并电泳转移到硝酸纤维素膜(Millipore,LA,USA)上。在含有 0.5% Tween 20(索莱宝,北京,中国)的 0.01 M Tris 缓冲盐水 (TBS) 中用 1% 牛血清白蛋白 (BSA) 封闭膜,并用指定的抗体进行印迹,包括抗 TG2 (1:200; sc-48387, Santa Cruz Biotechnology, Inc.)、抗 FN(1:500;货号 BA1772;武汉博斯特生物科技有限公司)和抗 LN(1:500;货号 BM4921;武汉Boster Biological Technology, Ltd.)、抗 OCN (1:200; sc-390877, Santa Cruz Biotechnology, Inc.)、抗 OPN (1:200; sc-73631, Santa Cruz Biotechnology, Inc.)、抗- COL I(1:500;目录号 BA0325;武汉博士德生物科技有限公司),在 4°C 下放置 12 小时。然后将膜与辣根过氧化物酶偶联的山羊抗兔 IgG(1:5000;Boster,武汉,中国)在室温下反应 2 小时。使用 Pierce ECL Plus 底物(Thermo Fisher Scientific)观察蛋白质条带,然后使用化学发光成像系统(Clinx Science Instruments,Shanghai,China)进行扫描。
抑制实验
为了证实 TG2 的参与,对 EMSCs 进行了 TG2 抑制实验。用抗 TG2 中和抗体(来自 BD Biosciences, San Diego, CA)进行中和实验。将细胞接种在具有正常培养基的 6 孔板中 24 小时以使其粘附,并用抗 TG2 抗体 (10 μg/ml) 处理。同时,设置对照组。接种到 6 孔板中 24 小时后,使用含 BPs (2 μg/ml) 的成骨培养基诱导 EMSC 成骨 14 天,每 7 天用新鲜的成骨培养基更换含有抑制剂的培养基。茜素红 S 染色用于观察磷酸钙的沉积。 Western blotting检测骨钙素、骨桥蛋白和1型胶原蛋白水平(分别为OCN、OPN和COL I表达)。
免疫荧光染色
培养的细胞在 4°C 下用 4% 多聚甲醛固定 8 小时。固定的细胞用 PBS 洗涤 3 次,并在含有 0.2% Triton X-100 和 1% BSA(索莱宝,北京,中国)的 PBS 中透化 30 分钟。透化后,这些固定的细胞用 PBS 洗涤,然后与一抗在 4°C 下孵育 8 小时,然后去除所有一抗。然后用 PBS 洗涤细胞 3 次,并在室温下用二抗 Alexa Fluor-594(1:800,Life Technologies Molecular Probes,Carlsbad,CA,USA)在 37°C 下孵育 2 小时。细胞核用 4'6-二脒基-2-苯基吲哚([DAPI];Sigma-Aldrich)复染。所有试验组均在免疫荧光显微镜下观察。
统计分析
数据从上述三个单独的实验中获得,并表示为平均值 ± 标准偏差(SD)。使用学生的 t 对数据分布进行分析 -检验以分析处理组和对照组之间差异的显着性。进行一种方差分析 (ANOVA),然后进行 Tukey 事后检验,以评估组间的显着差异。 p <0.05 被认为具有统计学意义。
结果
BP 的特征
通过动态光散射 (DLS) 测量的 BP 尺寸分布在 100 到 200 nm 的范围内相对较窄,峰值在 132 nm。结果如图 1A 所示。 BPs 的 zeta 电位测定为 − 23.7 ± 0.65 mV(图 1B),证实了 BPs 的稳定性。通过扫描电子显微镜 (SEM) 进一步研究颗粒的尺寸(图 1C)。结果与DLS对粒度分布的测量吻合良好。
<图片>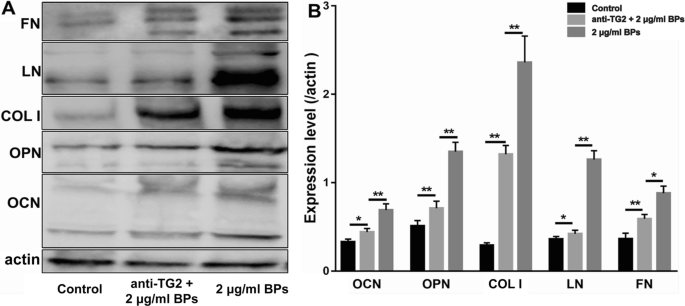
BP 的表征。 A 黑磷纳米粒子 (BPs) 的尺寸分布; B zeta 电位为 − 23.7 ± 0.65 mV 的 BP 的 zeta 电位报告; C BP纳米片(BPN)的扫描电子显微镜(SEM)图像。 D BPs的拉曼光谱; E X 射线衍射图和 F BPs的P 2p高分辨率X射线光电子能谱(XPS)光谱; G BPs的XPS光谱
图>拉曼散射(图 1D)在 362.3、438.5 和 466.9 cm -1 处显示了三个突出的峰 与面外声子模式 (A 1 g) 和两个面内模式 (B 2 g 和 A 2 g) BP,分别为 [17, 18]。 XRD(图 1E)显示了所制备材料的相纯度,平均结晶尺寸为 102.7 nm。衍射图案的加宽表明单个微晶的纳米尺寸。 P 2 p 的高分辨率 XPS 光谱(图 1F)显示了 130 eV 处的主峰,对应于黑磷的 P-P 键,此外还有一个位于 135 eV 处的附加峰,该峰源于表面氧化引起的 P-O 键BPs。通过宽扫描 X 射线检查 BP 表面(图 1G)。
EMSC 的特征
鼻粘膜免疫荧光染色显示巢蛋白阳性的 EMSCs 位于鼻中隔呼吸上皮下的固有层(图 2A)。第三代培养的 EMSC 表现为成纤维细胞样细胞,并在塑料板上快速增殖(图 2B)。我们在第 28 天通过茜素红染色评估了成骨培养基中的成骨分化(图 2C)。在第 21 天通过油红-O 溶液染色评估脂肪形成诱导培养基中的脂肪形成分化(图 2D)。免疫荧光染色显示几乎所有 EMSC 都表达神经嵴细胞标志物,例如图 2E 所示的巢蛋白 (> 95%) 和图 2F 所示的波形蛋白 (> 95%)。干细胞标志物的共表达结果表明这些EMSCs是一种间充质干细胞。
<图片>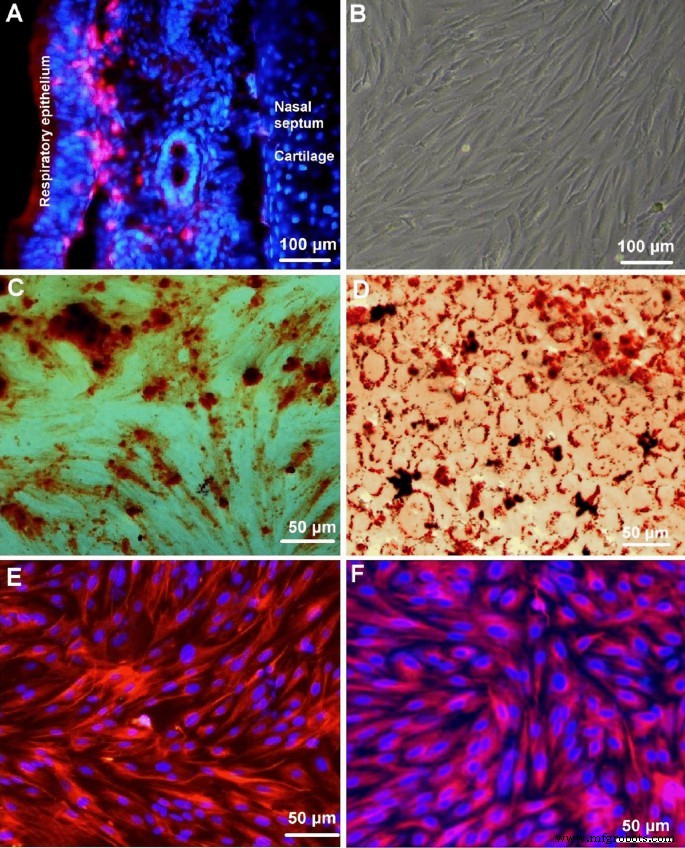
外胚层间充质干细胞 (EMSC) 的鉴定。 A 巢蛋白阳性的 EMSCs(cy3,红色)位于鼻中隔呼吸上皮下固有层; B 相差图像显示第三代培养的EMSCs表现为成纤维细胞样细胞,在塑料板上增殖迅速; C 成骨培养基中成骨分化的EMSCs经茜素红染色; D 成脂诱导培养基中成脂分化的EMSCs用油红-O染色; E , F 神经嵴细胞标志物的免疫荧光染色显示几乎所有的 EMSC 都表达巢蛋白和波形蛋白。 IgG-Cy3(红色)作为免疫荧光染色的二抗,细胞核用Hoechst 33342(蓝色)复染
图>细胞毒性
作为初步实验,进行了 CCK-8 测定以评估 BPs 的细胞毒性。暴露于 BPs 24 小时后 EMSCs 的生存能力如图 3 所示。在暴露于高达 32 μg/ml BPs 后没有发现显着的细胞毒性。然而,暴露于 BPs 会导致更高剂量(> 32 μg/ml)的显着细胞毒性。在低剂量组中观察到核仁和染色体中的 Ki-67 表达(图 4A-C)。然而,与未处理的对照相比,低剂量 BP 处理的 EMSC 中 Ki-67 阳性细胞核与细胞核总数之间的比率没有显着差异(图 4D)。因此,在本研究中,我们在以下未观察到显着细胞毒性的实验中选择浓度为 2 和 4 μg/ml 的 BPs。
<图片>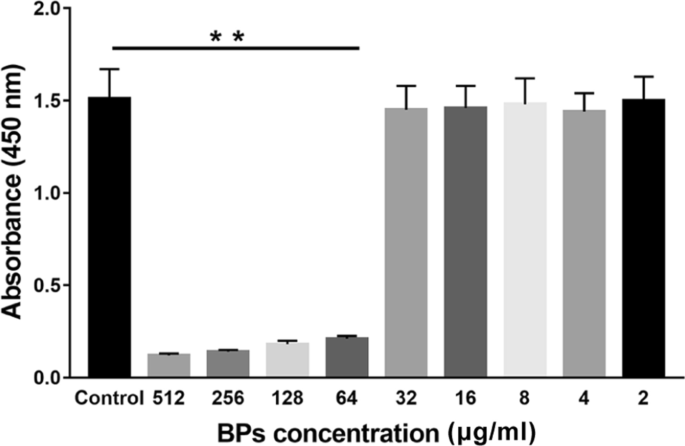
BPs的细胞毒性。细胞用 BPs(0、2、4、8、16、32、64、128、256 和 512 µg/ml)处理 24 小时,并通过细胞计数试剂盒 (CCK-8) 增殖测定 (n =9)。数据表示为平均值 ± 标准偏差(SD)。 **p <0.01.
图> <图片>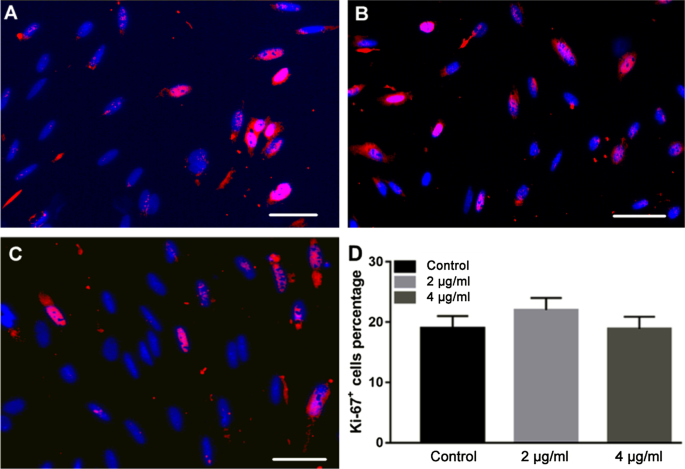
BPs处理组细胞增殖的评价。 EMSC 用 BP(0、2 和 4 μg/ml)处理 24 小时。用抗 Ki67 抗体(红色)和 DAPI(蓝色)通过免疫荧光染色测量细胞增殖,并显示合并图像(A –C )。 4',6-二脒基-2-苯基吲哚(DAPI)阳性细胞中的 Ki67 阳性细胞在三个板中的每一个中的两个高倍视野中计数。数据表示为平均值 ± SD (D ).
图>茜素红 S 染色
如图 5A 所示,在用条件培养基处理 14 天后,通过茜素红 S 染色评估细胞中的矿化。三组均可见钙结节,对照组可见未成形的钙结节。与对照组相比,2、4 μg/ml 组沉积的钙结节较大(p <0.05),但治疗组之间没有观察到明显差异(p> 0.05),如图 5C 所示。
<图片>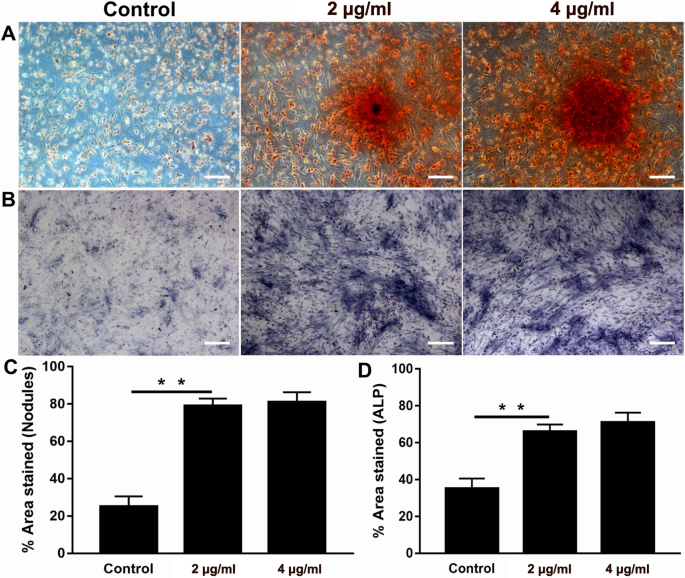
碱性磷酸酶 (ALP) 和茜素红染色测定。 A 成骨分化的 EMSCs 在第 14 天用茜素红溶液染色; B ALP染色在显微镜下可见; C 图表显示了茜素红沉积区域的量化; D BP 处理的 EMSCs 组的 ALP 活性显着高于对照组。数据表示为平均值 ± SD。 **p <0.01
图>ALP 测试
进行 ALP 染色以研究 EMSCs 的成骨分化。与对照组相比,2 和 4 μg/ml BP 组的 EMSC 颜色更深(图 5B),表明添加 BP 导致 EMSC 中 ALP 表达的增强。 BPs处理的细胞在第7天的ALP活性高于对照组(p> 0.05),但未观察到 2 和 4 μg/ml 之间的显着差异(p> 0.05),如图 5D 所示。
RT-qPCR
如图 6 所示,在第 14 天分析了主要分化标志物,包括矮小相关转录因子 2 (RUNX 2)、ALP、COL 1、OCN、OPN 和骨形态发生蛋白 2 (BMP-2)。在第 7 天,在三组细胞中发现了所有这些基因标记。未发现 2 和 4 μg/ml 组之间的基因表达存在明显差异。然而,与对照细胞相比,上述基因在BP处理的细胞中的表达显着增加。
<图片>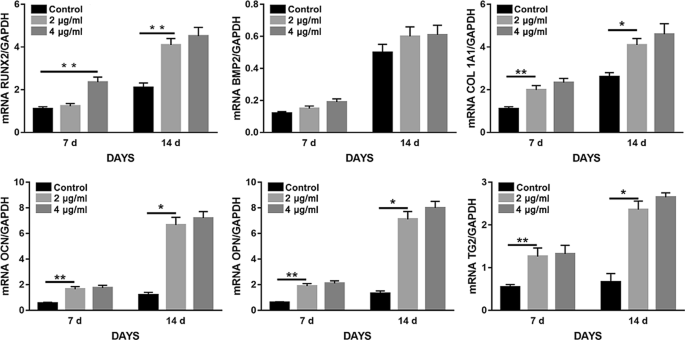
BPs 增强 EMSCs 的成骨作用。 EMSC 在含有 0 μg/ml、2 μg/ml、4 μg/ml BPs 的成骨培养基中生长 14 天。通过定量逆转录聚合酶链反应 (RT-qPCR) 对参与 EMSCs 成骨的基因表达进行量化。 **p <0.01, *p <0.05.
图>BPs 通过上调 TG2 表达增强 EMSCs 的成骨
由于 TG2 对多种细胞的整合素介导的分化和 ECM 沉积具有显着影响,我们研究了 BP 是否会通过上调 TG2 表达促进 EMSCs 的成骨分化。如图 7 所示,我们检测到 BP 处理组中细胞内和细胞外 TG2 的明显上调。 2 和 4 μg/ml BP 处理的细胞中的层粘连蛋白 (LN) 和纤连蛋白 (FN) 的水平高于对照组(图 7A)。与对照组相比,暴露于 BP 的 EMSC 的 TG2 水平增加了大约两倍,但在 BP 治疗组之间没有观察到显着差异(图 7B)。作为一种大分子蛋白,抗TG2抗体无法穿过细胞膜。 Thus, the extracellular TG2 was blocked by the antibody and failed to crosslink various growth factors and ECM proteins. The Alizarin Red S (Fig. 8A, C) results showed that anti-TG2 markedly suppressed the BP-mediated increase in calcium and ECM deposition. Meanwhile, as shown in Fig. 8D, anti-TG2 significantly inhibited BP-treated cells’ increase in ALP activity. Moreover, anti-TG2 effectively abolished the effects of BPs on the bone matrix proteins expression, including OCN, OPN, and COL I (Fig. 9).
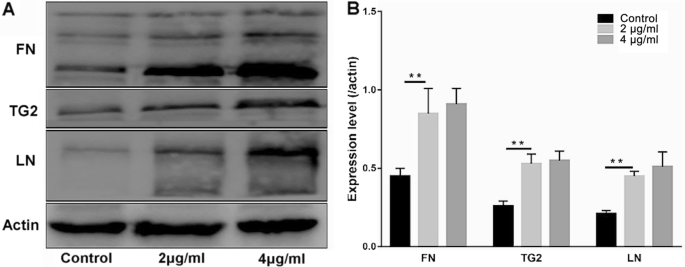
Transglutaminase 2 (TG2) was involved in the osteogenic differentiation of EMSCs. EMSCs were incubated with BPs (2 and 4 μg/ml) for 14 days, and fibronectin (FN), LN, and TG2 levels were assessed by immunoblotting. Data were expressed as mean ± SD. **p < 0.01; *p < 0.05.
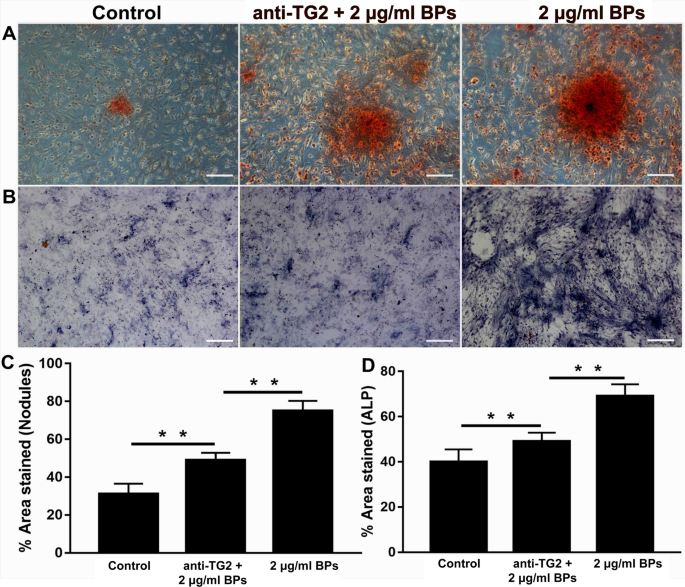
Osteogenic differentiation of EMSCs with TG2 neutralizing antibody. A ALP staining. B ARS staining performed to examine extracellular mineralization. C Quantitative ALP analysis. D Quantitative Alizarin red staining analysis. **p < 0.01
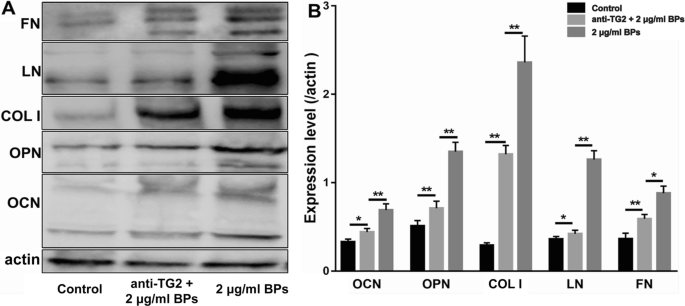
Anti-TG2 inhibited BP-induced EMSC osteogenic differentiation. A Representative western blots of osteoponin, osteocalcin, and collagen 1 (OPN, OCN, LN, FN, and COL I, respectively) in differentiated EMSCs following treatment with 2 μg/ml BPs and 2 μg/ml anti-TG2 or 2 μg/ml BPs alone. B Quantification of OPN, OCN, and COL I protein expression. Data were expressed as mean ± SD. **p < 0.01; *p < 0.05
Discussion
To the best of our knowledge, modification of phosphorus was first exhaustively studied as early as 1914; unfortunately, these studies did not receive much attention for an entire century [27]. Phosphorus is one of the essential elements making up about 1% of the total body weight as a bone component in the human body [28], while most of the other materials cannot warrant such a natural biocompatibility. In 2014, BP was introduced as a new member of the 2D layered materials.
In this study, the BP nanoparticles were prepared on a large scale from bulk BP crystals by using an improved mechanical grinding and continuous ultrasound method. Compared with other methods, this ultrasound and mechanical grinding synthesis is facile and efficient and enables production of BPs on a large scale. For characterization of BPs, SEM was carried out to examine the morphology of BPs. SEM images illustrated that BPs were successfully synthesized, and the typical sizes of BPs are about 100 to 150 nm. The size of particles was further investigated using DLS in the relatively narrow range around 133 nm. The result of SEM corresponded well with the measurement of particle size measurements based on DLS. Interestingly, previous studies demonstrated that BP with larger lateral size has higher cytotoxicity than small BP, while ultra-small BPs were even considered nontoxic up to the high concentration of 1000 μg/ml [29, 30]. Thus, for biomedical applications, the BP nanomaterials still need further study. In addition, Raman scattering revealed the presence of three prominent peaks at 361.5, 437.1, and 465.2 cm −1 that were associated with the out-of-plane phonon mode (A 1 g) and two in-plane modes (B 2 g and A 2 g) of BPs. These results were consistent with previous reports [31] in which showed that the BPs had been prepared successfully from bulk BP. XRD shows the phase purity of the prepared BPs. Broadening of the diffraction pattern indicates the nanometer size of individual crystallites.
To evaluate the stability and degradation rate of BPs, Wang and co-workers performed an experiment in which normalized absorption spectra of the BP nanosheets were dispersed in water and exposed to air. After 6 days, they found that the absorbance of the BP nanosheets at 450 nm decreased by 43% compared to the initial value, indicating that BP is easily degraded in the physiological environment [32]. Also, it is well-known that BP is sensitive to water and oxygen, but this shortcoming is considered a merit for biomedical applications. Because of these special characteristics compared with other biomaterials, BPs could potentially avoid material accumulation in human body and then reduce cytotoxicity caused by such material noumenon.
Since MSCs play key roles in bone formation, there is no doubt that transplanting MSCs in animal models of bone defects enhance bone regeneration and promotes functional recovery via BMSC acquisition [33]. Unfortunately, BMSC acquisition procedures are painful for the donor and frequently cause surgical site infection, and the number of harvested BMSCs is low [34]. Previous studies from our laboratory and other reports have shown that EMSCs could be isolated from several adult tissues, such as dental pulp and the nasal mucosa, without causing invasive injury. Moreover, EMSCs were easily induced into osteoblasts, rendering those cells as promising seed cells for bone tissue engineering. Therefore, EMSCs were used in this study. We attempted to obtain the maximum safety BP concentration for EMSCs. As shown in the results, we achieved the maximum safe concentration of BPs (less than 64 μg/ml). The concentrations of 2 and 4 μg/ml of BPs were chosen for further study to avoid any possible potential toxic. The Ki-67 assay was also used to quantify and evaluate EMSC proliferation in these samples after treating these cells with BPs (2 and 4 μg/ml) for 24 h. We did not observe any statistically significant suppression of EMSCs growth in the case of BPs. Meanwhile, our results indicate that during treatment with safe concentrations of BPs, promotion of EMSCs proliferation also occurred.
Some reports have shown that the concurrent binding of BP and calcium ions may benefit osteogenic differentiation, thereby leading to enhanced bone regeneration [35, 36]. To investigate these effects, in vitro EMSCs exposed to BPs were used in osteogenic differentiation experiments. Interestingly, the results of the ALP test and Alizarin Red S staining show that exposure to BPs could promote rather than compromise osteogenetic differentiation of EMSCs compared to the control group. Similar findings have been reported in which phosphorus-rich materials can stimulate mineralization and bone regeneration. Co-expression of osteogenesis-related genes, including ALP, OPN, OCN, COL1, and RUNX2, in differentiated EMSCs was detected at days 7 and 14 by RT-qPCR. Indeed, the expression levels of these osteogenic genes significantly increased in differentiated EMSCs treated with BPs, also providing confirmation of the osteogenic potential of the BPs. Similar results were reported in which BP could induce both the proliferation and osteogenic differentiation of human pre-osteoblast cells. Together these results indicate that the BPs were able to induce osteogenic differentiation of EMSCs.
It can be asked, “How do BPs promote osteogenetic differentiation of EMSCs?” It is well accepted that phosphorus can capture Ca 2+ in vivo to form calcium phosphate deposits that accelerate bone regeneration, while BP can be the resource of phosphorus ions [36]. Tong et al. believes that BP generate mild heat (40–42 °C), which causes up-regulation of heat shock protein (HSPs) expression and stimulates bone regeneration [37, 38]. Moreover, in this study, the upregulated activation of TG2 levels was also observed in BPs treatment groups. To the best of our knowledge, TG2 has important enzymatic and non-enzymatic functions at these locations in which it crosslinks various ECM proteins and modulates the interactions of cells with the ECM and soluble growth factors by non-covalent interactions with and regulation of integrins [39,40,41]. Obviously, the BPs can react strongly with oxygen and water and finally degrade to non-toxic phosphate in aqueous solutions, which is a crucial component of ATP. Nakano Y et al. reported that ATP can act as a significant phosphate (Pi) source for mineralization in MC3T3-E1 osteoblast cultures, indicating that ATP-hydrolyzing enzymes could induce mineral deposition [42]. They also found that TG2 could not only act as a phosphatase but could be involved in ATP hydrolysis in the osteoblast cultures thus further contributing to the elevation in Pi levels required for mineral deposition, which may be beneficial to EMSC energy metabolism. This process may be part of the contribution that BPs makes toward enhancement of EMSC osteogenic differentiation. On the other hand, thanks to TG2 bio-functions, a wide variety of ECM adhesion proteins, including LN, COL I, and FN, could maintain a stable state. Indeed, in the present study, we observed that ECM (FN, COL I, and LN) were significant higher in BP-treated EMSC groups. BPs provide a favorable ECM microenvironment for promoting greater osteogenic EMSC differentiation and proliferation.
Until now, no secretory signal sequences and hydrophobic or transmembrane domains have been clearly identified in TG2 because the protein is not localized in the endoplasmic reticulum (ER)/Golgi compartments [39, 43], and only few studies reported about these factors that control TG2’s secretion. Therefore, it remains unclear as to the exact mechanism of BP regulation of expression patterns of TG2 in the progress of EMSC osteogenic differentiation.
Conclusion
In the present study, BPs were successfully fabricated using mechanical grinding and continuous ultrasound method. At bio-safe concentrations, BPs activated TG2, and promoted the expression of ECM, which further promoted osteogenic differentiation of EMSCs. From these results, we can conclude that BPs would be suitable for incorporation into tissue-engineered scaffolds that utilize EMSCs to repair bone defects. Although our research highlights the great potential of BPs in nano-biomedicine, large-scale preclinical and clinical studies concerning its safety are needed before any clinical applications are established.
Availability of Data and Materials
The datasets generated during and/or analyzed during the study are available from the corresponding author on reasonable request.
Abbreviations
- BPs:
-
Black phosphorus nanoparticles
- EMSCs:
-
Ectodermal mesenchymal stem cells
- TG2:
-
Transglutaminase 2
- 2D:
-
Two-dimensional
- ECM:
-
Extracellular matrix
- DMSO:
-
Dimethyl sulfoxide
- NMP:
-
N -Methyl-2pyrrolidone
- PBS:
-
Phosphate-buffered saline
- DMEM/F12:
-
Dulbecco's modified Eagle's medium/nutrient mixture F12
- FBS:
-
Fetal bovine serum
- SD:
-
Sprague Dawley
- SEM:
-
Scanning electron microscopy
- XPS:
-
X-ray photoelectron spectroscopy
- CCK-8:
-
Cell counting kit
- OD:
-
Optical density
- RT-qPCR:
-
Reverse transcriptase polymerase chain reaction
- GAPDH:
-
Glyceraldehyde 3-phosphate dehydrogenase
- BSA:
-
Bovine serum albumin
- TBS:
-
Tris-buffered saline
- DAPI:
-
4′6-Diamidino-2-phenylindole
- DLS:
-
Dynamic light scattering
纳米材料