基于金属氧化物/石墨烯/碳纳米管的纳米生物传感器的最新进展概述
摘要
纳米生物传感器是一种方便、实用且灵敏的分析仪,可检测化学和生物制剂,并将结果转换为生物活性分子和物理化学检测器固定在信号传感器表面的识别元件之间的有意义的数据。由于其快速、准确和可靠的操作特性,纳米生物传感器广泛应用于临床和非临床应用、床边检测、医用纺织工业、环境监测、食品安全等。它们在这些关键应用中发挥着重要作用。因此,生物传感界面的设计对于确定纳米生物传感器的性能至关重要。纳米材料独特的化学和物理特性为生物传感器中新型和改进的传感设备铺平了道路。对具有改进的传感和选择性能力、响应时间短、检测下限和成本低的设备的需求不断增长,促使对纳米生物材料用作生物传感器支架的新研究。在所有其他纳米材料中,基于金属氧化物纳米结构、石墨烯及其衍生物、碳纳米管的纳米生物传感器的开发研究以及这些纳米材料作为混合结构的广泛使用最近引起了人们的关注。通过组合这些纳米结构创建的纳米混合结构将以其高电催化活性直接满足未来生物传感器的需求。这篇综述讨论了这些纳米材料及其衍生物的最新进展,以及它们作为生物传感器支架的用途。我们通过比较研究、表格和图表对这些流行的纳米材料进行了评估。
介绍
生物传感器是一种诊断设备,可将来自生物分析物的信号转换为可测量和可区分的电信号,用于对可能夹杂其他物理化学物质的分析物进行定性和/或定量检测 [1]。第一个已知的生物传感器是由克拉克等人开发的。 [2] 用于检测氧气,克拉克和里昂 [3] 开发的第一个电流型酶电极是一种基于酶的葡萄糖生物传感器。多年来,已经开发了基于酶的、基于组织的、基于脱氧核糖核酸 (DNA) 的以及热、光学、电化学生物传感器类型。在临床诊断、生物医学领域、食品生产和分析等一些应用中,生物传感器比传统方法提供更稳定和精确的结果 [2, 4]。而且,具有特异性、选择性、成本节约、操作简单、分析实时、可连续使用等特点,各种类型的生物传感器在本世纪下半叶得到迅速发展,并广泛应用于相关医疗、环境和法医领域 [5]。它们在这些关键应用领域的大量使用已经从生物传感器中出现了一些预期的特性,如高灵敏度、稳定性、高选择性、长使用寿命、可重复性、简单和廉价、宽测量范围和快速响应时间 [6]。>
根据国际纯粹与应用化学联盟 (IUPAC),生物传感器包含三个主要组件:生物识别元件、换能器组件和经常与换能器结合的电子系统。作为集成的受体-传感器装置,生物传感器能够使用生物识别元件 [7](图 1)提供选择性定量或半定量分析信息。在此框架内,核酸、酶、抗体、受体、微生物、细胞、组织甚至仿生结构都可以作为生物检测的生物受体。
<图片>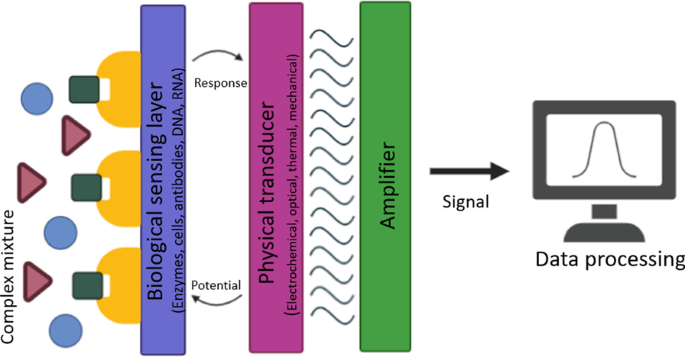
生物传感器示意图
图>生物传感器的设计对于在任何情况下或分析物可能出现的任何位置下进行快速方便的测试非常重要。在该设计中,换能器组件材料对检测质量也有显着影响。物理换能器随可量化信号源而显着变化,并且主要利用光学和电化学系统 [5]。用作物理换能器的材料的物理化学、电子/光学/电化学特性直接影响生物传感器的性能。此外,生物传感器的效率和有效性取决于用于酶固定的基质、介质和稳定剂。因此,生产物理换能器组件的材料的特性在获得诸如生物传感器的高信号稳定性和可重复性以及它们的选择性等特征方面起着关键作用。在生物传感器的上述三个组件中,本综述主要集中在使用纳米材料对换能器组件进行表面功能化的最新进展。
换能器主要可分为四类:电化学、生物发光、压电、量热和光学。传感器的表面可以通过使用多种不同的功能材料进行改性,以提高传感器的性能。控制这些材料的结构、形态和性质也有同样的帮助。在这些材料中,纳米材料,即纳米材料,以其独特的尺寸依赖性特性,如大表面积、改进的电导电性和高化学反应性。考虑到这些非凡的特性,纳米材料已成为满足构建高灵敏度生物传感器所需要求的首选材料之一[6]。
要被视为纳米材料,至少在一个维度上,纳米材料的尺寸应该在 1 到 100 纳米之间 [8]。由于它们非常微小的尺寸,在纳米材料中,大多数原子靠近表面或存在于表面上。这些纳米粒子 (NPs) 获得了显着的特征,如增强的物理化学性质、更高的表面积、更短的电子距离,与它们的大体积对应物相比具有显着差异。因此,这些纳米级材料的光学、热学、电学和磁学性能将保持提高,以非常有效地用作生物传感器组件。此外,具有更高表面积的纳米材料为在电极表面上固定足够数量的生物受体提供了合适的空间。因此,研究人员最近对用于生物传感器应用的纳米材料的生产、表征和使用表现出极大的兴趣 [9, 10]。
在所有纳米材料中,MON、石墨烯及其衍生物和碳纳米管因其独特的特性而脱颖而出[11, 12]。 MONs 由于其令人印象深刻的形态多样性、无毒和生物相容性而表现出显着的催化性能。还应注意的是,MONs为生物分子的固定提供了合适的结构。
它们的晶格允许由于量子限制效应而改变电池参数和电化学特性,并且通过改变其影响电导率和化学反应性的表面特性来控制带隙使它们非常有可能用作生物传感元件并将 MON 与其本体区分开来同行 [12, 13]。此外,为了通过形成复合结构进一步改善这些性能,最近将 MON 与碳纳米材料(如石墨烯和 CNT)广泛结合以形成纳米混合结构。这样做提高了检测和诊断的电化学反应性,以满足未来对生物传感器的灵敏度和选择性等要求[14]。
这些碳纳米材料与 MON 的杂化提供了具有一种或多种功能的先进生物传感器的生产,这些传感器具有卓越的光学、磁性和电学特性 [14,15,16]。石墨烯及其衍生物可以很容易地与其他纳米材料结合,形成纳米杂化材料,以获得所需的电化学活性 [13, 17, 18]。例如,在许多应用中,石墨烯被认为是促进电子转移到蛋白质氧化还原反应的有用工具 [19]。然而,石墨烯在生物环境中的物理稳定性及其对细胞的毒性评估仍存在争议[20,21,22]。另一方面,与石墨烯不同,CNT 具有不同的光学特征,因为它们的手性变化使它们在光学生物传感应用中优于石墨烯 [23]。碳纳米管具有出色的电化学能力,易于化学改性,并具有像石墨烯一样的高表面积体积比 [24]。就表面特性而言,当暴露于环境中时,尽管石墨烯由于其单层二维性质而以其全部体积暴露,但在一维 (1D) CNT 的情况下,这种暴露受到限制 [25]。此外,在之前的研究中多次报道,与碳纳米管相比,石墨烯具有更高的生物分子传感和信噪比特性,因此具有更高的抗干扰选择性。这主要是由于具有高表面积的石墨烯的无金属石墨边缘。然而,由于残留金属催化剂的存在,基于碳纳米管的生物传感器中存在信号扰动等问题[25]。在上述所有方面,由石墨烯和/或碳纳米管结构组合形成的纳米杂化物可能在先进生物传感器的设计中发挥至关重要的作用,通过由它们形成复合结构来补偿两种材料的缺点将克服这些问题和检测可以最大化。利用 MON、石墨烯和 CNT 的复合结构所产生的合作,提供改进的信号放大和准备先进的生物亲和策略似乎是必不可少的,从而开发出改进的生物传感设备以满足未来的需求。因此,在本次审查的范围内,它一直专注于最近实现的 MON、石墨烯和基于碳纳米管的生物传感器。此外,还讨论了使用这些纳米材料(不仅是单独使用,还可以一起使用)在生产具有通过它们组合获得的优异性能的生物传感器中的关键作用。通过评估未来的期望和挑战,我们想为进一步研究提出另一种视角。
基于金属氧化物纳米结构的生物传感器
自 1954 年开始进行生物传感器研究以来,金属氧化物 (MO) 一直是传感器应用的重要候选材料 [26, 27]。 MO 可以以各种纳米形态合成,例如 NPs [28, 29]、纳米纤维 [30]、纳米球 (NSs) [31]、纳米棒 [32]、纳米管和纳米线 (NWs) [33]、纳米片 [34, 35] .除了形态多样性外,MONs 还具有以下优点:高表面积/体积比、无毒、良好的生物相容性、化学稳定性、优异的选择性、电子和声子限制、高催化效率、强吸附能力、物理化学界面特征 [36,37,38, 39,40]。此外,MON 可以通过相对简单且具有成本效益的方法生产,例如射频 (RF) 磁控溅射 [41,42,43]、热蒸发 [44、45]、等离子体增强化学气相沉积 (PECVD) [46 , 47]、分子束外延 [48] 和溶胶凝胶技术 [49]、电化学沉积工艺 [50] 和水热法 [51]。这些显着特征使 MON 成为生物医学应用和生物传感器市场最需要的材料之一。对 2010 年至 2020 年关于 MON 的出版物进行了分析,如图 2 所示,饼图表示 MON 的生物医学应用分布。
<图片>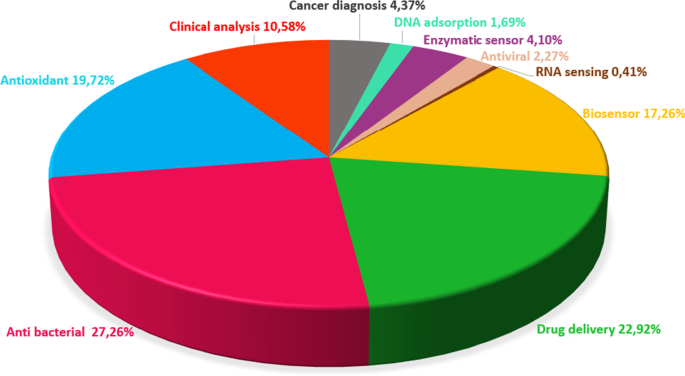
生物医学应用中MONs分布的饼图
图>另一方面,主要是近年来,各种 MON,如 ZnO、Fe3O4、CuO、NiO、TiO2、MgO 已经作为多功能和功能性生物传感器长期连续生产 [44, 52]。在MONs中,ZnO和Fe3O4因其广泛的应用而被认为是生物传感器构建中的重要成员[53, 54]。
氧化锌纳米结构
ZnO 纳米结构由于其独特的性质,包括高等电点 (IEP ~ 9,5) [55]、宽带隙、有用的电子通信特征、高化学稳定性、良好的生物相容性,在新型纳米结构生物传感器的制造中发挥着广泛的作用,和压电性。特别是,其高等电点清楚地解释了为什么 ZnO 是用于生物传感技术的最普遍的金属氧化物。此外,ZnO 可用于所有临床或非临床应用,因为它是环境友好且安全的材料 [53, 54, 56]。例如,Akhtar 等人。 [57] 利用具有更大表面积的花状 ZnO 纳米结构,开发了一种基于荧光增强机制的无试剂光学生物传感器,用于淀粉样蛋白检测,用于诊断神经退行性疾病,如阿尔茨海默病和胰岛素依赖型 II 型糖尿病.此外,据报道,ZnO 纳米花是一种良好的性能增强材料,可提供更快且具有成本效益的淀粉样蛋白生物传感器 [57]。此外,Zong 和 Zhu [54] 通过水热法制造了一种使用基于 ZnO 纳米棒的场效应晶体管 (FET) 的葡萄糖生物传感器,该传感器与糖尿病患者的可穿戴连续血糖监测应用相关。他们实现了具有 1.6 mA/µM cm 2 高灵敏度的高性能生物传感器 具有 180 µm 的微小感应区域 2 在 ZnO 纳米棒的大表面积与体积比的优势下,检测限为 1 µM [54]。萨哈尔等人。 [58] 开发了一种新的基于 Ag 掺杂 ZnO NPs 的生物传感器,用于早期检测肉类腐败。他们用酶黄嘌呤氧化酶 (XO) 修饰的电极(纳米银-ZnO/聚吡咯(PPy)/铅笔石墨电极)分析的结果表明,他们获得的酶生物传感器显示出高选择性,灵敏度为 0.03μA/mM,并且0.07 μM 检测下限 [58]。
在另一项研究中,Yue 等人。 [59],成功开发了一种基于 Au NPs-ZnO 纳米锥阵列/石墨烯泡沫电极的理想多巴胺 (DA) 生物传感器。在他们的表征中,他们证明他们修改的电极具有高灵敏度(4.36 μA μM -1 ) 和检测 DA 时的低检测限 (0.04 μM, S/N =3)。此外,他们报道了基于 ZnO 纳米锥的电极在尿酸 (UA) 干扰下表现出优异的选择性、良好的重现性和稳定性。他们还强调,该电极在医学和保健方面具有巨大潜力[59]。同年,钱等人。开发了一种使用 ZnO NPs 的电化学葡萄糖检测器。该传感器由用 ZnO NPs 装饰的 CeO2 纳米晶须组成,他们表示 ZnO/CeO2 纳米复合结构具有广泛的表面积、无毒和高电催化活性。该纳米复合材料在检测葡萄糖方面表现出非凡的性能,线性范围为 0.5-300 μM,检测限 (LOD) 为 0.224 μM (40 ppb)。他们还强调,纳米复合传感器在电流信号强度和葡萄糖浓度(R 2 =0.99944) [60]。另一种葡萄糖生物传感器是由 Rafiee 等人开发的。 [61] 通过结合以高导电性和化学稳定性而闻名的石墨烯纳米片 (GNP) 和已知对葡萄糖敏感的 ZnO NW。在他们的研究中,他们通过在三种不同浓度(0.5、1 和 2 毫克)(定义为 GNP1、GNP2 和 GNP3)的 GNP 薄膜上合成 ZnO NW 来修改设备的结构,如葡萄糖生物传感器。该系统表明,ZnO NWs 和 GNPs 的双重作用导致了高效葡萄糖生物传感器的完美改进。例如,他们指出,对于低葡萄糖浓度,设备的响应随着溶液中石墨烯量的增加而增加,并且传感器响应时间随着 GNP 数量的增加而减少。此外,他们报告说,在暴露于 30 mg/dL 葡萄糖 30 天后,在用 GNP 修饰的样品中观察到长期稳定性,即对浓度关系的一致抵抗力,这是理想生物传感器的重要标准。因此,他们提出了一种具有有用特性的理想葡萄糖生物传感器:响应时间为 5 秒,检测范围为 0.003-30,000 毫克/分升,以及长期电稳定性 [61]。除了这些研究之外,表 1 还给出了最近使用不同 ZnO 纳米结构检测各种酶的其他一些研究。
图>考虑到表 1 中显示的当前研究,可以表示 ZnO 结构是通过多种方法生产的,具有不同的形态,并且由于易于集成到复合结构中而继续被广泛使用。生产替代方案和形态多样性,以及与其他纳米材料,尤其是碳纳米结构形成纳米复合材料和纳米混合结构,为 ZnO 结构提供了非凡的潜力,即在理想的生物传感器中充分高效地满足预期特性。
Fe3O4 纳米结构
近年来,Fe3O4纳米结构由于具有良好的生物相容性、低毒性、超顺磁性、催化活性和易于制备等优异特性,在许多有前景的应用中引起了广泛关注,包括生物传感器、药物输送、细胞分离和制药。和修改过程。磁性 Fe3O4 NPs 适用于固定所需的生物分子,如酶 [73,74,75,76],因为其磁性 [77] 具有与介质的简单分离能力。 Fe3O4 磁性 NPs 及其衍生物已广泛用于生物传感器技术,文献中讨论了各种有吸引力的研究 [75, 78]。在这种情况下,Sanaeifar 等人。 [75] 设计了一种用于葡萄糖检测的新型电化学生物传感器。他们评估了通过在聚乙烯醇 (PVA) 中通过共沉淀法生产的 Fe3O4 磁性纳米颗粒分散制备的纳米复合材料的电化学性能。他们报告说,PVA 基质中的 Fe3O4 NPs 对固定化葡萄糖氧化酶具有优异的催化性能,增加了酶和电极表面之间的电子转移速率。制备的生物电极可在5 \(\times \hspace{0.17em}\)10 −3 范围内测量葡萄糖 至 30 mM,灵敏度为 9.36 µA mM −1 并显示检测限小于 8 µM [75]。董等人。 [79] 开发了基于 Ag/Fe3O4 核-壳 NSs 的传感器,通过简单的溶剂热方法生产,用于检测肼以保护环境。他们报告说,高性能肼传感器的响应时间为 2 秒,线性范围为 0.25–3400 µm,灵敏度为 270 µA mM − 1 厘米 − 2 ,检测限为 0.06 μM。比较这些数字,开发出一种远远优于文献中其他传感器的肼传感器[79]。
在另一项研究中,Sriram 等人。 [80] 开发了 Fe3O4 NSs/还原氧化石墨烯 (rGO) 纳米复合材料来检测尿液和血清样品中的 UA。电化学分析结果表明,Fe3O4 NSs/还原氧化石墨烯(rGO)纳米复合材料具有高稳定性和可重复性,显示出优异的电化学还原峰。此外,他们强调他们开发的 UA 传感器的线性范围在 0.02 到 783.6 µM 之间,LOD 为 0.12 nM [80]。同样,Cai 等人开发了一种通过结合氧化石墨烯 (GO) 和 Fe3O4 来检测 DA 的新型生物传感器。 [81]。在他们的研究中,他们通过分散和共沉淀方法成功合成了 Fe3O4/GO/原始石墨烯 (PG) 三元复合材料。后来,他们通过滴落技术将纳米复合材料沉积在工作电极玻璃碳电极 (GCE) 上。在循环伏安图 (CV) 中记录了 Fe3O4/GO/PG 结构的最高峰值电流。同样,他们报告说,存在 DA 时的最高峰值电流属于 Fe3O4/GO/PG/GCE 样品。他们还强调了由于 DA 浓度增加,Fe3O4/GO/PG/GCE 样品的峰值电流增加。最后蔡等人。指出电化学传感器可以有效地用于 DA 检测 [81]。 Fe3O4纳米结构作为生物传感器组件的一些代表性研究见表2。
图>尽管具有优异的性能,磁性 Fe3O4 纳米结构在生物传感器和生物应用中存在限制性问题。由于它们的高表面能、化学反应性和强磁性相互作用,它们极易团聚,难以稳定 Fe3O4 磁性纳米结构。为了克服这个问题,Fe3O4 纳米结构的表面涂有聚合物层[95]。然而,用聚合物涂覆表面可能会降低电化学生物传感器应用的效率。因此,在稳定磁性 Fe3O4 纳米结构时,可以使用生物分子,如基因、细胞、酶、蛋白质和其他必需的纳米结构(石墨烯、碳纳米管、量子点、纳米粒子等)。因此,可以预测,基于磁性Fe3O4纳米结构的复杂纳米杂化和纳米复合体系将成为未来新一代生物传感器生产的一种现象。
毕竟,结合各种纳米结构的基于 MO 的生物传感器在实际和工业应用中呈现出独特而新颖的功能。由于其无与伦比的特性,MO 的纳米结构极大地影响了设计高度灵敏、快速和稳定的生物传感器。此外,每种金属的纳米结构和氧化物都有其优点。因此,传感设备的新进步很可能发生在生物技术中。此外,可以看出纳米碳结构在最近的研究中获得了很大的空间,并且 MO 与它们一起使用。因此,本工作的第二部分将重点研究生物传感器中最常用的两种纳米碳(石墨烯和碳纳米管)。
石墨烯及其衍生物生物传感器
石墨烯是最流行的碳同素异形体之一,就像石墨、碳纳米管、富勒烯、金刚石一样。是sp 2 的二维层 -杂化碳原子。 Geim和Novoselov发现石墨烯后,它在透明电极、储能、药物输送、生物传感器、超级电容器、电池和催化等各个学科引起了全世界的广泛关注[96, 97]。石墨烯与许多其他纳米材料一样,可以通过自上而下(机械剥离、化学剥离和化学合成)和自下而上的方法(热解、外延生长、化学气相沉积 (CVD))合成 [97]。不同的生产方法导致大量石墨烯类材料的存在,例如石墨烯、GQD、GO、rGO、石墨烯纳米带(GNR)、纳米网、纳米片 [98]。常用的衍生品如图3所示。
<图片>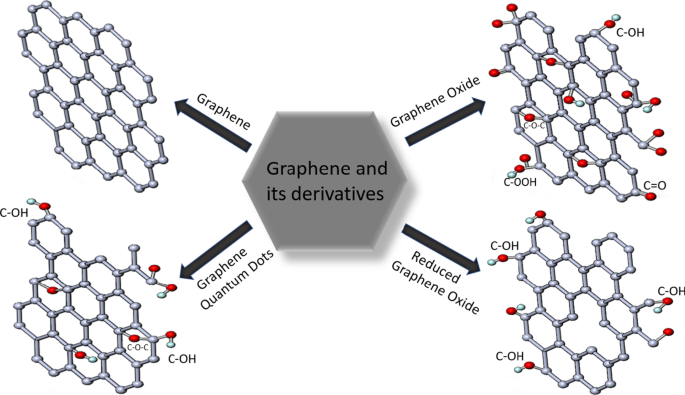
最流行的石墨烯基材料的结构
图>石墨烯具有良好的导热性 (5000 W/mK)、室温下的高电子迁移率 (250,000 cm 2 /V s),大表面积 (2630 m 2 /g)、高弹性模量 (21 T Pa) 和良好的导电性 [99]。此外,石墨烯片的原子厚度及其高表面积提供了材料对条件变化的敏感性。因此,石墨烯的表面特征,其中每个原子都可以直接接触,使其对环境敏感。因此,与其他材料 [, , 4, 100, 101] 相比,它是传感器应用的绝佳候选者。对过去十年中与石墨烯及其衍生物相关的研究进行了分析,并在图 102 中呈现了一个饼图,该饼图呈现了石墨烯的生物医学应用的分布。可以说,由于上述石墨烯的特性,研究人员主要关注生物传感器领域。
<图片>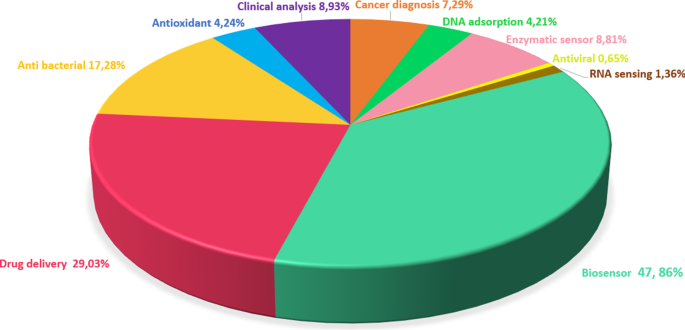
石墨烯在生物医学应用中的分布饼图
图>如第一部分所述,一些生物传感器是通过将石墨烯和石墨烯衍生物与 MON 结合来制备的。在这部分评论中,我们专注于基于石墨烯及其衍生物的生物传感器。基于石墨烯的生物传感器的一般表示和机制如图 5 所示。在这里,分析物与石墨烯表面上的官能团相互作用,基于这种相互作用可以获得电化学、光学或其他输出 [96 , 97, 103]。例如,Mani 等人。 [104] 开发了一种基于 rGO 纳米带/MWCNTs/壳聚糖的三元纳米复合材料,用于灵敏和选择性检测 H2O2 和 NO2 − .他们探索了生物传感器在隐形眼镜清洁液和肉类样品中的有益特性。他们报告说,对于 H2O2,基于纳米生物复合材料的传感器的灵敏度为 0.616 µAµM -1 cm −2 ,检测限为 1 nm,线性范围为 0.001–1625 µM,而 NO2 − 的这些值 , 0.643 µAµM −1 cm −2 、10 nm 和 0.01–1350 µM,分别。因此,他们证明了基于石墨烯的传感器可以有效地用于医疗应用和食品安全 [104]。 Yin 等人 制备了另一种基于石墨烯的 H2O2 传感器。 [105]。在他们的研究中,Yin 及其同事使用水热法合成了用 Ni3N NPs 装饰的导电三维 (3D) 石墨烯气凝胶 (GA)。作为他们表征的结果,他们表明他们获得的 Ni3N/GA 复合材料不仅可以用于 H2O2,还可以用于葡萄糖测定。他们报告说,基于 Ni3N/GA 的电极在测定 H2O2 时表现出很高的电化学性能,检测范围为 5 µM–75.13 mM,灵敏度为 101.9 µAmM -1 cm −2 ,以及 1.80 µM 的低检测限。此外,对于葡萄糖测定,他们强调设计的电极的检测范围为 0.1–7645.3 µM,检测限为 0.04 µM,灵敏度为 905.6 µA mM -1 cm −2 [105]。
<图片>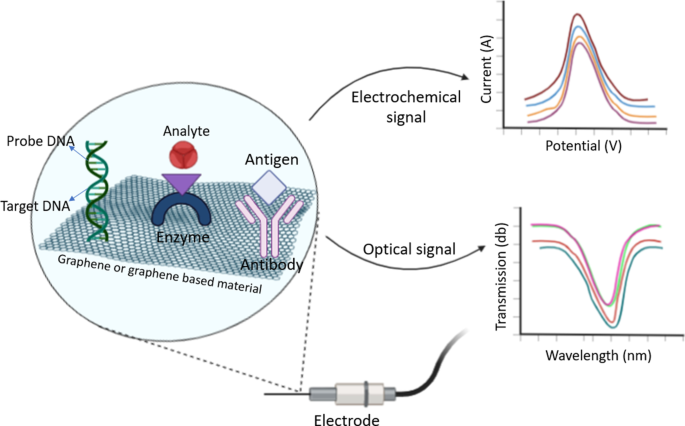
基于石墨烯的生物传感器的表征及其机理
图>可以说,最近人们对基于石墨烯的生物传感器用于葡萄糖的实际检测产生了浓厚的兴趣,如表 3。例如,Đurđić 等人。 [106] 通过共沉淀成功合成了一种基于 Bi2O3 装饰的 GNR 的一次性生物传感器。由于他们的表征,他们证明他们获得的传感器的检测限为 0.07 mM,线性范围为 0.28-1.70 mM,灵敏度为 64.81 μA/mMcm 2 .因此,他们提出基于石墨烯的传感器可以检测血清和尿液样本中的葡萄糖,可重现且稳定[106]。同年,Lu 及其团队通过单锅水热合成 3D 掺氮多孔石墨烯水凝胶 (NHGH) 与 NiCo2O4 纳米花 (NHGH/NiCo2O4) 成功设计了有用的葡萄糖生物传感器。他们用他们获得的纳米复合材料修改了 GCE,并评估了修改后的电极在测定葡萄糖方面的电化学性能。首先,他们在 0.1 M NaOH 溶液中接收 CV,扫描速率为 50 mV s −1 , 检查电化学催化性能。他们报告说,NHGH/GCE 的氧化峰值电流比裸 GCE 的弱阳极峰值电流增加了 0.5 V。此外,在他们的研究中,他们观察到氧化还原峰对是可见的,这表明与其他电极相比,NHGH/NiCo2O4/GCE 的电化学活性最高。他们将这种改进归因于石墨烯的扩展表面积、 良好的导电性,以及 Co 和 Ni 的氧化还原反应。此外,他们还展示了电极在 5.0 mM 葡萄糖添加中的电化学催化性能。他们将 NHGH/NiCo2O4/GCE 的最高峰值电流解释为 0.5 V,这清楚地表明,由于 NiCo2O4 和 NHGH 的双重作用,葡萄糖氧化可以比其他电极更好地催化。 They also reported that the peak currents increased linearly with increasing glucose concentration and the NHGH/NiCo2O4-based glucose sensor exhibited a broad linear relationship between peak current and glucose concentration in the range of 5 μM–2.6 mM and 2.6 mM–10.9 mM, respectively. Also, they emphasized that NHGH/NiCo2O4/GCE has a high sensitivity (2072 μA mM − 1 cm − 2 ) and a low detection limit (0.39 μM). As a result, they suggested using for a precise determination of glucose in real blood samples [107].
图>As seen in Table 3, graphene and its derivatives have become an indispensable building block for biosensor applications, because of its excellent properties. Considering the studies performed recently Table 3, it is remarkable that graphene and its derivatives are used in hybrid nanostructures with MONs to improve biosensors' sensitivity and reproducibility. Additionally, MONs/graphene synergy should be evaluated to obtain multifunctional biosensors and achieve high electrocatalytic activity. Moreover, graphene can be easily combined with other nanocarbons such as CNTs. Therefore, rich edge density and highly beneficial edge defects for creating enzymatic biosensors can be obtained.
Carbon Nanotubes-Based Biosensors
CNT's, discovered by Iijima in 1991, can be conceived as the formation of a graphene layer into a cylinder. CNTs can be categorized in general two types as single-walled carbon nanotubes (SWCNTs) Fig. 6a and MWCNTs Fig. 6b [125]. The diameter and wrapping angle determine the physical features of the CNTs by chirality and the (n, m) index [126,127,128]. According to the (n,m) index, CNTs can exhibit metal or semiconductor behavior [129,130,131,132],depending on chirality, SWCNTs may be classified in three different ways:(1) m = n is the armchair nanotube Fig. 6c, (2) n > m and if m = 0 is the chiral nanotube Fig. 6d, and m = 0 is the zig-zag nanotube Fig. 6e. CNTs display the semiconductive behavior in their nature, but for a given (n , m ) SWNT, when (2n + m )/3 is an integer, the CNTs will be metallic. Thus, it can be claimed that all armchair nanotubes are metallic [130]. Therefore, the ability to control chirality during production means to control the electronic features of CNTs, which provides a great advantage in biosensor applications. Several different methods have been proposed to synthesize CNTs in recent years. However, there are three main synthesis techniques (arc discharge, laser ablation, and CVD for CNTs production [133]. Compared to arc-discharge and laser ablation methods, CVD is the most effective method for simple and cost-effective controlling the chirality of CNTs [133, 134].
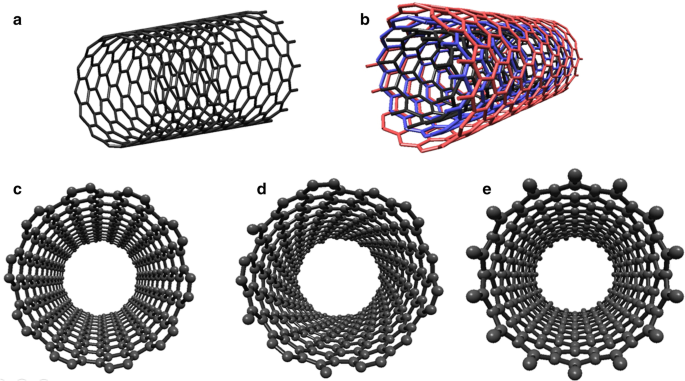
The classification of the CNTs of a SWCNT, b MWCNT; Schematic representation of three typical types of SWCNTs c Armchair (10, 10), d Chiral (13, 6), and e Zigzag (14, 0)
图>The ends and sidewalls of the CNTs can be easily modified by the addition of virtually any desired chemical species. CNTs can be excellent transducers in nanoscale sensors owing to their significant sensitivity. Additionally, CNTs have very favorable properties for transmitting electrical signals generated upon recognition of a target and therefore play an essential role in the final development of enzyme-based biosensors [135]. Moreover, CNTs with small size, fast response times, and excellent electrochemical properties are equal or superior to most other electrodes with their ions, metabolites, and protein biomarkers [136]. As a result of their unique tubular nanostructures with extensive length and diameter ratios, CNTs are desirable materials in applying electrochemical biosensors due to their excellent electrochemical stability, great mechanical flexibility, rapid electron transport, and unique thermal conductivity [137, 133]. CNTs are also widely used in tissue engineering and drug delivery systems to improve electrical and mechanical features after being functionalized to ensure their biocompatibility and conjugated with organic compounds or metallic NPs. [138]. Studies on CNTs from 2010 to 2020 were analyzed and are presented in Fig. 7 as a pie chart that shows the distribution of biomedical applications of CNTs.
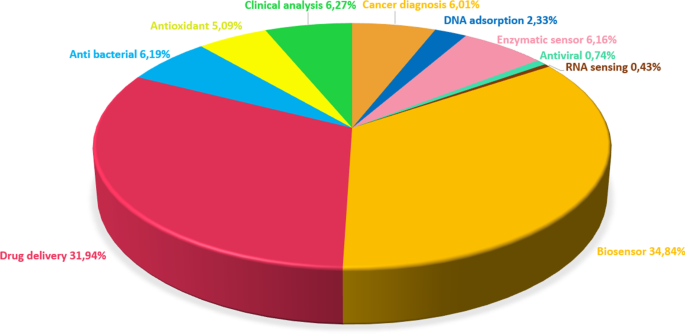
Pie chart showing the distribution of CNTs in biomedical applications
图>CNTs, as with graphene and its derivatives, also make important contributions to the development of biosensors with higher sensitivity and selectivity by hybridizing with MONs. Researchers have recently focused on the production and characterization of new nanobiosensors that can combine the unique properties of CNTs with the superior properties of metal NPs. For instance, Rahman et al. [139] designed the Fe3O4-decorated CNTs based 3-methoxyphenyl (3-MP) biosensor for environmental protection applications. Fe3O4/CNTs nanocomposites synthesized by wet-chemical method and coated the nanocomposite on the GCE surface as a thin layer. Then, they evaluated the electrochemical performance of the modified electrodes by I-V characterization and reported that the Fe3O4/CNT-based electrode showed a wide detection range (90.0 pM–90.0 mM), low detection limit (1.0 pM), and high sensitivity (9 × 10 −4 μA μM −1 cm −2 ) in detecting dangerous phenol [139]. Similarly, for environmental protection, MWCNT/TiO2/chitosan-based biosensor was developed by Fotouhi et al. [140] to detect dihydroxy benzene isomers released into the environment from the chemical and pharmaceutical industries. Fotouhi et al. reported that they performed the simultaneous determination of hydroquinone (HQ), catechol (CC), and resorcinol (RS), causing pollution in real water samples by the MWCNTs-based sensor. Additionally, they indicated the detection limits (S /N = 3) of HQ, CC and RS, as 0.06 μmol d m −3 , 0.07 μmol d m −3 , and 0.52 μmol d m −3 , and the linear response ranges are between 0.4–276.0 μmol d m −3 , 0.4–159.0 μmol d m −3 , and 3.0–657 μmol d m −3 , respectively [140].
Besides environmental protection, biosensor designs of CNTs for clinical applications have recently become extremely interesting Table 4. For instance, Zhu et al. [141] obtained the buckypaper containing two layers:purified SWCNTs and SWCNTs decorated with NiO, by helium arc discharge method. Later, as a result of their analysis to evaluate its electrochemical performance, they showed that glucose biosensor has a broad linear range (0.1–9 mM), high sensitivity (2701 μA mM −1 cm −2 ), and fast response time (< 2.5 s) [141]. Barthwal and Singh [142] designed a ZnO/MWCNTs nanocomposite biosensor to detect urea in their study. They indicated that the ZnO/MWCNTs-based sensor has the highest detection characteristics compared to the ZnO and MWCNTs-based sensor. Also, they emphasized that the nanocomposite's sensitivity containing 2% MWCNTs is less than 10 s, and the detection limit is 10 ppm [142]. In the same year, Guan et al. successfully developed a CNTs-based hybrid nanocomposite as an electrochemical biosensor for simultaneous high-sensitivity detection of DA and UA. In their study, they reported that the most extensive (ΔE p = 144 mV) and highest oxidation current was observed in the electrode modified with CNTs-based nanohybrid. Additionally, they investigated the simultaneous detection of DA and UA in nanohybrid-modified GCE via differential pulse voltammetry (DPV). They showed that the anodic peak current response of the nanohybrid/GCE increased linearly due to the increase in DA concentration. Also, they obtained a similar observation for the UA concentration. They emphasized that the concentration range for both target analytes is 2–150 μM. As a result, they reported that the limit of DA and UA detection values was 0.37 μM and 0.61 μM, respectively [143].
图>Studies on increasing the efficiency of CNTs-based biosensors in different application areas by hybridizing with MONs and graphene and graphene derivatives and improving their properties are of great interest Table 4. The higher electrochemical activity and higher conductivity of nanohybrid structures designed with CNTs-based electrochemical sensors can be considered a result of the inherent properties of CNTs. On the other hand, one of the features that limit the use of CNTs in biosensor applications is that they are not dissolved in most solvents. Also, it has low biocompatibility and, in some cases, toxicity. To overcome these problems, combining different functional groups on the surface and end caps of CNTs with MONs, and applying surface modifications can be considered as a solution.
Additionally, due to the integration of CNTs with graphene and its derivatives, it is possible to create more active sites for biomolecules due to strong binding interactions. Another advantage of CNTs/graphene hybrid structure is that it allows biosensors to respond in a shorter time due to their higher electron transfer rate. Thus, in the next generation of biosensors to be developed in the future, it seems inevitable to achieve high sensitivity and selectivity, simultaneous target biomolecule detection by benefiting from the dually effect of CNTs with MONs or other nanocarbons such as graphene and its derivatives.
Conclusion and Outlook
Biosensors and bioelectrodes play a crucial role in environmental monitoring, food safety, the medical textile industry, drug discovery and analysis, clinical and nonclinical applications. With the recent COVID-19 pandemic, fast responsive, reusable, cheap and highly selective biosensors became crucial for the fight against infectious diseases to be taken under control. For the design of a biosensor, the material used in transducer component and to functionalize transducer surfaces has an explicit effect on the results with aforementioned properties obtained from a biosensor. Within this frame, for the improvement of the properties of these devices, nanomaterials have been extensively used and their expanded surface area, ability to adapt to the surface modifications for the use of any type of analyte, and such extraordinary nanosize-dependent properties brought them one-step ahead unprecedently in the production of an ideal biosensor.
With this motivation, this paper presents an overview on recent developments in hybrid nanosystems created by the combined use of MONs, graphene, and CNTs. Numerous efforts have been made to create biosensors with improved sensitivity and selectivity to detect biomolecules with the help of these nanostructures. Obviously, apart from each of these materials’ unique characteristics, the multiple effect of hybrid design of them is a key point in obtaining a higher performance biosensor. Combining these nanostructures to create a hybrid design improves the biosensor's electrocatalytic activity, its electron transfer rate, and enables more active sites to allow two or more biomolecules to be detected, simultaneously. It also meets other desired functions expected from an ideal biosensor, such as stability, long shelf life, repeatability, wide measuring range, fast response time for next-generation biosensor applications. However, there are compelling factors in combining these three trending nanomaterials, such as the control on agglomeration tendency, cytotoxicity, the choice of the right concentration, and the extensive optimization of conditions to improve purity and these materials better integration with each other. Therefore, there are still open allowance for improvements to be made for the preparation of nanomaterials and their composite structures. Furthermore, for an onsite diagnosis of an analyte, having a major impact for biosensors for medical applications, it is important to have a quick and reliable result in a cost-effective way. For this purpose, nanomaterials used in biosensors might be modified to facilitate diagnosis with more delicate sensing especially for the biomarkers of some diseases with a very minute concentration at their early stages. For gaining and improving such features, graphene, CNTs and MONs, should be produced with minimum catalyst impurities, high crystallinity, and in massive amounts in a cost-effective way. They should also be engineered for their density of states and the structure of bonds for tailoring a better electron transport properties. Within this review, a combination of nanostructures that help to develop an accurate 'future biosensor' mechanism was proposed and expectations as sensitivity, superior selectivity, low limit of detection, real-time sensing with multi-functional properties were summarized.
数据和材料的可用性
不适用。
缩写
- 1D:
-
One-dimensional
- 3D:
-
三维
- CD:
-
Cyclodextrin
- CVD:
-
化学气相沉积
- 简历:
-
循环伏安图
- DA:
-
多巴胺
- DNA:
-
脱氧核糖核酸
- DPV:
-
微分脉冲伏安法
- FET:
-
场效应晶体管
- GCE:
-
玻碳电极
- GelMA:
-
Gelatin methacryloyl
- GNPs:
-
Graphene nanoplatelets
- GNRs:
-
Graphene nanoribbons
- 开始:
-
氧化石墨烯
- GQDs:
-
石墨烯量子点
- 细节层次:
-
检测限
- MIP:
-
Molecularly imprinted polymer
- MOF:
-
Metal organic frameworks
- MOs:
-
Metal oxides
- MWCNTs:
-
多壁碳纳米管
- NHGH:
-
Nitrogen-doped porous graphene hydrogel
- NP:
-
纳米粒子
- NR:
-
Nanorods
- NSs:
-
Nanospheres
- NWs:
-
纳米线
- PANI:
-
聚苯胺
- PDADMAC:
-
Poly(diallyl dimethyl ammonium chloride)
- PDPA:
-
Poly Diphenylamine
- PECVD:
-
等离子体增强化学气相沉积
- PEDOT:
-
Poly(3,4-ethylenedioxythiophene)
- PG:
-
Pristine graphene
- PPy:
-
聚吡咯
- PTA:
-
Phosphotungstic acid
- PVA:
-
聚乙烯醇
- RF:
-
射频
- rGO:
-
还原氧化石墨烯
- SERS:
-
表面增强拉曼散射
- SWCNTs:
-
单壁碳纳米管
- UA:
-
尿酸
- XO:
-
Xanthine oxidase
纳米材料