羟基磷灰石纳米颗粒通过内皮细胞相互作用对间充质干细胞的潜在骨诱导作用
摘要
纳米羟基磷灰石(nano-HA)在再生医学领域引起了广泛关注。内皮细胞 (EC)-间充质干细胞 (MSC) 相互作用是骨骼重建所必需的,但纳米 HA 在此过程中的相互作用方式仍然未知。在此,我们使用由 ECs 介导的间接共培养模型研究了 HA 纳米粒子 (HANPs) 对 MSCs 的细胞毒性和骨诱导作用,并强调了其潜在机制。结果发现,在亚细胞毒性剂量下,HANP 增加了成骨细胞基因的活力和表达,以及 MSC 的矿化结节和碱性磷酸酶的产生。这些现象依赖于 ECs 分泌的 HIF-1α,它触发了 ERK1/2 信号级联。此外,建立两阶段细胞谱系数学模型,定量分析HIF-1α对MSCs成骨分化的影响。结果表明,HIF-1α对MSCs的成骨分化率具有剂量依赖性刺激作用,最高可达1500 pg/mL,与上述结果一致。我们的数据表明 HANP、EC 和 MSC 之间的协同相互作用可能有助于刺激骨再生。此外,两阶段细胞谱系模型有助于评估效应分子在骨组织工程中的潜在影响的体外系统。
介绍
外伤、先天性畸形或手术切除引起的骨缺损的重建对骨科手术提出了巨大的挑战[1]。羟基磷灰石 (HA) 是一种代表性的生物活性陶瓷,已被用作骨替代品 [2]。然而,不良的机械和骨诱导特性限制了其临床应用[3]。近年来,nano-HA由于其独特的仿生特性表现出更优的生物活性和更好的机械性能,并在与再生医学相关的生物医学领域引起了极大的兴趣[4]。当nano-HA被植入骨缺损时,参与骨修复的多个细胞将暴露于其中。因此,有必要评估纳米 HA 的生物学行为。几条证据直接表明 HA 纳米粒子 (HANPs) 可以被人脐带沃顿的果冻间充质干细胞 (hWJ-MSCs) 和成骨细胞吸收,从而增强成骨分化 [5,6,7]。杜阿等人。先前报道了 HANP 促进工程软骨整合到从头软骨中的能力 [8];相反,HANP 抑制人脐静脉内皮细胞 (HUVEC) 的血管生成能力 [9]。在人类健康方面,有必要更全面地了解 HANPs 对骨再生的影响,而工程纳米人工骨的持续应用增加了此类研究的紧迫性。
骨再生不可避免地伴随着新生血管的侵袭。 ECs 是血管系统的内部细胞衬里,用于被动输送血液,还在诱导、指定和引导器官再生以及维持体内平衡和新陈代谢方面发挥作用 [10, 11]。间充质干细胞是内皮周生态位的一部分,在其驻留生态位内特定的生理和生化微环境的诱导下具有自我更新和多分化能力[12]。蔡等人。发现 ECs 可以分泌内皮素-1 以指导 MSCs 向骨和软骨谱系分化 [13]。此外,萨利赫等人。使用微阵列数据分析来识别与 MSC 膜结合受体相互作用以增强增殖和成骨分化的 HUVEC 分泌蛋白和相关串扰信号通路 [14]。在骨组织工程中,HANP 可以与新血管接触并被 EC 内吞,这已被证明会改变这些细胞的生理功能 [9, 15]。这也可以影响周围的骨祖细胞并通过改变旁分泌信号影响骨骼修复。然而,虽然已经探索了 HANPs 对 MSCs 的直接影响,但对于 HANPs 是否可以通过 ECs 间接诱导 MSCs 的成骨分化仍然缺乏明确的认识,这对于我们理解 HANPs 在这方面的影响至关重要。进行骨修复。
在这项研究中,为了进一步了解 HANPs 对 ECs 和 MSCs 之间相互作用的生物学影响,使用 HUVECs 和 hWJ-MSCs 建立了间接共培养模型。通过利用该系统,评估了 HANP 通过 HUVEC 介导的旁分泌信号传导对 hWJ-MSC 的细胞毒性和骨诱导作用。为了确定影响 HANP 诱导的内皮细胞 - MSC 相互作用的关键因素,评估了用 HANP 刺激的 HUVEC 上清液中的可溶性因子,重点是基因和蛋白质水平的相关机制。结果表明缺氧诱导因子(HIF)-1α在这些相互作用中起关键作用。
为了定量观察和预测 HIF-1α 对成骨过程的影响,建立了一个将两阶段细胞谱系与 HIF-1α 相结合的数学模型。在这里,通过分析经验数据,基于定义的初始细胞接种密度和 HIF-1α 浓度,使用两阶段细胞谱系模型预测任何时间点的 MSC 数量和分化程度,这可能反过来为初始培养条件和孵育时间提供适当的建议。本研究结果将有助于阐明纳米骨替代品与生物系统之间的相互作用,有助于促进用于再生医学的创新生物材料的发展。
材料和方法
颗粒的制备和表征
纯度 ≥ 99.0% 的 20 nm (np20)、20*80 nm (np80) 和微米级 HA 颗粒 (m-HAP) 的 HANP 购自南京英皇纳米材料有限公司(中国南京)。使用透射电子显微镜(TEM;FEI Tecnai G2 Spirit Bio-Twin,FEI,Hillsboro,OR,USA)和扫描电子显微镜(SEM;LEO1530VP,德国)观察颗粒的大小和形状。通过 Zetasizer Nano ZS90 和 Mastersizer 3000 (Malvern Instruments, Malvern, UK) 测定 HA 颗粒 (HAPs) 的流体动力学尺寸和 zeta 电位。
细胞制备和培养
所有实验方案均经南京医科大学伦理委员会批准。如前所述,在获得捐赠者的书面知情同意后,从新鲜人脐带中收集 HUVEC 和 hWJ-MSC [16, 17]。简而言之,用含有 1% 青霉素和链霉素(PS;Hyclone,GE Healthcare Life Sciences,Pasching,Austria)的磷酸盐缓冲盐水 (PBS) 冲洗脐带和脐静脉。然后,脐静脉充满 0.1% 胶原酶 I(Sigma,St. Louis,MO,USA)并在 37°C 下孵育 15 分钟。收集后,将 HUVEC 在 EC 培养基(ECM)(Sciencell,San Diego,CA,USA)中培养。
随后,去除血管,将沃顿氏果冻切成 1 毫米 2 件,然后放置在 25 cm 2 组织培养瓶(Corning Incorporated, Corning, NY, USA)。这些细胞在补充有 10% 胎牛血清 (GIBCO) 和 1% PS 的 L-DMEM (GIBCO Life Technology, Grand Island, NY, USA) 中培养。
使用针对 CD13、CD29、CD34、CD44、CD45、CD51 和 CD105 的单克隆抗体(BD Biosciences,San Jose,CA,USA)评估 hWJ-MSC 以确认表型。使用 von Willebrand 因子(vWF;上海昌道生物科技有限公司,中国上海)评估 HUVEC。在这些实验中使用了第 3-7 代之间的 HUVEC 和第 3-5 代之间的 hWJ-MSC。
然后将 PBS 中 1 mg/mL 的颗粒悬浮液在 L-DMEM 中稀释至最终浓度。如图 1 所示,HUVEC 在指定浓度的 HAP 中孵育 18 小时。将培养基在 4°C 下以 15,000 rpm 离心 15 分钟,将补充有 10% FBS 的上清液用作 hWJ-MSC 的条件培养基 (CM) 以完成以下实验。 CM 由补充有成骨诱导液的成骨培养基组成,其中含有 10 mM β-甘油磷酸、50 μg/mL L-抗坏血酸-2-磷酸和 0.1 μM 地塞米松(Sigma-Aldrich,St Louis,MO,USA)。此外,2-甲氧基雌二醇 (2-MeOE2) (Selleck Chemicals, Houston, TX, USA) 被用作特异性 HIF-1α 抑制剂。在 2-MeOE2(+) 组中,hWJ-MSC 与来自 HUVEC 的 CM 一起培养,在补充 HAP 之前用 5 μM 2-MeOE2 预处理 40 分钟。 PD98059 用作特异性 MEK 抑制剂。在PD98059组中,hWJ-MSCs用含有5 μM PD98059的CM培养。
<图片>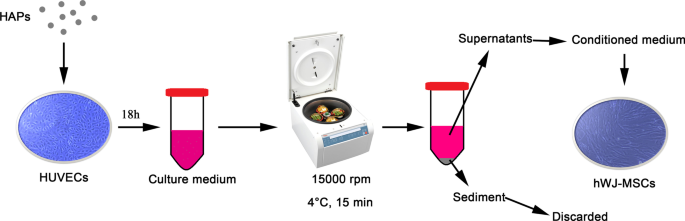
HUVECs 介导的 HAPs/hWJ-MSCs 间接共培养的图示。缩写:HAP 羟基磷灰石颗粒,hWJ-MSCs 人脐带沃顿氏果冻间充质干细胞,HUVECs 人脐静脉内皮细胞
图>确定细胞活力和数量
使用 MTS 检测试剂盒(MTS;Bestbio,Beyotime Biotechnology,Shanghai,China)评估细胞活力。 hWJ-MSC 粘附 24 小时,然后与 CM 一起培养 24 和 72 小时。使用酶标仪 (SpectraMax M2; Molecular Devices LLC, Sunnyvale, CA, USA) 在 490 nm 处评估甲臜的吸光度。在相同条件下,使用标准校准曲线将吸光度转换为细胞数。
定量实时聚合酶链反应 (RT-PCR)
TRIzol 试剂(Invitrogen,Carlsbad,CA,USA)用于从在 CM 中孵育指定时间的 hWJ-MSCs 细胞中分离总 RNA。使用 PrimeScript 第一链 cDNA 合成试剂盒(TaKaRa,东京,日本)在 T3 热循环仪(Mastercycler 5333;Eppendorf,Hamburg,Germany)中从 1.0 µg RNA 转录互补 DNA。使用 FastStart Universal SYBR Green Master (ROX) 试剂盒(Roche,Basel,Switzerland)在定量实时扩增系统(7900HT Fast;Applied Biosystems,Foster City,CA,USA)上分析指定基因的表达水平。将靶基因的相对 mRNA 表达归一化为 GAPDH,然后使用 \(2^{{ - \Delta \Delta C_{t} }}\) 方法确定。目的基因引物序列见表1。
图>茜素红 S (ARS) 染色和定量分析
hWJ-MSCs 在具有成骨培养基的 12 孔板中培养长达 14 天,然后使用 ARS 染色(Leagene,Leagene Biotechnology,Beijing,China)观察细胞外基质矿化。简而言之,将样品用无水乙醇固定 15 分钟,然后在室温下在 1% (w/v) ARS(pH,4.2)中染色 5 分钟。染色的细胞用双蒸水洗涤两次,然后拍照。为了对矿化过程进行定量分析,将 300 μL 10% (w/v) 氯化十六烷基吡啶鎓(BOMEI,BOMEI Biotechnology,合肥,中国)加入到每个孔中,并将板温育 30 分钟。将每个样品中总共 90 μL 转移到 96 孔板中,然后在 405 nm 处测量吸光度,一式三份。
碱性磷酸酶 (ALP) 染色和定量分析
hWJ-MSC 在 12 孔板中用成骨培养基培养长达 14 天后,使用 BCIP/NBT 碱性磷酸酶显色试剂盒 (Beyotime) 进行 ALP 染色。简而言之,hWJ-MSCs 固定在 4% 多聚甲醛中。然后,将样品在硝基蓝四唑鎓和 5-溴-4-氯-3-吲哚基磷酸酯的混合物中染色 4 小时并拍照。为了量化 ALP 合成,将细胞在冰冷的 RIPA 裂解缓冲液(Beyotime)中裂解 30 分钟。细胞裂解物在 4°C 下以 12,000 rpm 离心 10 分钟,上清液使用 ALP 检测试剂盒 (Beyotime) 进行 ALP 定量分析。吸光度在 405 nm 处重复测量三次,并使用标准曲线转换为 ALP 活性。
酶联免疫吸附测定 (ELISA)
HUVEC 以 2 × 10 5 接种 细胞/孔。从与 HAP 一起培养 18 小时的 HUVEC 中收集 CM(附加文件 1),并对上清液进行 ELISA 分析(图 1)。人源 HIF-1α ELISA 试剂盒(安徽乔伊生物技术公司,安徽,中国)按照制造商的说明使用。
免疫荧光
HUVECs 接种在 12 孔板的载玻片上 (7.6 × 10 4 细胞/孔)。暴露于 CM 18 小时后,将细胞固定在 4% 多聚甲醛(Biosharp,北京,中国)中,并在与 1% 抗 HIF-1α 抗体 [EPR16897 ](ab179483, Abcam, UK) 4℃过夜。随后,将细胞与 1% CoraLite594-缀合的山羊抗兔 IgG (H + L)(Proteintech,美国)在黑暗中孵育 1 小时。然后,将细胞核用 DAPI(Beyotime,上海,中国)染色,将其加入细胞中并反应 30 秒。使用激光共聚焦显微镜(Olympus,日本)检查样品。使用ImageJ v.1.4分析软件(Bethesda, MD, USA)定量荧光强度。
Western Blot 分析
hWJ-MSC 在 CM 中培养 24 小时(细胞外信号调节激酶(ERK)1/2,p-ERK1/2)或在成骨培养基中培养 7 天(runt 相关转录因子(RUNX)-2,1 型)胶原蛋白/胶原蛋白 1 (COL I))。然后,将细胞在 RIPA 裂解缓冲液中裂解 30 分钟。将细胞裂解物离心,并将上清液储存在 - 20 °C 进行分析。 12% SDS-PAGE 后,将蛋白质转移到聚偏二氟乙烯 (PVDF) 膜上。使用的一抗是抗 p-ERK1/2、抗 ERK1/2、抗 RUNX-2、抗 COL I 和 GAPDH(1:1,000,兔多克隆抗体;Cell Signaling Technology,波士顿,马萨诸塞州,美国)。去除未结合的抗体后,将膜与二抗孵育 1 小时。使用化学发光凝胶成像系统(LAS4000M;GE Healthcare Biosciences AB,Uppsala,Sweden)检测膜上的信号。使用ImageJ v.1.4分析软件(Bethesda)定量p-ERK与ERK和RUNX-2/COL I与GAPDH的比率。
细胞凋亡评估
hWJ-MSCs 以 10 5 的密度接种 6 孔板中每孔细胞数。贴壁细胞用指定浓度的 HIF-1α 处理指定时间。然后收集细胞并用 FITC-膜联蛋白 V 和 PI(Fcmacs,南京,中国)在黑暗中标记 15 分钟。所有样品均使用 FACScan 流式细胞仪(BD Bioscience, San Jose, CA, USA)进行测试。数据使用 FlowJo v10 (BD Biosciences) 进行分析。
两阶段细胞谱系模型
考虑到 HAPs 的巨大潜力,以及分析共培养系统的困难,需要一个可以提供定量分析的数学模型,需要可靠的预测来理解 HIF-1α 在 hWJ- 成骨分化中的作用间充质干细胞。
hWJ-MSC 与 0、300、500、1000、1500、2000、3000 和 4000 pg/mL HIF-1α 以及成骨诱导液一起培养。在对这些浓度的数据进行拟合后,我们使用对照、m-HAP、np80 和 np20 组(分别为 240、300、325 和 375 pg/mL)中的 HIF-1α(由 HUVEC 产生)的浓度来确定使用 MATLAB (MathWorks, Natick, MA, USA) 测试拟合方程。为了简化模型,我们认为它们在不同浓度的 HIF-1α 下的相似生长模式是相同的。采用平均分化程度拟合分化程度-时间方程。在规定时间检测不同组hWJ-MSCs的增殖率、凋亡率和成骨分化程度。
根据实验数据建立了一个简化的两阶段细胞谱系模型,类似于多阶段细胞谱系模型 [18, 19]。 C 0 和 C 1分别代表hWJ-MSCs和终末细胞的细胞数。 C 0 和 C 1 受以下约束:
$$\left\{ \begin{gathered} \frac{{{\text{d}}C_{0} }}{{{\text{d}}t}} =\left[ {\frac{{K - C_{0} - C_{1} }}{K}p - (p - 1)} \right]\upsilon_{0} C_{0} \hfill \\ \frac{{{\text{d}} C_{1} }}{{{\text{d}}t}} =\left( {2 - \frac{{K - C_{0} - C_{1} }}{K}p - p} \ right)\upsilon_{0} C_{0} - AC_{1} \hfill \\ \end{gathered} \right.$$在这里,p ,受 HIF-1α 和时间的影响,代表 hWJ-MSC 的复制概率。相应地,d =1 − p 是细胞数的估计和实验数据拟合得到的分化率。参数 v0 量化细胞在每个谱系阶段分裂的速度(特别是 v =ln2/c ,其中 c 是细胞周期的持续时间)。终末细胞凋亡率用A表示 .为简单起见,我们忽略了细胞凋亡率会随时间略有变化,因此 A =4.5% 是一个常数。 K 表示环境容量,因为细胞不能无限增殖 [20]。 HIF-1α 增加 hWJ-MSCs 的分化率,导致分化率建模为:
$$\begin{aligned} d &=\frac{{d_{0} }}{{1 + (r*H)^{m} }} \\ p &=1 - d \\ \end{aligned} $$在此,H 代表 HIF-1α 的浓度; d 0 表示在 0 pg/mL HIF-1α 时的分化率; r代表调控强度(此处代表HIF-1α对MSCs的调控强度); m对应Hill系数[21],说明MSC分化率与HIF-1α浓度的关系。
统计分析
满足正态性和同方差性要求的所有数据表示为来自三个或更多独立实验的平均值 ± 标准偏差 (SD)。 SPSS 24.0 软件(SPSS Inc., Chicago, USA)用于通过单向方差分析或双向方差分析进行统计分析。 A P 值 <0.05 被认为具有统计学意义。使用 GraphPad Prism 5(GraphPad Software, La Jolla, CA)进行统计分析。
结果
HAP 的特征
如图 2 所示,制备了具有特定尺寸和形状的 HAP。接近球形的np20的直径平均为20nm,np80为棒状,平均长度为80nm,宽度为20nm。 m-HAP 的形状也接近球形,直径约为 12 微米。所有颗粒在 L-DMEM 中都带有负表面电荷。已经表明,zeta 电位的负值对骨细胞的附着和增殖以及直接骨结合和新骨形成具有显着的有利影响 [22, 23]。在 L-DMEM 中观察到的颗粒有在水性体系中聚集的趋势。还测试了它们的流体动力学尺寸,这可能也是影响其生物学行为的重要因素。
<图片>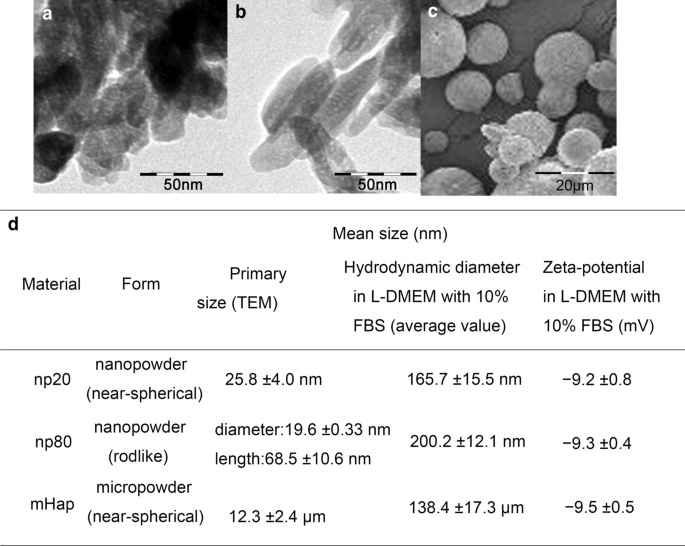
HAP 的表征。 a 的 TEM 显微照片 np20 和 b np80 和 c 的 SEM 显微照片 M-HAP。 d HAP 的表征 (n =6)。缩写:TEM 透射电子显微镜,SEM 扫描电子显微镜,HA 羟基磷灰石,m-HAP 微型HAP颗粒
图>HAPs 对 hWJ-MSCs 的间接毒性
为了评估 HAPs 对 hWJ-MSCs 的间接毒性,通过 MTS 测定测量细胞活力。含有 50 µg/mL HANP 的 CM 可以在 24 小时和 72 小时后显着刺激 hWJ-MSC 的活力,尤其是在 24 小时。然而,与 72 小时后的对照相比,含有 100 µg/mL HANP 的 CM 将细胞活力降低了 15-20%。此外,含有 25 µg/mL np20 而不是 np80 的 CM 在 24 小时后刺激细胞活力。这些现象证实,50 µg/mL HANP 是在所有后续实验中使用的亚细胞毒性浓度(图 3)。
<图片>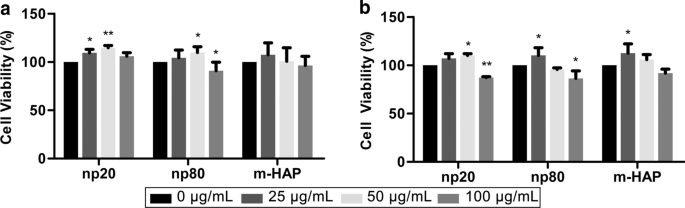
HAPs 对 hWJ-MSCs 的间接毒性。用HAPs间接培养hWJ-MSCs的活力测定a 24 和 b 72 小时。 *P <0.05; **P 相对于对照<0.01。对照组由在没有 HAP 处理的 CM 中培养的细胞组成,细胞活力被标准化为对照的百分比。缩写:HAP 羟基磷灰石颗粒,m-HAP 微型HAP颗粒,hWJ-MSCs 人脐带沃顿氏果冻间充质干细胞
图>HAPs 对 hWJ-MSCs 的间接骨诱导作用
为了确定 HANP 对 hWJ-MSC 的间接骨诱导作用,通过定量 RT-PCR 分析评估了成骨基因的表达。 HANP 组中 runt 相关转录因子 2(RUNX-2)的转录水平,尤其是 np20,从第 7 天到第 21 天显着增加(图 4a)。 np20 组中 I 型胶原蛋白 (Col I) 的基因表达从第 7 天到第 21 天增强,而 np80 组从第 7 天到第 14 天持续增加(图 4b)。在第 14 天,HANP 组的骨钙素 (OCN) mRNA 明显上调,表明成骨速度加快(图 4c)。 HANP组碱性磷酸酶(ALP)的mRNA水平明显增加(图4d),表明hWJ-MSCs的成骨分化。然而,与对照组相比,m-HAP 组在这三种成骨基因的水平方面表现出有限的变化(图 4)。此外,与对照组相比,HANPs 组中多能性标志物 NANOG、OCT3/4 和 SOX2 的表达降低(图 4e-g),这表明 HANPs 组中的 hWJ-MSCs 已经分化,尤其是在np20 组。 Western印迹分析也得到了类似的结果(图5c、d),表明np 20组可以间接增强hWJ-MSCs中RUNX-2和COL I的表达。
<图片>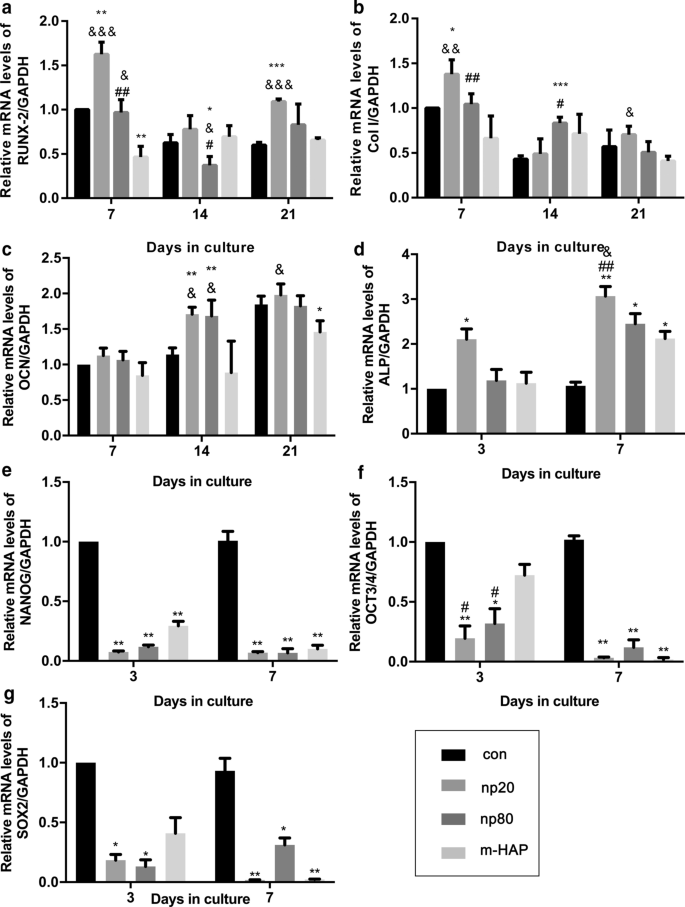
HAPs对成骨分化相关基因表达的间接影响。 一 RUNX-2,b Col I,c OCN,d 碱性磷酸酶,e NANOG,f OCT3/4 和 g 在指定时间用 CM 培养的 hWJ-MSC 中的 SOX2 基因水平。 *P <0.05; **P <0.01; ***P <0.001 与对照组相比; & P <0.05; && P <0.01; &&& P <0.001 与 m-HAP 组相比; # P <0.05; ## P <0.01 与 np20 组相比。在没有HAPs处理的成骨培养基中培养的细胞用作对照组。缩写:HAP 羟基磷灰石颗粒,m-HAP 微型HAP颗粒,hWJ-MSCs 人脐带沃顿氏果冻间充质干细胞,RUNX-2 runt 相关转录因子 2,Col I I 型胶原蛋白,OCN 骨钙素,ALP 碱性磷酸酶,SOX 2 SRY相关HMG-box 2
图> <图片>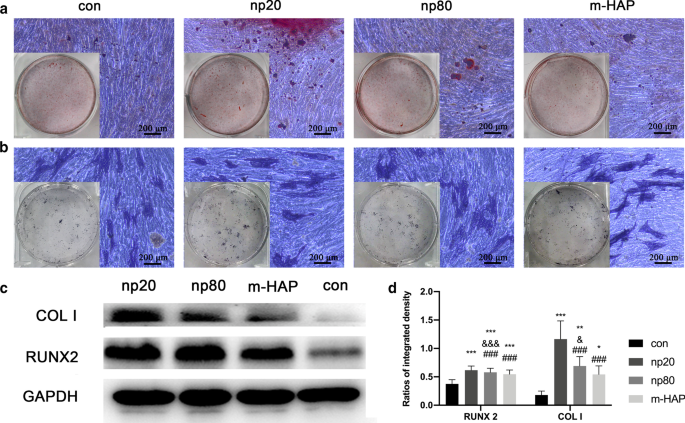
HAPs 对细胞外钙沉积和 ALP 活性的间接影响。 hWJ-MSCs 在成骨培养基中培养 14 天。 一 然后通过 ARS 染色观察细胞外钙沉积; b hWJ-MSC 的 ALP 活性通过 ALP 染色评估,比例尺:200 μm。 c Western Blot分析表明hWJ-MSCs在第7天成骨培养基中RUNX-2和COL I的表达。d RUNX-2 和 COL I 的光密度测量结果 (c )。在没有HAPs处理的成骨培养基中培养的细胞用作对照组。 *P <0.05; **P <0.01; ***P <0.001 与对照组相比; & P <0.05; &&& P <0.001 与 m-HAP 组相比; ### P <0.001 与 np20 组相比。缩写:HAP 羟基磷灰石颗粒,m-HAP 微型HAP颗粒,hWJ-MSCs 人脐带沃顿氏果冻间充质干细胞,ALP 碱性磷酸酶
图>为了直观地观察 HANP 对 hWJ-MSC 的间接骨诱导作用,将细胞与指定的成骨培养基孵育 14 天,然后进行 ARS 和 ALP 染色。如图 5a、b 所示,与 m-HAP 和对照组相比,在 HANP 组中观察到矿化结节数量增加和 hWJ-MSC 的 ALP 活性更高。此外,与对照组相似,m-HAP对hWJ-MSCs的成骨分化作用有限。
HAP 激活与 HUVEC 间接共培养的 hWJ-MSC 中的 ERK1/2 信号
为了研究 HAPs 对 HUVECs 旁分泌功能的影响,使用免疫荧光和 ELISA 测定来鉴定可能促进 hWJ-MSCs 成骨分化的蛋白质。如图6a-c所示,HANPs(尤其是np20)显着促进了HIF-1α的细胞内外产生,而m-HAP对其产生的影响有限。
<图片>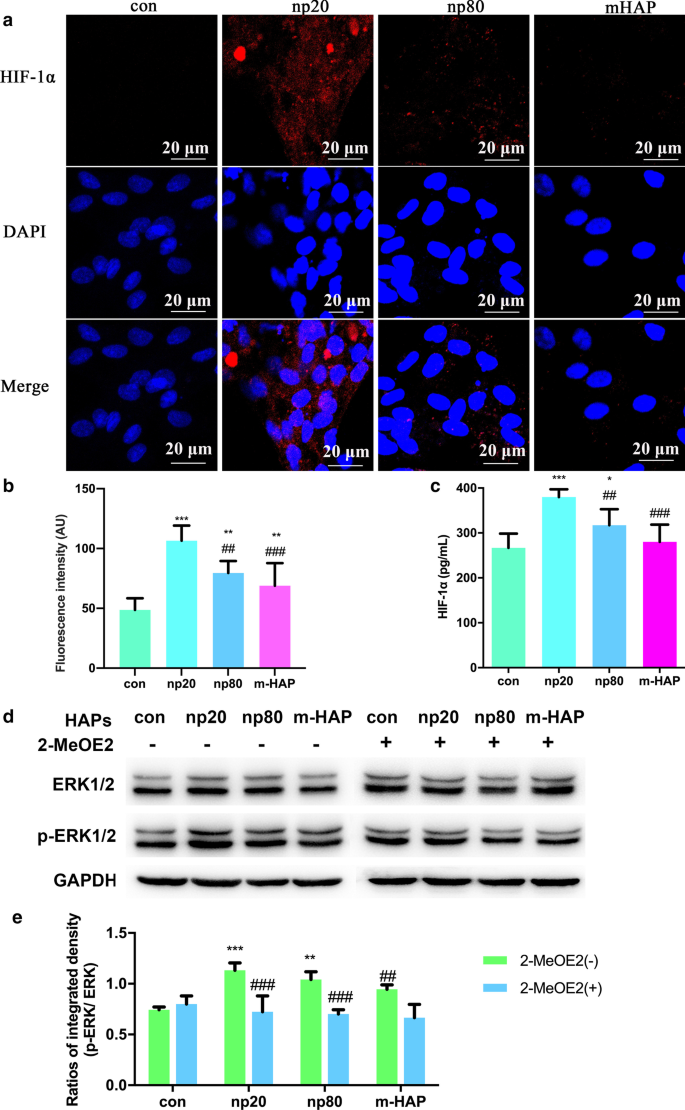
HAPs 在与 HUVECs 间接共培养的 hWJ-MSCs 中激活 ERK1/2 信号。 一 在用/不用 HAP 处理 18 小时的 HUVEC 中进行的 HIF-1α 免疫荧光。 b 来自(a部分的HIF-1α的荧光强度 )。 c 通过ELISA测量用/不用HAP处理18小时的HUVEC的培养基中HIF-1α的细胞外浓度。比例尺:20 μm。 *P <0.05; **P <0.01; ***P <0.001 对比对照; ## P <0.01; ### P <0.001 与 np20 组相比。未经HAPs处理的细胞用作对照组。 hWJ-MSC 用 CM 处理 24 小时。 d 蛋白质印迹分析表明 ERK1/2 通路中关键激酶的激活。 e p-ERK1/2 部分 (b )。 **P <0.01; ***P <0.001 对比对照; ## P <0.01, ### P <0.001 与 2-MeOE2( -) 组相比。在没有HAP处理的CM中培养的细胞用作对照组。缩写:HAP 羟基磷灰石颗粒,m-HAP 微型HAP颗粒,hWJ-MSCs 人脐带沃顿氏果冻间充质干细胞,HUVECs 人脐静脉内皮细胞,ERK 细胞外信号调节激酶,HIF-1α 缺氧诱导因子1α
图>为了更准确地了解 HIF-1α 激活的 hWJ-MSC 的分化信号通路,我们通过蛋白质印迹分析检查了 ERK1/2 通路的关键调节因子。如图 6d、e 所示,虽然总 ERK1/2 的蛋白质水平保持不变,但在用 HANP 培养的 hWJ-MSC 中 p-ERK1/2 水平明显增加,这在 np20 组中尤其如此。然而,m-HAP 对 hWJ-MSCs 中的 p-ERK1/2 水平几乎没有影响,类似于它对 HUVECs 中 HIF-1α 产生的影响。重要的是,HIF-1α 激活的 hWJ-MSCs 中 p-ERK1/2 水平的增加可以被 2-MeOE2(一种特定的 HIF-1α 抑制剂)阻断,表明 HIF-1α 在 ERK1/2 信号通路上游起作用在 hWJ-MSCs 中。
HIF-1α 通过 ERK1/2 通路促进 hWJ-MSC 的成骨分化
为了确定观察到的 hWJ-MSC 成骨分化刺激是否需要 HIF-1α,将特定的 HIF-1α 抑制剂 (2-MeOE2) 应用于这些细胞培养物。如图 7 所示,在成骨培养基中培养的 2-MeOE2 (+) 处理组 hWJ-MSCs 的矿化基质沉积和 ALP 活性减弱,表明 HIF-1α 对于 hWJ- 的成骨分化必不可少。 MSC。基于这些结果,我们进一步探讨了 ERK1/2 通路在 HIF-1α 激活的 hWJ-MSC 成骨分化中的作用。使用特异性MEK抑制剂PD98059后,成骨培养基培养的hWJ-MSCs的矿化基质沉积和ALP活性受到抑制。
<图片>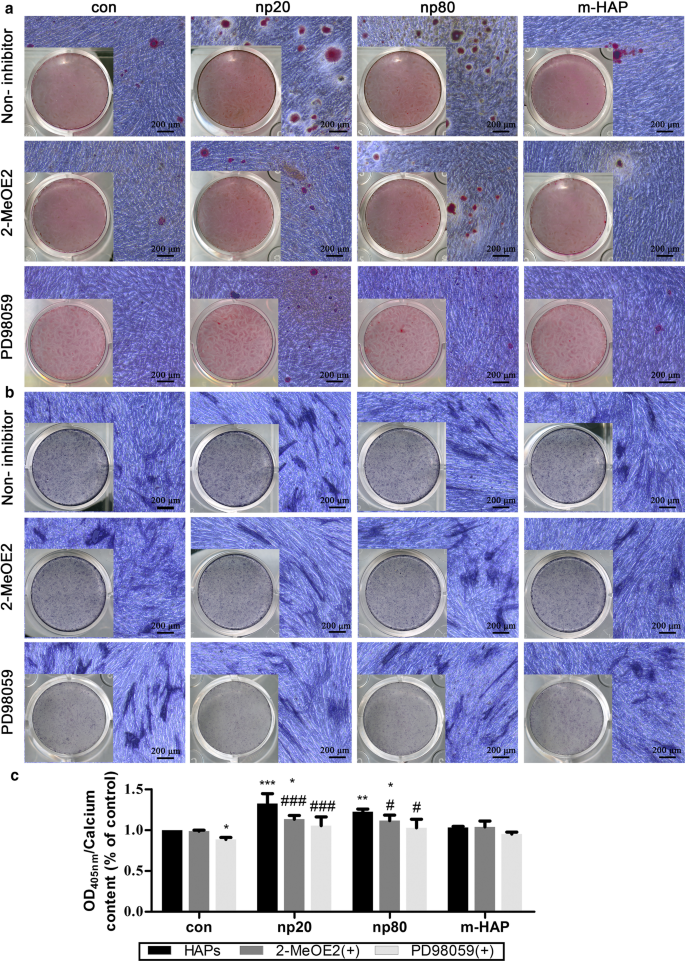
HIF-1α 通过 ERK1/2 通路促进 hWJ-MSCs 的成骨分化。 hWJ-MSC 在含有或不含 PD98059 的成骨培养基中培养 14 天。 一 通过 ARS 染色观察细胞外钙沉积。 b 通过 ALP 染色评估 hWJ-MSC 的 ALP 活性。比例尺:200 微米。 c 细胞外钙基质的定量分析。 *P <0.05; **P <0.01; ***P <0.001 对比对照; # P <0.05; ### P < 0.001 versus the np20 group. Cells incubated in osteogenic medium without HAPs and PD98059 treatment were used as the control group. Abbreviations:HAPs hydroxyapatite particles, m-HAP micro-sized HAP particles, hWJ-MSCs human umbilical cord Wharton’s jelly-derived mesenchymal stem cells, HUVECs human umbilical vein endothelial cells, ERK extracellular signal-regulated kinase, HIF-1α hypoxia-inducible factor 1α, ALP alkaline phosphatase
图>Two-Stage Cell-Lineage Models
To quantitatively reveal the intrinsic connection between the concentration of HIF and osteogenic differentiation of hWJ-MSCs, 0–4000 pg/mL of HIF-1α was used to treat eight groups of hWJ-MSCs. A two-stage cell-lineage mathematical model was used to analyze the proliferation, apoptosis, and osteogenic differentiation rates of these hWJ-MSCs treated with different concentrations of HIF-1α. As shown in Fig. 8a, fitting data were employed to obtain the simulated formula (\(\frac{d}{{d_{0} }} =\frac{1}{{0.14H^{2} - 0.43H + 1}}\)) and curve (blue curve), showing that the differentiation rate first increased and then decreased with the increase in HIF concentration. More specifically, the differentiation rate reached a peak at 1500 pg/mL HIF-1α.
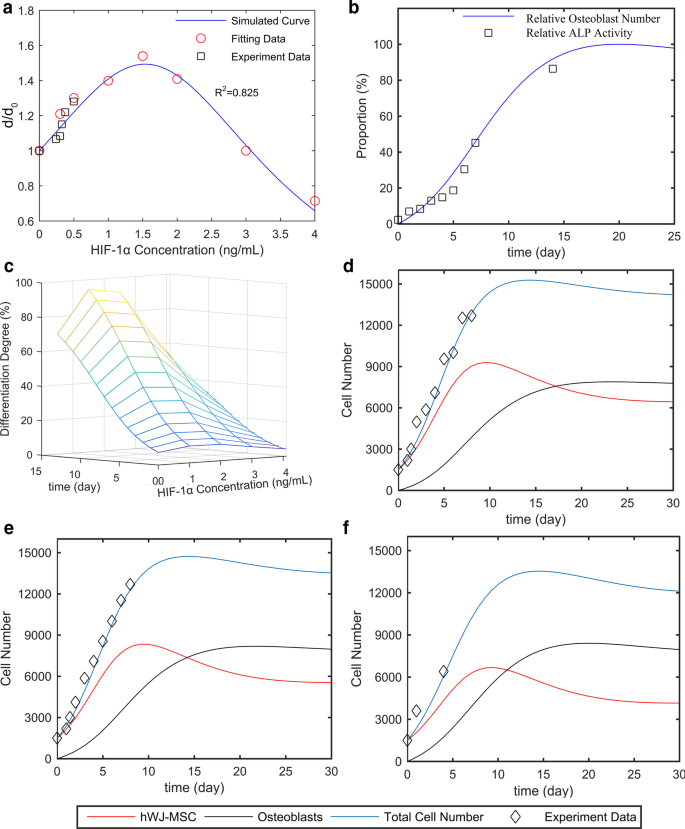
Two-stage cell-lineage models. hWJ-MSCs were incubated in a defined concentration of HIF-1α for the indicated times. 一 Relative differentiation rates of hWJ-MSCs at different concentrations of HIF-1α, b relative ALP activity (differentiation degrees of hWJ-MSCs) and relative osteoblast cells on different culture days. c Three-dimensional surface of differentiation degree evolving with time and HIF-1α. Cell number with an initial seeding density of 1500 cells per well (in a 96-well plate), as well as d 0, e 375, and f 1500 pg/mL HIF-1α. Abbreviations:hWJ-MSCs human umbilical cord Wharton’s jelly-derived mesenchymal stem cells, HIF-1α hypoxia-inducible factor 1α, ALP alkaline phosphatase
图>According to our study, the concentrations of HIF-1α produced by HUVECs in the control, m-HAP, np80, and np20 groups were 240, 300, 325, and 375 pg/mL, respectively. The black square represents the differentiation rate promoted by 240, 300, 325, and 375 pg/mL HIF-1α, and this matches well with the simulated curve. In addition, the differentiation degrees of the hWJ-MSCs (relative ALP activity) treated with different concentrations of HIF-1α increased similarly increased with time. Therefore, in order to simplify the model, we consider their growth patterns to be similar or even identical. We found that the increase in ALP activity from Day 0 to Day 7 was proportional to the number of osteoblasts, and osteoblast cells reached their peak at the platform period. Therefore, after fitting the ALP activity with the relative osteoblasts cell number curve (osteoblasts cell number / maximum osteoblasts cell number), the maximum of ALP activity was predicted and relative ALP activity (ALP activity/ maximum of ALP activity), representing the differentiation degree, was acquired (Fig. 8b). Combining these two studies, the three-dimensional surface of the differentiation degree evolving with time and HIF-1α was obtained (Fig. 8c).
In order to estimate the optimal culture time, it was necessary to simulate cell numbers. After elucidating the differentiation rate under different concentrations of HIF-1α, we simulated the size of each population. The simulation utilized an initial seeding density of 1500 cells per well (in a 96-well plate) using different concentrations of HIF-1α. As shown in Fig. 8d–f, the experimental data (black square) match well with the simulated total cell numbers (blue curve), which is a sum of the number of hWJ-MSCs and osteoblasts, and this supports the ability of this model to predict the number of hWJ-MSCs and osteoblasts at any time point. Moreover, the osteoblast cell number approached the platform period at 21, 18, and 15 days with 0, 375, and 1500 pg/mL HIF-1α, respectively. This model provides the optimum culture time for guiding tissue engineering, and it also provides direct evidence that HIF-1α accelerates the osteogenic differentiation of hWJ-MSCs.
讨论
With recent advances in nanobiomaterials, nano-based artificial bone substitutes have been an area of intense investigation. The accumulating evidence suggests that there are complex interactions between cells and nanobiomaterials due to their capacity to penetrate cell membranes and increase internal retention times [24, 25]. A previous study revealed that collagen/alginate nanofilms can adsorb onto the MSC membrane to activate intracellular signaling cascades and promote osteogenic differentiation [26]. Elegant experiments by Wu and his colleagues clearly demonstrated that TiO2 nanotubes can improve vascularization and osteogenic differentiation by facilitating paracrine effects and cell junctions via EC-MSC interactions [27]. For the purpose of developing excellent candidates for bone tissue engineering, it is necessary to clarify the direct crosstalk between nano-based bone substitutes and cells implicated in bone repair as well as their indirect interactions. However, our current understanding of this is still limited. In the present study, we utilized an indirect co-culture model to further elucidate the biological effects of HANPs on MSCs in regard to the indirect interactions mediated by ECs.
Cytotoxicity is a primary issue for assessing the biocompatibility of any nanobiomaterial. Although our previous study found that HANPs did not directly influence the viability or apoptosis of hWJ-MSCs, they may still exert different impacts via the mediation of other cells [28]. Thus, it was necessary to evaluate the cytotoxic effects of HANPs on hWJ-MSCs mediated by HUVECs. Interestingly, after incubation in CM for 24 h and 72 h, hWJ-MSC viability was maintained and even elevated in the 0–50 µg/mL HANP groups, especially in the np20 group, indicating the existence of effector molecules in the CM. When the concentration of HANPs reached 100 µg/mL, they became cytotoxic to the hWJ-MSCs. However, 0–100 µg/mL m-HAP had no influence on hWJ-MSC viability (Fig. 3).江等人。 have shown that engineered nanoparticles of a particular size can have distinct endocytic routes and kinetics associated with altered downstream signaling involved in regulating target cell functions [29]. In our previous study, we showed that np20 and np80 were endocytosed by HUVECs, and this was followed by morphologic changes and the appearance of large vacuoles, indicating the activated state of the HUVECs. Additionally, np20, with their faster uptake speed and increased accumulation, might result in a stronger activation of HUVECs, possibly resulting in increased hWJ-MSC viability via paracrine signaling. Conversely, few m-HAPs can be endocytosed by HUVECs, and this might account for their limited influence on the metabolism of hWJ-MSCs [9].
To further explore the potential osteoinductive effect of activated HUVECs, a subcytotoxic dose of 50 µg/mL HAPs was used in subsequent studies. The CM collected from the activated HUVECs promoted extracellular calcium deposition, ALP activity, and osteogenic proteins expression in hWJ-MSCs, as well as the mRNA expression of osteogenic genes (Figs. 4, 5). Runx2, an essential transcription factor involved in specifying the osteoblast lineage [30], showed a substantial enhancement in the np20 group, indicating a strong osteoinductive effect on hWJ-MSCs (Fig. 4a and Fig. 5c, d). The np20 group demonstrated a 1.5-fold improvement in COLI expression at Day 7 (Figs. 4b, 5c, d) and a double increase at Day 14, which implied the presence of additional differentiated osteoblasts in the HANP-treated groups (Fig. 4b) [30]. OCN is a mature stage bone marker [31], and this gene showed a significant increase in the HANP groups at Day 14 (Fig. 4c), indicating that np20 and np80 can accelerate bone maturation compared to m-HAP. ALP is an early marker of osteoblast differentiation, and it obviously increased with culture time in each group, especially the np20 group, revealing that additional transformation occurred from MSCs to osteoblasts (Fig. 4d). Pluripotency markers, NANOG, OCT3/4, and SOX2 imply the capacity for differentiation [32]. As shown in Fig. 4e–g, the decreased expression in the genes of the HANP groups implied that most of the hWJ-MSCs in HAP groups had transformed into osteoblasts.
Our data demonstrated that the endocytosis of HANPs by HUVECs was associated with an improved osteogenic differentiation of hWJ-MSCs. However, the cause of this outcome is currently unclear. In terms of the paracrine function of HUVECs, we focused on soluble differentiation-inducing proteins in the supernatant of activated HUVECs. HIF-1α signaling is essential in coupling ossification and angiogenesis during bone regeneration [33, 34]. Heikal et al. reported that injured ECs secrete more HIF-1α even under normoxia conditions [35]. It has also been shown that exposure to HANPs inhibits the angiogenic ability of HUVECs [9]. Thus, we measured the concentrations of HIF-1α in the CM, and the results showed that the HIF-1α content increased in the HANP treatment groups compared to the m-HAP and control groups (Fig. 6a). To identify the role of HIF-1α in the osteogenic differentiation of hWJ-MSCs, we used 2-MeOE2, which is a specific HIF-1α inhibitor, was used. The decreased concentration of HIF-1α paralleled the impaired mineralized matrix deposition and ALP activity in these hWJ-MSCs, indicating that HANPs can promote the HIF-1α production of HUVECs to facilitate the osteogenesis of hWJ-MSCs (Fig. 7).
To properly apply HANPs for use in bone tissue engineering, it is necessary to gain further insights into the mechanisms by which HANPs promote the osteogenic differentiation of hWJ-MSCs mediated by HUVECs. The ERK1/2 pathway is downstream of HIF-1α [36] and is fundamental to the differentiation of MSCs [37]. In this work, the concentrations of HIF-1α in the CM coincide well with the p-ERK1/2 levels in the hWJ-MSCs (Fig. 6b, c). When 2-MeOE2 was applied, the p-ERK1/2 expression in the hWJ-MSCs failed to be activated, indicating that HIF-1α functioned upstream of ERK1/2 signaling. To directly address the role of ERK1/2 signaling in the osteogenic differentiation of hWJ-MSCs, PD98059, a specific MEK inhibitor, was used. The suppression of ERK1/2 signaling resulted in the lowest osteogenic differentiation of hWJ-MSCs. One possible reason for this occurrence is that the ERK1/2 pathway plays a key role in both HIF-1α signaling and in the apoptosis and proliferation signaling pathways, which could be responsible for the observed changes in osteogenic differentiation in these cells [38, 39]. Additionally, this could also be related to the presence of vascular endothelial growth factor (VEGF). VEGF is one of the downstream effectors of HIF-1α signaling [33], and it can also promote the osteogenic differentiation of MSCs via activation of the ERK1/2 pathway [37]. Our previous study found that np20 induced the production of VEGF in HUVECs [9]; therefore, it is possible that the suppression of the ERK1/2 pathway may result in inhibition of VEGF, which would lead to the decreased osteogenic differentiation of hWJ-MSCs. According to the available experimental results, we can summarize as follows. HANPs are able to more optimally process better direct [5] and indirect osteoinductive effects than m-HAPs. Compared to autogenous bone grafts and bone allografts, there is an extensive source of HANP and without secondary damage and potential immunogenicity. However, compared to m-HAPs, HANPs can suppress the angiogenic ability of HUVECs [9] and exhibit slight cytotoxicity in both a time- and dose-dependent fashion.
Recently, growing evidence has demonstrated the importance of HIF-1α in the bone regeneration. However, few studies have been able to quantitatively predict the MSC differentiation rate under specific initial conditions, such as the HIF-1α concentration. Taking cell proliferation, apoptosis, and osteogenic differentiation into account, we present a mathematical model that combines a two-stage cell lineage with HIF-1α that is highly correlated with our experimental data. By fitting the differentiation rate of hWJ-MSCs in 0–4000 pg/mL HIF-1α, we acquired the equations for describing the differentiation rate, HIF-1α concentration, and time. As shown in Fig. 8d, this model can depict the cell number map under different HIF-1α concentrations, so that it is possible to explore the intrinsic dynamics of the two-stage system [40]. Additionally, this model mathematically validates the effect of HIF-1α on the osteogenic differentiation of hWJ-MSCs. Moreover, based on a multi-stage cell-lineage model and logistic model, our model is sufficiently stable to enable long-term predictions without falling into the trap of population unlimited explosion [41].
By using the existing experimental data, both the cell number and differentiation rate can be predicted with a defined initial cell seeding density and HIF-1α concentration. As such, the optimum incubation time is also obtained. Consequently, we can predict the optimum concentration of HIF-1α and determine the most optimal time for osteogenesis, which is important for efficient tissue engineering. A two-stage cell-lineage model is applicable for predicting the proliferation and differentiation of stem cells, which have two cell lineages. On this basis, the model founded on the initial conditions and existing experimental data can be established to identify the optimum culture conditions in vitro, which will assist in optimizing bone repair in vivo.
结论
In this study, we explored the specific biological effects of HANPs on hWJ-MSCs mediated by HUVECs. Compared to m-HAPs, both np20 and np80 showed slight cytotoxicity in both a time- and dose-dependent fashion. Importantly, the size of the HANPs appeared to have no significant impact on this cytotoxicity. Our data also showed that HANPs, especially np20, were capable of facilitating HUVECs to secrete increased levels of HIF-1α, which directly correlated with the enhanced osteogenic differentiation of hWJ-MSCs via the activation of the ERK1/2 pathway (Fig. 9). More remarkably, the results from the two-stage cell-lineage model suggested that HIF-1α exerted a dose-dependent stimulatory effect on the osteogenic differentiation rate of hWJ-MSCs. Additionally, the optimum concentration of HIF-1α and incubation time were estimated based on the initial conditions using an in vitro model, which could be invaluable in the future for tissue engineering applications. Collectively, these observations provide evidence that HANPs may improve bone regeneration by modulating cell–cell interactions.
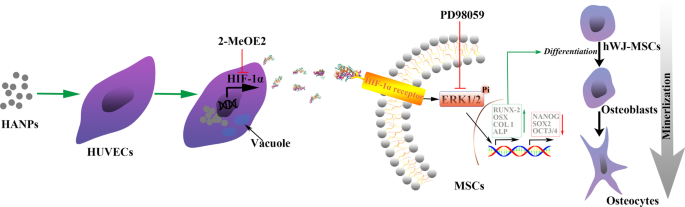
A schematic illustration of the possible mechanisms
图>数据和材料的可用性
本研究中使用和分析的数据集可向相应作者索取合理要求。
缩写
- HA:
-
Hydroxyapatite
- HANPs:
-
HA nanoparticles
- hWJ-MSCs:
-
Human umbilical cord Wharton’s jelly-derived mesenchymal stem cells
- HUVECs:
-
人脐静脉内皮细胞
- m-HAP:
-
Micro-sized HAP particles
- PBS:
-
磷酸盐缓冲盐水
- ECM:
-
EC medium
- 2-MeOE2:
-
2-Methoxyestradiol
- ELISA:
-
酶联免疫吸附试验
- RUNX-2:
-
Runt-related transcription factor 2
- Col I:
-
Type I collagen
- OCN:
-
Osteocalcin
- ALP:
-
碱性磷酸酶
- SOX 2:
-
SRY-related HMG-box 2
- ERK:
-
Extracellular signal-related kinases
- VEGF:
-
Vascular endothelial growth factor
纳米材料
- 专用电池
- 太阳能电池
- 聚合物纳米结构上 U2OS 细胞中肌动蛋白和焦点粘附组织的分析
- 纳米技术灭活癌症干细胞
- Au 纳米颗粒对 HT29 和 SPEV 细胞系影响的体外研究
- 磁性纳米粒子组装中的相互作用
- 使用多功能 GaN/Fe 纳米颗粒靶向内皮细胞
- Ag 纳米颗粒/BiV1-xMoxO4 与增强的光催化活性的协同效应
- 用 6-巯基嘌呤和神经元穿透肽修饰的金纳米颗粒促进 SH-SY5Y 细胞生长
- 用银纳米粒子作为抗菌剂装饰的基于氧化石墨烯的纳米复合材料
- C60 富勒烯对二苯基-N-(三氯乙酰基)-氨基磷酸酯在硅胶中与 DNA 相互作用的影响及其对体外人白血病细胞系的细胞毒活性
- 基于生物羟基磷灰石的含锶微晶玻璃复合材料的开发和表征