银纳米结构的合成方法和应用的最新进展
摘要
作为先进的功能材料,银纳米颗粒主要基于其各种性质,在光电、生物传感、催化、抗菌等领域具有潜在的应用价值。然而,银纳米粒子的性质通常由它们的大小、形状和周围介质决定,这些可以通过各种合成方法进行调节。在这篇综述中,详细说明了合成不同形状和特定尺寸的银纳米粒子的制备方法。此外,本文还讨论了银纳米粒子的相应性质和应用。
背景
在过去的几十年中,具有独特光学和电学特性的金属纳米粒子得到了广泛的研究。银纳米粒子 (AgNPs) 是研究最深入的金属纳米粒子,因为它们具有独特的性质和应用 [1,2,3,4,5]。 AgNPs 的特性在很大程度上取决于粒子的形态,包括形状、大小和周围介质。人们在银纳米粒子的合成方法和形态调控方面做了大量工作。
近期,研究人员对银纳米粒子的光电[6]、催化[7]、抗菌[8、9]、生物传感器[10]、表面增强拉曼散射(SERS)等优异功能进行了深入研究。 ) [11]。目前,AgNPs已通过化学还原[12,13,14,15,16]、光还原[17、18]和激光合成[19]等方法成功制备,但这些方法通常耗时耗能。同时,它们也存在制备条件严格和AgNPs尺寸不均匀的缺点。因此,迫切需要开发简单、经济的方法来精细控制 AgNPs 的尺寸、形状和尺寸分布。利用保护剂是使 AgNPs 具有良好稳定性和分散性的有效方法。同时,通过保护剂可以防止颗粒之间的团聚。因此,保护剂对于AgNPs的合成非常重要[20]。
在这项工作中,详细回顾了不同形状的银纳米粒子的制备,如纳米立方体、纳米线和纳米球。之前已经回顾了制备具有不同形状和尺寸的 1-10 nm AgNPs、10-100 nm AgNPs 的银纳米粒子的代表性工作。由于其优良的环保特性和简单的操作,获得银纳米粒子的新生物合成方法可以作为复杂化学合成程序的替代方法被选为重点。同时,对AgNPs的抗菌、荧光、催化、表面等离子共振等特性和应用进行了详细综述如下。这篇综述强调了银纳米粒子在纳米传感器中的重要应用。
这项研究提供了一种对 AgNPs 研究具有重要意义的综合方法。但值得注意的是,创新的制备方法和应用突破仍有待探索。
合成方法
银纳米粒子的合成方法多种多样,如种子生长法[21]和逐步还原法[22]。每种方法都有优点和局限性。因此,开发一种有效的制备方法仍然是一个挑战。由于其独特的性质和广泛的应用,银纳米粒子的合成方法值得优化。我们在这项工作中总结了六种类型的制备方法,包括新的生物合成方法。希望能为从事该领域的工作者提供一点帮助。
不同类型 AgNPs 的制备
最近,由于 AgNPs 的形态相关特性,研究人员专注于其形状控制 [23, 24]。同时,为了扩大其目前的应用,制备不同形状(如珊瑚状[25]、笼状[26]和三角形纳米晶[27])的银纳米粒子引起了广泛的科学研究。长期以来,人们对银纳米粒子的形成机理和不同的制备方法进行了探索。
银纳米立方体的合成
夏等人。 [28,29,30] 在聚乙烯吡咯烷酮 (PVP) 存在下,通过用乙二醇还原硝酸银,大量制备银纳米立方体的单分散样品。在合成过程中,PVP用作保护剂,可以稳定分散的银纳米粒子并防止团聚。同时,PVP的添加量也会影响AgNPs的形貌。因此,在合成过程中使用 PVP 是必不可少的。众所周知,加热可以提供更多的反应能量,有利于提高乙二醇的还原性。在氢氧根离子存在下,Ag + 被还原形成银纳米立方体。这项研究的优点是可以用来制备均匀的单晶纳米立方体。在纳米尺度上,其中大部分为面心立方 (fcc) 的金属倾向于成核并生长成孪晶和多孪晶粒子 (MTP),因为它们的表面由纳米尺度上的最低能量小面包围 [31] .此外,这种结构有利于应用于光子学、催化和基于 SERS 的传感领域。图片(图 1)显示了银纳米立方体的 SEM、TEM 和 XRD 图像。这些银纳米立方体的平均边长为 175 nm,标准偏差为 13 nm。它们的表面很光滑,这些颗粒的所有角落和边缘都被略微截断。这种结构可用于通过将药物注入截角的药物输送系统。
<图片>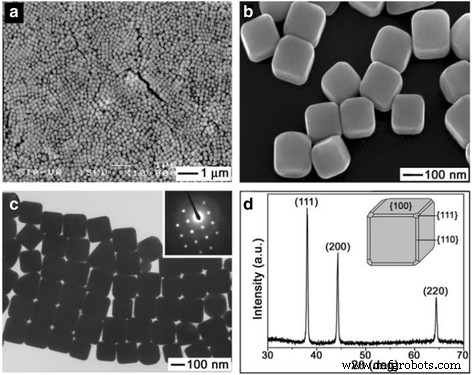
一 低-和b 略微截断的银纳米立方体的高倍放大 SEM 图像。 c 同一批银纳米立方体的 TEM 图像。 d 同批次样品的XRD图,证实形成纯fcc银[28]
图>Yam 等人发表了一种新型银纳米粒子。 [32]利用十六烷基三甲基溴化铵(CTAB)作为水溶液中的表面活性剂。溴离子可以与银氨络合物([Ag (NH3) 2] + )产生AgBr沉淀,银离子在后续反应中缓慢释放。同时,残留的银离子被葡萄糖还原,与包覆的表面活性剂形成了~ 55 nm的纳米银立方体。表面活性剂 CTAB 可以通过物理吸附作用吸附在 AgNPs 表面。因此,可以通过抑制有效地控制 AgNPs 的团聚和规模增长。由于CTAB的存在,可以获得分散均匀、尺寸合适的AgNPs。
Xia 和 Yam 合成方法报道的纳米银立方体的制备需要很长时间。但是银纳米粒子可以通过微波方法快速生产。萨拉夫等人。 [33] 在聚电解质和微波加热 60-120 秒的情况下,利用大量金种子制备银纳米立方体。实验表明,聚电解质引导粒子在特定的结晶方向上生长,从而产生多面粒子,即纳米立方体。目前,多元醇法制备纳米银颗粒较为成熟。
银纳米线和纳米棒的合成
墨菲等。 [34] 报道称,在银种子、胶束模板 CTAB 和 NaOH 存在下,使用抗坏血酸还原 AgNO3 可以成功制备纳米棒和纳米线。 Ag 种子的平均直径为 4 nm。在这项工作中,Ag + 的种子浓度和碱基相对浓度 在制造更大纵横比的纳米材料方面发挥关键作用。 CTAB 也是制备高产棒材所必需的。 TEM照片(图2)显示了纳米棒和纳米线的形状。
<图片>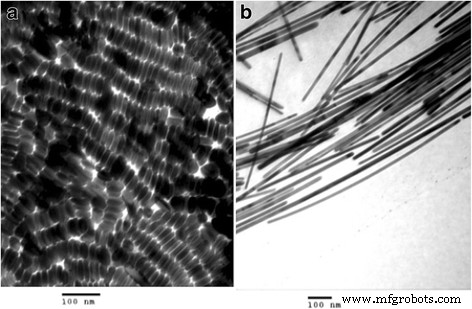
一 从具有 0.06 mL 种子的制剂中分离出形状分离的银纳米棒。 b 形状分离的银纳米线 [34]
图>银纳米棒由 Lee 等人制备。 [35]。在种子介导的生长方法中,首先制备小金属颗粒,然后将其用作制备纳米棒的种子。在作为稳定剂的柠檬酸钠二水合物存在下,通过用硼氢化钠还原银离子来制备银种子。将这些银种子加入含有更多银盐、抗坏血酸(弱还原剂)和 CTAB 的溶液中。在这项研究中,反应温度和 pH 值控制着所得棒的纵横比和均匀性。反应温度的升高导致银纳米棒的纵横比降低和单分散颗粒的尺寸增加。此外,pH 值的增加也显示出类似的结果。当反应温度和pH值升高时,银的还原率进一步提高。实验中,在30°C和pH 10.56的条件下合成了具有高纵横比和单分散性的银纳米棒。 Zhu等人在聚乙二醇(PEG)存在下,通过电化学方法从AgNO3水溶液合成银纳米棒。 [36]。结果表明,AgNO3和PEG的浓度对纳米棒的形成有影响。
墨菲等。提供了更好的制备银纳米线的方法,但 Sun [37, 38] 的合成方法更精细。他们通过在种子和 PVP 存在下用乙二醇还原 AgNO3 来合成银纳米线。反应机理如下:
$$ {2\mathrm{H}\mathrm{OCH}}_2\hbox{--} {\mathrm{CH}}_2\mathrm{O}\mathrm{H}\to {2\mathrm{CH}} _3\mathrm{CHO}+{2\mathrm{H}}_2\mathrm{O} $$ (1) $$ {2\mathrm{CH}}_3\mathrm{CHO}+{2\mathrm{Ag} \mathrm{NO}}_3\to {\mathrm{CH}}_3\mathrm{CO}\hbox{--} {\mathrm{COCH}}_3+2\mathrm{Ag}+{2\mathrm{HNO }}_3 $$ (2)接下来,将 AgNO3 和 PVP 加入反应体系中,使银成核和生长,形成均匀形状和尺寸的纳米线。通过这种方式生产了直径为 30-40 nm,长度可达 ∼ 50 μm 的银纳米线。本综述讨论了各种反应条件(温度、反应时间和晶种条件)对形态和尺寸的影响。图3为纯化后纳米线的形状和尺寸。
<图片>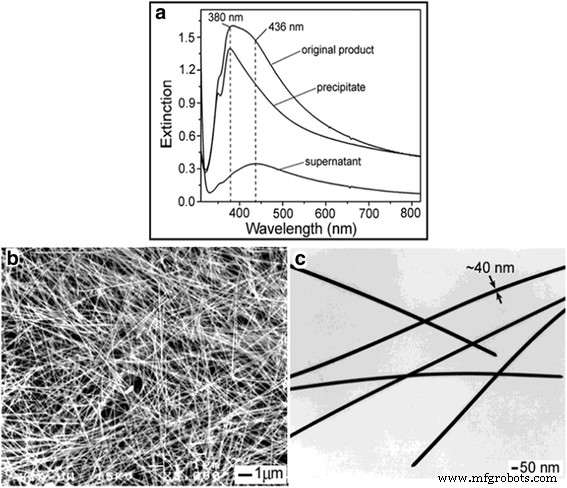
一 最终产品在 3 次离心和分离循环前后的紫外-可见消光光谱。 b SEM 和 c 银纳米线纯化样品的TEM图像[37]
图>在UV-vis光谱中(图3a),可以看出,与纯化后的原始样品相比,银纳米线在UV-vis吸收方面表现出明显的蓝移。 UV-vis 吸收峰出现在 380 nm。图 3c 显示这些纳米线的宽度为 40 nm。可以获得相同宽度的纳米线,这是这项工作的最佳优势。这些纳米线可用于制备导电薄膜[39]和高效有机太阳能电池[40]等。
通过进一步研究 PVP 如何与银纳米线反应,谢等人。 [41,42,43] 得出结论,PVP 单层通过 Ag-O 键与 Ag 纳米线反应。在此基础上,谢等人。 [44, 45] 在实验中观察到多重孪晶的存在,证明Xia对多重孪晶是形成Ag纳米线的关键因素之一。控制硝酸银的初始用量或降低硝酸银的初始还原速率,有利于溶液中银纳米线的形成[46、47]。他们采用的具体方法是通过向反应溶液中加入氯离子或降低银离子的释放速率来控制金属盐与硝酸银的反应。
唐等人。 [48] 通过在具有更高离子浓度的系统中添加不锈钢网,合成了尺寸可控的银纳米线。主要是不锈钢网可以与硝酸反应,有利于防止多晶粒的腐蚀。在氯离子存在下,他们通过水热法、微波法和其他实验方法制备了均匀的银纳米线 [49, 50]。硫化银纳米粒子是一种新型半导体,通过硫离子与银离子的反应很容易合成。硫化银纳米粒子可以提供电子,使银离子吸附在其表面,起到核心和还原剂的作用。同时,银原子也可以沉积在Ag2S表面形成Ag2S@Ag种子,起到自催化还原作用,有利于银纳米线的形成[51]。
银纳米球的合成
由于银原子在银纳米粒子的合成过程中容易形成球形结构,因此常采用化学还原法合成的准球形银纳米粒子被大量研究报道。在化学还原过程中,常用的还原剂包括硼氢化钠[52]、柠檬酸钠[53]、水合肼[54]、抗坏血酸[55]和氢气[56]。在所有的研究团队中,夏的团队是研究最详细、最全面的。为了获得高质量的单晶银纳米球 [57],他们采用了一种不同于化学还原的基于湿蚀刻的新方法。通过将均匀的 Ag 纳米立方体的悬浮液与少量硝酸铁或铁氰化物基蚀刻溶液快速混合,他们可以截断尖角和边缘以形成圆形纳米立方体或获得与原始直径相同的没有尖锐特征的纳米球立方体。因为以前的合成方法无法制备大于~ 35 nm 的均匀球体。值得注意的是,该方法可用于生产具有广泛尺寸范围的均匀 Ag 纳米球,并为 SERS 的基础研究开辟了新的可能性。在这项工作中,可以制备最小尺寸为 25 nm 和最大尺寸为 142 nm 的 Ag 球。图4表明银纳米立方体被蚀刻成准纳米球。
<图片>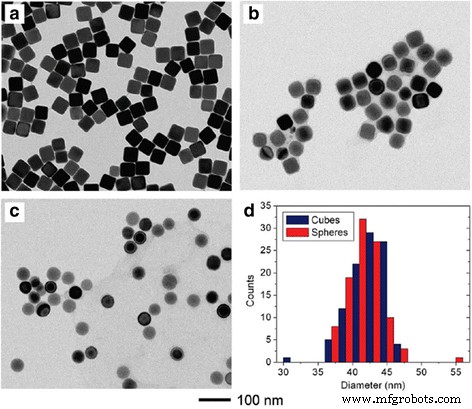
TEM 图像 (a–c ) 用不同体积的 0.5 mM 硝酸铁蚀刻时覆盖有 PVP 的 42 nm Ag 立方体。 一 0 微升。 b 10 微升。 c 100 微升。 d 从 a 部分中的 100 个粒子计算的粒度分布 和 c [57]
图>用这种方法制备的 AgNPs 具有规则的形状和均匀的尺寸。这些形状规则、尺寸均匀的银纳米球可用于制备均匀的金纳米笼,用于生物靶向给药[58]。
梁等人。 [59] 报道了一种制造单分散银纳米粒子的新技术。 PEG 既用作溶剂又用作还原剂,PVP 用作合成单分散银纳米粒子的封端剂。为了获得平均直径为 54 nm 的均匀纳米球,Liang 在 260°C 下使用了比例为 8 的 PVP/AgNO3。图5为银纳米球的TEM、HRTEM和XRD图。
<图片>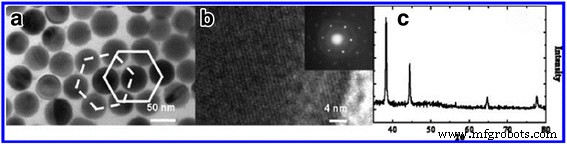
一 TEM 和 b 在 260 °C 下制备 24 小时的银纳米球的 HRTEM 图像,PVP 与 AgNO3 的摩尔比为 8,以及直径约 50 nm 的单个银纳米球的 SAED 图案(插图)。 c 同一批样品的粉末XRD图[59]
图>从TEM图像可以看出Ag纳米球的尺寸是均匀的。而且合成方法简单,可应用于批量生产。当然,关于球形纳米银的研究还有很多其他的论文也值得学习。但在这项工作中,我们不会重复它们。下一节,我们将描述三种制备不同尺寸银纳米粒子的方法。希望对从事规模和绩效影响研究的工作者有所帮助。
不同尺寸AgNPs的制备
众所周知,不同尺寸的银纳米粒子对材料的性能有显着影响。然而,我们发现很少有论文系统地描述不同尺寸的银纳米粒子的制备方法。所以,我们在下面的部分介绍了一些合成方法,希望它可以帮助那些想要得到确定尺寸的人。
1-10 nm AgNPs 的制造
小尺寸的银纳米粒子一般采用硼氢化钠作为还原剂的快速还原工艺制备,所制备的颗粒尺寸和形状不均一。谢哈尔等人。 [60]通过混合不同比例的硼氢化钠和柠檬酸钠作为还原剂(使用硼氢化钠优先减少快速成核和柠檬酸钠再次还原以保持稳定生长)制备了5-10 nm的银纳米粒子。通过这种方法,获得了均匀大小和形状的 AgNPs。下表1给出了合成不同尺寸银纳米粒子的设计条件。
图>林等人。 [61] 于 2003 年制备了 7-10 nm 形状和尺寸均一的银颗粒。描述了一种简单的合成方法,他们更喜欢直接制备窄分散的银纳米颗粒,而不是通过三氟乙酸银在异戊醚在油酸存在下。这种直接合成在综合上易于控制,并且能够获得直径在 7-10 nm 范围内且尺寸分布较窄的 AgNP。实验中使用有机溶剂中的单一来源前体,而不是使用涉及银盐前体和还原剂在溶剂中的传统方法。出于这个原因,他们选择三氟乙酸银作为单源前体,因为它很容易获得,并且可以在不同温度下热还原成银金属。最后,他们通过调整油酸与三氟乙酸银的摩尔比来改变 AgNPs 的直径。下面的图 6 显示了在油酸/三氟乙酸银摩尔比为 10:1 的持续时间 (A, B) 30, (C, D ) 90 和 (E, F) 150 分钟。
<图片>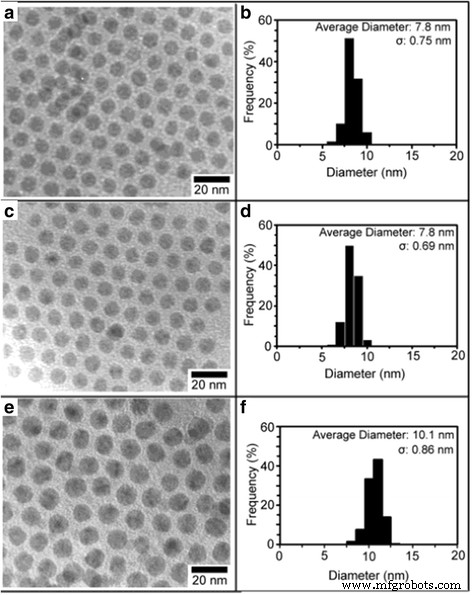
在油酸/三氟乙酸银摩尔比为 10:1 的持续时间 (a , b ) 30, (c , d ) 90 和 (e , f ) 150 分钟 [61]
图>Yang等人发现了一种在高浓度下合成直径小于10nm的单分散银纳米粒子的简单方法。 [62]。他们率先采用苯胺作为还原剂,十二烷基苯磺酸(DBSA)作为稳定剂的方法。在 DBSA 苯胺 AgNO3 系统中加入过量的 NaOH 后,在 90°C 下,银纳米颗粒的形成几乎在 2 分钟内完成(产率为 94%)。此外,所得银纳米粒子的平均粒径为8.9 ± 1.1 nm,胶体在常温下可保存1年以上。图7是AgNPs的TEM、DLS和XRD图。
<图片>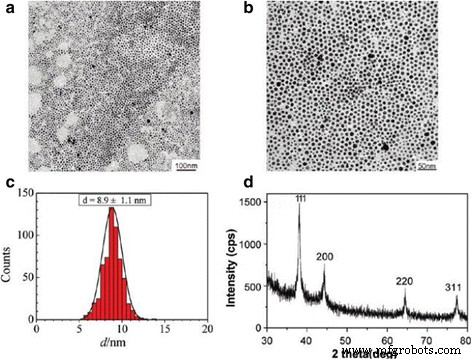
一 , b 在 90°C 下加入 NaOH 1 小时后,从反应系统中收集的银纳米粒子在两个放大倍数下的 TEM 图像。 c 银纳米颗粒尺寸分布的对应直方图。 d 银纳米粒子的XRD图谱[62]
图>上述合成小尺寸银纳米粒子的方法均在液相体系中。然而,郑等人。 [63] 在固相系统中合成了直径为 2-4 nm 的银纳米粒子。他们利用热还原法合成了发光和拉曼活性的银纳米粒子。图 8 为固相热解产生的 3 nm 银纳米粒子的尺寸分布、结构和发光。
<图片>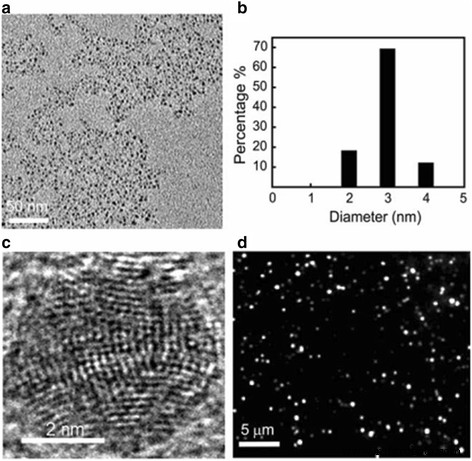
使用固相热解产生的 3 nm 银纳米粒子的尺寸分布、结构和发光。 一 这些纳米颗粒的低分辨率 TEM 图像。 b 由 TEM 确定的纳米颗粒的尺寸分布。 c 这种小银纳米粒子的高分辨率 TEM 图像显示出高度多域结构。 d 这些小的银纳米粒子在 ~ 10 W/cm 2 的 488 nm 激光激发下拍摄的发光图像 [63]
图>10–100 nm AgNPs 的制造
通过用 6 MeV 电子辐照,Bogle 等人合成了直径为 10-60 nm 的 AgNPs。 [64] 在硝酸银和 PVP 的混合物中。该方法具有制备效率高、产率高、副产物少等优点。阿比德等人。 [65]通过使用类似于上述工作的激光照射制备银纳米粒子。不同的是,他们利用十二烷基硫酸钠(SDS)作为封端剂与硝酸银混合,可以制备出尺寸为 13-16 nm 的银纳米粒子。粒径由激光强度和 SDS 表面活性剂的初始浓度控制。使用抗坏血酸还原,Qin 等人合成了尺寸为 30-72 nm 的球形银颗粒。 [66]。同时,随着反应体系的pH值从6.0增加到10.5,银纳米颗粒的尺寸减小。阿吉塔等人。 [67] 通过调节 PH 值利用化学还原获得 14-31 nm 的 AgNPs。他们使用乙醇作为溶剂,硼氢化钠作为还原剂,聚乙烯醇(PVA)作为封端剂。图9显示了这些银纳米颗粒的形成机理。
<图片>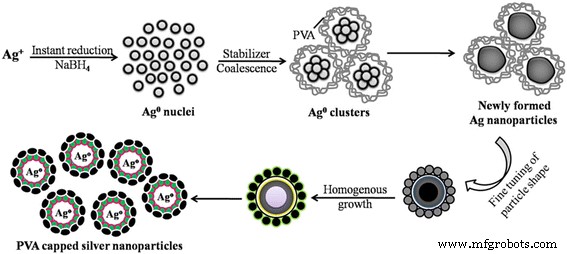
采用化学还原法合成尺寸控制的AgNPs示意图[67]
图>同样,直径为 15-21 nm 的 Ag 颗粒由 Silvert, P. Y. 等人合成。 [68] 谁利用乙二醇-PVP 溶液在特定温度条件下还原硝酸银。通过该方法合成了均匀的准纳米球。为了检测不同尺寸的银纳米颗粒的溶解度,Rui Ma 等人制备了 10-80 nm 的 Ag 颗粒。 [69]。他们通过多元醇法制备了分散胶体银,这是一种成熟的制备方法[70]。它们的制备方法基于 Silvert, P. Y,通过改变保护剂的类型。近来绿色合成研究十分火热,研究人员通常利用氨基酸或去皮还原Ag + 合成银纳米粒子。因为一种环保的合成方法可以克服物理和化学制备方法中产生有毒物质的问题。其中,Maddinedi 等人。 [71]利用酪氨酸作为还原剂和封端剂,通过将 PH 从 12 调整到 10 来制备 13-33 nm 的银颗粒。Mandal 等人。 [72]得到了相同的结果。他们使用香樟叶提取物作为还原剂和封端剂,通过调节香樟叶提取物的内含量来制备11-31 nm的银颗粒。图 10 显示了 AgNPs 的 TEM 和 SAED 图。
<图片>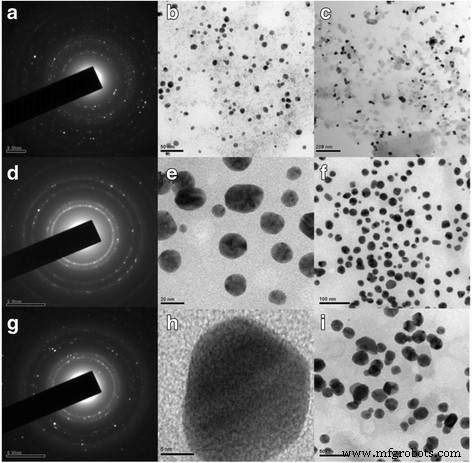
TEM 图像和胶体的 SAED 图案 叶提取物的体积 4 ml (Ct4) (a –c ), Ct3 (d –f ) 和 Ct1 (g –我 ) 纳米粒子 [72]
图>图11显示了不同叶提取物体积为1、3和4ml的AgNPs的动态光散射(DLS)。
<图片>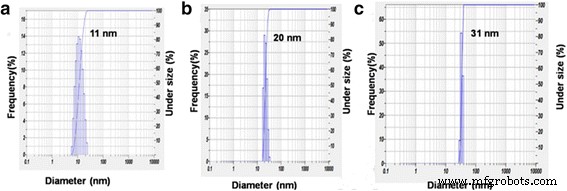
AgNPs Ct1 (a ), Ct3 (b ) 和 Ct4 (c ) [72]
图>当然,还有许多其他方法可以制备 1-100 nm 的银颗粒。以上论文仅为典型。我们做这项工作是因为我们希望帮助那些想要合成确定尺寸的人。综上所述,银纳米粒子的制备应朝着合成友好、尺寸可控的方向发展。
通过生物合成方法制备 AgNPs
使用生物系统合成金属纳米粒子已经发展成为纳米生物技术的一个重要领域。生物合成方法是制备 AsNPs 的更好候选方法,因为它们的制造过程采用了环境友好的技术,并且产品适合生物应用。在此,生物合成方法具有发展和研究的前景。所以,我们对一些综合案例进行了详细的讨论。 1999 年,克劳斯等人。 [73] 首次使用施氏假单胞菌 合成尺寸为 200 nm 的银纳米晶体。随后,利用其他菌株制备银纳米粒子得到了很大发展,如黄曲霉和木霉。和 Kazemi 等人。 [74] 利用 Geotricum sp. 成功合成了银纳米粒子。 Geotricum sp.在 Sabro 葡萄糖琼脂 (SDA) 培养基中,在 25 ± 1°C 下培养 96 小时。菌丝体用于将硝酸银溶液转化为纳米银。使用这些真菌(Geotricum sp.)在细胞外合成银纳米颗粒。这种高效、环保且简单的合成方法可用于合成 30-50 nm 的 Ag 纳米粒子。由于使用常温条件,且不含有害还原剂,我们认为这种方法是环保且成本低的。最近,拉里萨等人。 [75] 利用食线虫真菌 Duddingtonia flagrans 的无细胞滤液制备银纳米颗粒。在这项研究中,他们报告了使用食线虫真菌 D. flagrans 合成 AgNPs 的简单生物过程。与廉价、环保和高产的生物合成相比,细胞外合成不需要额外的处理来从活细胞中分离颗粒,是一个更简单的过程。生物合成和功能化的 AgNPs 具有良好的稳定性和高产率,其优异的抗菌、抗真菌、抗病毒和抗癌特性使其在治疗应用中具有广阔的前景,为利用真菌 D. flagrans 提供了新的实验设计。
可见,生物微生物的类型将是纳米银研究的最新研究方向。
AgNPs 的特性和应用
AgNPs 在抗菌方面的特性和应用
近年来,Ag纳米材料的抗菌性能逐渐引起人们的关注,并报道了大量的抗菌应用[76, 77]。 Helmlinger 等人研究了不同形状的抗菌 AgNPs。 [78]。通过对四种银纳米金属的细胞毒性和抗菌作用的研究,可以看出,不同形状的纳米银具有相同的细胞毒性,但抗菌作用不同。 Meanwhile, particles with a higher specific surface area are more toxic for bacteria than particles with smaller specific surface areas. The dissolution kinetics is correlated to the estimated specific surface area of the particles where particles with a higher specific surface area dissolve faster than particles with a smaller one. The difference in the dissolution rate may be exploited to synthesize silver nanoparticles with a relative higher antibacterial effect and a lower cytotoxic effect towards tissue. However, Helmlinger et al. did not give a further detail study on the antibacterial effect of different sizes of AgNPs.
The antibacterial properties of silver particles with different sizes were studied by Agnihotri et al. [60]. It can be seen that 5 nm nanoparticles have the best antibacterial properties. It was found that the smaller particles exhibited the better antibacterial properties. The Fig. 12 shows the antibacterial properties of the different-sized silver nanoparticles.
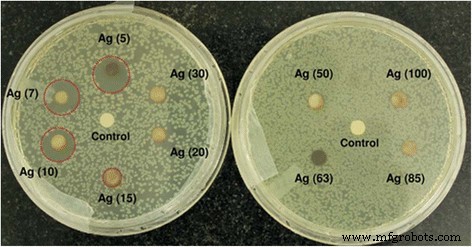
Disk diffusion tests for different-sized silver nanoparticles against the E.大肠杆菌 MTCC 443 strain. The zone of inhibition is highlighted with a dashed circle indicating a noticeable antibacterial effect [60]
图>Silver extends its antibacterial properties by combining with other materials. Research about combining with other materials included SiO2@Ag [79], PLLA microcapsules combined with silver nanoparticles [80], electrodeposited chrome/silver nanoparticles (Cr/AgNPs) [81], graphene quantum dot/silver nanoparticles [82], Ag-decorated polymeric micelles with curcumin [83] and so on.
All the above studies are about the antibacterial properties of AgNPs. Next, we introduced the silver nanoparticles for antimicrobial application. It was found that the silver nanoparticles can be directly utilized as antibacterial agents which have been also testified by Kujda et al. [84]. It is shown that silver particles attach to the bacteria surface inducing disintegration, which enables their penetration inside the bacteria. In the future, the antibacterial properties of silver nanoparticles should be applied in industry by combining with other materials. For example, Meng et al. [85] made silver nanoparticles adhered to multilayered film-coated silk fibers with the aim to get antibacterial application. The as-prepared silk could effectively kill the existing bacteria and inhibit the bacterial growth, demonstrating the antimicrobial activity. Moreover, the release of Ag + for the modified silk can last for 120 h, rendering the modified silk sustainable antimicrobial activity. This work may provide a novel method to prepare AgNPs-functionalized antimicrobial silk for potential applications in textile industry. Figure 13 shows the surface morphologies of pristine silk fiber and coated morphologies of silk. By the EDS analysis, we can make sure that nanosilver was coated with silk.
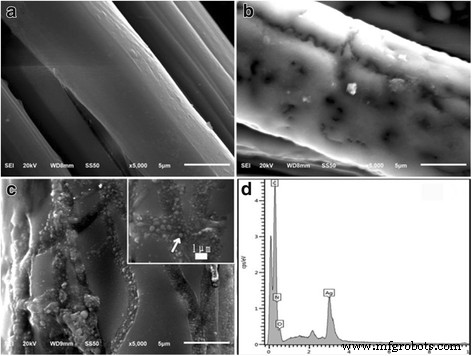
Surface morphologies of pristine silk fiber (a ), (PAA/PDDA)8 film-coated silk fiber (b ), and AgNPs-(PAA/PDDA)8 film-coated silk fiber (c )。 Inset:SEM image with higher magnification. (d ) EDS spectrum of AgNPs-(PAA/PDDA)8 film-coated silk. The arrow indicates the point randomly selected for the EDS analysis [85]
图>Other people like Zulfiqar Ali Raza et al. [86] investigated single-bath fabrication and impregnation of silver nanoparticles on enzymatic pretreated cotton fabric by using starch both as reducing as well as stabilizing agent under the autoclave conditions of 103.42 kPa, 121 °C for 15 min. The silver nanoparticles impregnated cotton fabrics showed good durable antibacterial activity against Escherichia coli and Staphylococcus aureus strains. Figure 14 shows the formation mechanism of impregnation of silver nanoparticles on cotton fabric.

Schematic diagram of impregnation of silver nanoparticles on cotton fabric [86]
图>Recently, silver nanoparticles were coated with zirconia by Yamada et al. [87] for antibacterial prosthesis. In view of the pronounced antimicrobial properties and small toxicity of AgNPs, the biocompatible AgNPs-coated yttria-stabilized zirconia can be potentially utilized to control dental caries and periodontal disease. Maybe the inspiration about wound repair will be obtained by this study. The excellent antibacterial properties of silver nanoparticles can be revealed by the above studies. Moreover, this work will help someone who wants to do further research on antibacterial.
Properties and Applications of AgNPs on Fluorescence
Because nanomaterials with fluorescent property have a great application prospect. Many efforts have been devoted to study the fluorescent property [88, 89]. Research on fluorescent nanoparticles mainly concentrates on semiconductor particles, which are usually referred to as quantum dots. Among these, CdSe particles and ZnS particles have stronger fluorescent intensity. In spite of their broaden applications, quantum dots frequently still have some problems which are related to the intrinsic blinking of their luminescence and to toxicity issues that limit their applications in the health sciences [90]. Silver is expected to have lower toxicity and can be readily prepared reproducibly and with excellent solution stability. At the same time, Ag is readily detectable in the visible spectral region [91]. Because silver has the abovementioned advantages, the preparation of highly fluorescent silver nanoparticles is needed. Highly fluorescent silver nanoparticles were prepared by Maretti et al. [92] with a facile photochemical method, which can yield these materials with excellent long-term stability in just a few minutes. The method is used photogenerated ketyl radicals which can reduce Ag + from silver trifluoroacetate in the presence of amines. The conclusion they obtained is that the luminescence arises from particle-supported small metal clusters (predominantly Ag2). Typically, silver nanoparticles show a distinct plasma band which has been between 390 and 420 nm in their past work. Due to the presence of small silver clusters, the study of the absorption band obtained was closer to 450 nm. Figure 15 shows the UV-vis absorption spectra of silver nanoparticles. Figure 16 shows the absorption (red), emission (green), and excitation (blue) spectra of Ag particles after 4 min of irradiation in tetrahydrofuran (THF) under the conditions of Fig. 15 and resuspension in toluene. From Fig. 16, we can draw the conclusion that the silver nanoparticles can emit green light. This property can be used for fluorescence diagnosis in biomedical field [93].
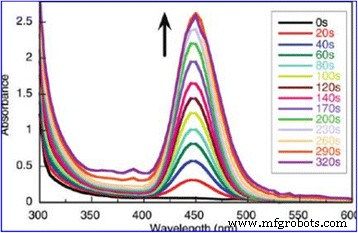
UV-vis absorption spectra following irradiation (350 nm, four lamps) of a toluene solution containing 2 mM silver trifluoroacetate, 2 mM I-2959, 2 mM cyclohexylamine. Reaction performed and monitored directly in a 0.7 × 0.3 cm quartz cuvette [92]
图>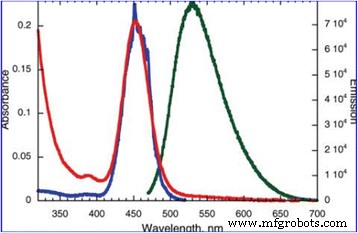
Absorption (red), emission (green), and excitation (blue) spectra of Ag particles after 4 min of irradiation in THF under the conditions of Fig. 15 and resuspension in toluene [92]
图>In order to distinguish these ultra-small particles, these nanoparticles which are smaller than 2 nm are usually called nanoclusters. In this size regime, metal nanoclusters become molecular species and size-dependent strong fluorescent emission can often be observed upon photoexcitation in the UV-visible range [94]. In particular, Ag nanoclusters, which show higher fluorescent intensity than Au nanoclusters in solutions, received considerable attention in the past few years owing to their great promise in a wide range of applications [95]. Fluorescent Ag nanoclusters were found to have wide applications in bio-imaging [96], chemical sensing [97, 98], fluorescence labeling [99], and single-molecule microscopy [100].
Properties and Applications of AgNPs on Catalysis
Since the addition of silver nanoparticles into reaction, the catalytic performance of the reaction has been significantly improved. Thus, nanocatalysis of silver nanoparticles has been a rapid growing research area which involves the use of nanoparticles as catalysts. As we all know, metals such as Ag, Au, Pt, and other metal ions can catalyze the decomposition of H2O2 to oxygen [101].郭等人。 found that when the AgNP colloid was added into the solution of luminol-H2O2, the chemiluminescence (CL) emission from the luminol–H2O2 system could be greatly enhanced. AgNPs exhibited a better catalytic performance of CL than gold and platinum nanoparticles. The AgNPs-enhanced CL was ascribed to that AgNPs could catalyze the decomposition of H2O2 to produce some reactive intermediates such as hydroxyl radical and superoxide anion. Figure 17 shows the effect of Ag colloid, Au colloid, Pt colloid, and filtrated solution of precipitated Ag colloid on luminol–H2O2 CL [102].
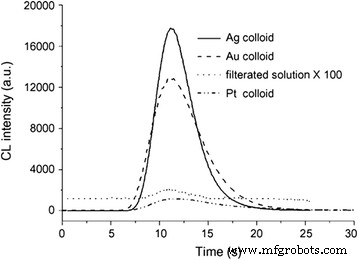
Effect of Ag colloid (solid line), 38 nm Au colloid (dashed line), Pt colloid (dash-dot-dot line), and filtrated solution of precipitated Ag colloid (dotted line) on luminol–H2O2 CL. The blank (filtrated solution of precipitated Ag colloid) signal was amplified by 100 times. Conditions:luminol, 1 × 10–4 mol/L; H2O2, 0.15 mol/L; pH 9.32 carbonate buffer for Ag, pH 12.0 NaOH for Au, pH 10.3 carbonate buffer for Pt [102]
图>Silver is the most popular catalyst when it has interaction with oxygen, water, carbon dioxide, ethylene, and methanol [103]. From the study that the catalytic properties of silver nanoparticles have accordingly changed can be realized. Jiang et al. [104] enhanced the catalytic properties of Ag by combining silver nanoparticles with silica spheres, and they also applied it to the detection of dye reduction. The technique to support silver particles on silica spheres effectively avoids flocculation of nano-sized colloidal metal particles during a catalytic process in the solution, which allows one to carry out the successful catalytic reduction of dyes. Figure 18 shows how the absorbance spectrum of the dyes decreases when the dyes are reduced.
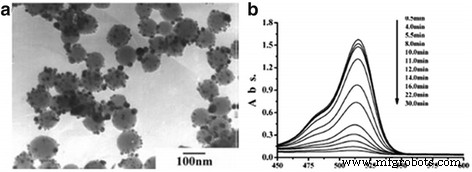
一 Silver nanoparticles immobilized on silica spheres are illustrated. b The absorbance spectrum of the dyes decreases as the dyes are reduced by sodium borohydride. This process is catalyzed by silver nanoparticles. The arrow marks the increase of reaction time [104]
图>In addition, the catalytic properties of silver also have important applications in other areas, for example, wet-spun fibers [105].
Properties and Applications of AgNPs on Surface Plasmon Resonance
In 1902, Wood found the SPR phenomenon for the first time in an optical experiment and made a brief record about that, but until in 1941, a scientist named Fano explained the phenomenon of SPR. Over the next 30 years, the theory about SPR has not been further explored nor has it been put into practical application. In 1971, Kretschmann put forward prism coupling structure that settled the foundation for the structure of SPR sensor, and SPR theory started to be widely achieved for experiments. On this basis, the surface plasma resonance effect of silver nanoparticles was explored deeply. The most successful part of the applications of plasmonic structures was in the detection of molecules. This technique has been commercialized for propagating surface plasmons (PSPs) on continuous metal films. The films are chemically functionalized to selective bind target molecules like DNA strands or proteins. Upon binding the target molecule, the dielectric environment is altered around the surface of the metal film. Consequently, binding can be monitored by measuring the change in coupling geometry (i.e., the angle) between the metal film and the excitation source needed to generate PSPs [106, 107]. This technique plays a key role, and a number of commercially available instruments are widely used today in the biological sciences [108].
Recently, the combination of silver nanoparticles with other materials to improve their surface plasmon resonance performance is another way of development. The nanosilver particles were bonded with starch by Vasileva et al. [109], and the materials were applied as a surface plasmon resonance-based sensor of hydrogen peroxide. Figure 19 shows the change of hydrogen peroxide decomposition.
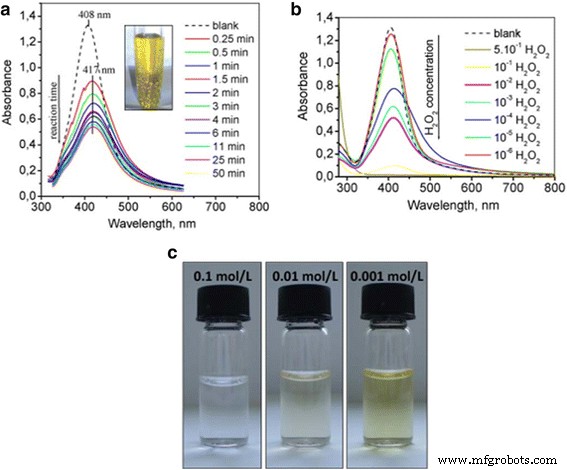
一 Change of the LSPR absorbance strength with time due to the introduction of 10–3 mol/L H2O2 solution in the as-synthesized Ag-NPs solution at a volume ratio 1:1.5; the inset shows the bubbles from H2O2 decomposition generated by the catalytic reaction between hydrogen peroxide and starch-stabilized Ag-NPs. b UV-vis absorption spectra recorded 15 min after the introduction of hydrogen peroxide solution with different concentrations in the solution of Ag-NPs at a volume ratio 1:1.5. c relevant photographs of Ag-NPs dispersions 60 min after the introduction of hydrogen peroxide with different concentrations [109]
图>SPR has a wide range of applications in other fields such as life science, medical testing, drug screening, food testing, environmental monitoring, and forensic identification.
The SPR technology becomes an indispensable part in the field of biological chemistry, food, and drug monitoring. The applications of SPR biosensors will be more diversified. And especially its emerging application in small molecule detection and lipid field will make it play an increasingly important role in the film and biology. In recent years, its development is particularly rapid. With the continuous improvement of SPR instruments and the continuous enhancement of biological membrane construction capability, SPR biosensor has a bright future.
Applications of AgNPs on Nanosensors
Due to the great research prospect of silver nanoparticles in nanosensors, many researchers have devoted to study it [110, 111]. So, we pick three representative examples to write in detail. Among them Zhu et al. [110] fabricated rhombic silver nanoparticles for biosensing. The rhombic silver nanoparticles were prepared by follow method. The mixed solution (polystyrene nanospheres and glass nanospheres with fluorocarbon surfactant) was coated onto the glass substrate to form a deposition mask, and then followed by hydrofluoric acid etching to remove the glass nanospheres. After that, the Ag metal thin film was deposited through the nanosphere masks using thermal evaporation or electron beam evaporation. After removal of the polystyrene nanospheres by sonication in absolute ethanol for 3 min, well-ordered rhombic AgNPs array was finally obtained on the substrates. The rhombic AgNPs array was single particle dimension of 140 nm in-plane width and 47 nm out-of-plane height. To prepare the biosensing, the Ag nanorhombuses are firstly functionalized using the self-assembly monolayer technique. Then assisting with 1-ethyl-3-[3-dimethylaminopropyl] carbodiimide hydrochloride, we covalently attached biotin to the carboxylate groups. The advantage of this biosensor is that the rhombic AgNPs array-based sensor with more hot spots has higher sensitivity than that of the traditional Ag triangular nanoparticles-based sensor. A detection of high sensitivity of the bio-molecule in lower concentration has been realized by means of the LSPR-based nanobiosensor. This type of biosensor will have potential applications in many fields such as medical science and biological technology. Meanwhile, M. Ghiaci et al. [111] utilized silver nanoparticles compounds as new electrochemical sensors for glucose detection. These electrochemical sensors were prepared based on synthesizing of two amine compounds bounded to silica support. The size of used AgNPs is 10 nm. The electrochemical sensor prepared by this method has a lower limit of glucose detection than other electrochemical sensors. This type of nanosensors will be more conducive to diabetes detection and treatment. Silver nanoscale sensors can also be used for environmental detection such as Li et al. [112] synthesized aza-crown ether (ACE)-modified silver nanoparticles as colorimetric sensors for Ba 2+ . What is more, colorimetric sensors merely need minimal instrumentation, achieve high sensitivity, and thus can make on-site detection even easier. The colorimetric sensors were synthesized by silver nanoparticles efficiently conjugated with CS2–ACE. ACE-modified AgNPs have good recognition of Ba 2+ , with the detection limit of 10 − 8 mol/L.
In addition to the abovementioned, silver nanosensors also have other different applications that are worth us to explore.
Other Applications
Ag nanomaterials also have many other applications in various fields, such as nanoscale detection [113] and solar cells.
Silver nanoparticle and its complex can be used for solar cells to enhance photoelectric conversion efficiency and photovoltaic performances [114,115,116].
Shen et al. [114] enhanced photovoltaic performances of polymer solar cells by incorporating Ag–SiO2 core–shell nanoparticles in the active layer. They creatively incorporated Ag–SiO2 core–shell nanoparticles (Ag–SiO2-NPs) into photo−/electro-active layers consisting of poly(3-hexylthiophene) (P3HT) and phenyl-C61-butyric acid methyl ester (PCBM) in polymer solar cells (PSCs). By this way, the photovoltaic performance of PSCs have largely been enhanced. The results demonstrate a 13.50% enhancement of short-circuit photocurrent density and a 15.11% enhancement of power conversion efficiency as the weight percent of doped Ag–SiO2-NPs is 1.5 wt% in the active layer of corresponding PSCs. In the later research, bare silver nanoplate (Ag-nPl) were spin-coated on indium tin oxide and silica capsulated Ag-NPs were incorporated to a PBDTTT-C-T:PC71BM active layer by Shen et al. [115]. As a result, the devices incorporated with Ag-nPl and Ag@SiO2-NPs showed great enhancements. With the dual effects of Ag-nPl and Ag@SiO2-NPs in devices, all wavelength sensitization in the visible range was realized; therefore, the power conversion efficiency of PSCs showed a great enhancement of 14.0 to 8.46%, with an increased short-circuit current density of 17.23 mA cm − 2 . Importantly, the methodology of multiple shape combination of metallic nanoadditives improves the photovoltaic performance of PSCs very effectively compared to the single-shape method.
Thus, Ag is a promising material for the conversion of solar energy into electricity and good detection. In addition to the abovementioned, Ag also has many other applications, but it still needs people to further explore it.
Conclusions
This work reviewed the development progress of Ag nanomaterials on synthesis methods and applications. Different shapes of Ag nanostructures had been synthesized such as cubic, rod-shaped, and sphere-shaped, Ag nanostructure obtained by chemical synthesis and microwave methods were successfully prepared. In addition, different size of AgNPs have been synthesized such as 1–10 nm, 10–100 nm, AgNPs obtained by chemical synthesis, laser ablation, and green synthesis. Meanwhile, it has been successfully applied to many fields, such as antibacterial, fluorescence, catalysis, SPR, and nanosensors, and it is expected to use in other fields. In fact, there are still limitations for their practical applications in photoelectric and medical fields because it often requires complex preparation process, and the yield is very low. In most cases, AgNPs are easy to agglomerate, which will greatly reduce its optical properties. Therefore, it is necessary to utilize surface active agent to achieve a good effect. Although, there are so many challenges, the advances in nanoscience and nanotechnology of silver still promise a better future for many kinds of industries. In conclusion, the future research of silver nanoparticles should be directed towards biosynthetic, size controllable, and uniform shape preparation. And the future application of AgNPs-based will be utilized in new energy battery or wearable intelligent equipment by its excellent localized surface plasmon resonance effect and antibacterial activity. In addition, AgNPs-based materials can be further utilized for applications in nanodevices by self-assembly and molecular molding technology.
缩写
- ACE:
-
Aza-crown ether
- Ag-nPl:
-
Silver nanoplate
- AgNPs:
-
Ag nanoparticles
- CL:
-
Chemiluminescence
- CTAB:
-
Cetyltrimethyl ammonium bromide
- DBSA:
-
Dodecyl benzene sulfonic acid
- DLS:
-
动态光散射
- EDS:
-
Energy dispersive spectroscopy
- fcc:
-
Face-centered cubic
- HRTEM:
-
高分辨透射电子显微镜
- LSPR:
-
局域表面等离子体共振
- MTCC:
-
The name of bacteria
- MTPs:
-
Multiply twinned particles
- P3HT:
-
聚(3-己基噻吩)
- PAA:
-
Poly(acrylic) acid
- PBDTTT-C-T:
-
Poly[4,8-bis((2-ethylhexyl)thiophen-5-yl)-benzo[1,2-b:4,5-b’]dithiophene-2,6-diyl]-alt-[2-(20-ethylhexanoyl)-thieno[3,4-b]thiophene-4,6-diyl]}
- PC71BM:
-
Fullerene derivatives acceptor material C71-butyric acid methyl ester
- PCBM:
-
Phenyl-C61-butyric acid methyl ester
- PDDA:
-
Poly(dimethyldiallylammonium chloride)
- PEG:
-
Polyethylene glycol
- PLLA:
-
Poly(L-lactide)
- PSCs:
-
Polymer solar cells
- PSPs:
-
Propagating surface plasmons
- PVA :
-
Poly-vinyl alcohol
- PVP:
-
Poly-vinyl pyrrolidone
- SDA:
-
Sabro dextrose agar
- SDS:
-
Sodium dodecyl sulfate
- SEM:
-
扫描电子显微镜
- SERS:
-
表面增强拉曼散射
- SPR:
-
Surface plasmon resonance
- TEM:
-
透射电子显微镜
- THF:
-
四氢呋喃
- UV-vis:
-
Ultraviolet-visible
- XRD:
-
X射线衍射
纳米材料