用于改进诊断和治疗应用的多功能金纳米粒子:综述
摘要
金属的医学特性在用于治疗感染和疾病的传统医学中已经探索了几个世纪,并且至今仍在实践中。铂类药物是继 40 多年前美国食品和药物管理局 (FDA) 批准顺铂后,第一类临床用作抗癌药物的金属类药物。从那时起,更多具有健康益处的金属被批准用于临床试验。有趣的是,当这些金属被还原为金属纳米粒子时,它们显示出优于大块金属的独特而新颖的特性。金纳米粒子 (AuNPs) 是 FDA 批准的金属纳米粒子之一,并在医学中的各种作用中显示出巨大的前景。它们被用作药物递送、光热 (PT)、造影剂、治疗剂、放射增敏剂和基因转染剂。本文综述了它们的生物医学应用,涵盖了它们在疾病诊断和治疗中的潜在用途。还讨论了一些被批准用于临床试验的基于 AuNP 的系统,以及 AuNP 的潜在健康威胁和一些可用于提高其生物相容性的策略。所审查的研究提供了原理证明,即基于 AuNP 的系统有可能单独使用或与传统系统结合使用以提高其功效。
介绍
医学是受益于纳米技术的众多领域之一。纳米技术的出现为通过使用纳米材料改进和开发新型诊断和治疗药物带来了很多机会 [1, 2]。特别是 AuNPs 表现出独特的物理化学性质和良好的化学稳定性。它们很容易被几乎所有类型的给电子分子官能化,通过各种化学反应或基于它们对硫醇化分子的强亲和力 [3, 4]。由于其体积小,AuNPs 具有更大的表面积和高载药量。可以在 AuNPs 中加入多个部分用于生物医学应用;其中包括用于提高特异性的靶向分子、用于生物成像和实时监测疾病对药物反应的造影剂,以及用于疾病治疗的治疗剂 [5, 6]。有趣的是,即使没有添加任何生物分子,AuNPs 也能够靶向、成像和治疗疾病。基于它们的尺寸相关特性,可以创建新的基于 AuNP 的系统,用于各种生物医学应用 [7]。
AuNP 由热稳定的金属前体制成,因此非常稳定且不可生物降解。大块黄金用于医药,已被证明具有生物惰性和无毒 [8, 9];因此,AuNP 中的金核将基本上显示出相似的特性 [3, 10]。 AuNPs 及其应用已被广泛研究了 50 多年,并在临床前 [5, 11,12,13] 和临床研究 [14,15,16,17,18] 中显示出作为治疗诊断剂的巨大前景。正如本综述中所讨论的,存在更多基于 AuNP 的新型系统的机会。 AuNPs 已经在临床试验中被探索作为治疗晚期癌症的药物载体 [16, 17],以及作为治疗前列腺癌 [19] 和痤疮 [18] 的 PT 药物。在不破坏围绕使用 AuNP [20] 的健康和监管问题的情况下,这些系统在生物医学中的未来就在眼前。基于 AuNP 的多功能系统能够以局部和改进的功效对抗耐药性 [11, 21, 22]。该评论通过反思 AuNP 作为诊断和治疗剂的生物应用,突出了临床前和临床研究中 AuNP 的生物学特性。还描述了它们潜在的健康威胁和用于克服其局限性的策略。最后,强调了金纳米粒子在医学上的未来前景。
金纳米粒子
由于其独特的化学和物理特性,AuNPs 在医疗应用中的普及获得了很大的推动力。 AuNPs 是固体胶体颗粒,尺寸范围从 1 到 100 nm [23]。 AuNPs 在生物学中的应用植根于它们的物理化学特性,而不仅限于它们的大小、表面等离子体共振 (SPR)、形状和表面化学 [3, 10]。这些参数影响它们的活性并使它们成为用于疾病诊断和治疗的完美候选者,无论是作为传递、敏化、对比还是治疗剂。它们的小尺寸与更大的表面积相关,这允许表面修饰和多种有效载荷的附着,例如靶向、成像和治疗剂 [4, 24,25,26]。它们的小尺寸也使纳米颗粒及其货物能够穿过难以到达和穿透的生物屏障[11]。
AuNP 越来越被认为是可行的诊断、治疗和治疗诊断(一种可以同时用于诊断和治疗疾病的药剂)药剂,它有可能解决与传统疗法相关的脱靶效应。然而,AuNPs 与它们的生物相容性大块对应物相比具有不同的特性和功能,这可能对人类健康造成危害 [27,28,29]。临床上使用大块金化合物治疗疾病是一种古老的做法,并被证明是安全的 [8]。近年来,研究表明 AuNPs 具有相似或改进的医学特性 [29]。由于其独特的光学、化学和物理性质,AuNPs 与大块金相比通常具有新的性质 [30, 31],可作为诊断和治疗剂 [5]。
AuNPs 的合成
AuNP 可以按照自上而下或自下而上的方法以多种方式生产。自上而下的方法使用物理和化学方法从大块材料中生产所需尺寸,而自下而上的方法涉及化学方法来组装纳米尺寸系统中的构建块 [32, 33]。物理方法(如研磨、光化学、辐射和光刻)使用大量的能量和压力将大块材料按比例缩小到 10 –9 十亿分之一米 [10, 32, 34]。使用物理方法时,成核过程很容易控制,不需要还原剂,并且在其中一些方法中,合成与 NPs 的灭菌同时发生。然而,物理技术通常成本高昂、不易获得并且需要专门的设备。此外,封端剂和稳定剂可能无法在这些过程中涉及的高能过程中存活下来[34]。
自下而上的方法在 AuNPs 的合成中最受青睐,因为它快速、简单且不需要使用复杂的设备 [33,34,35]。它基于 Turkevich 于 1951 年开发的化学方法(图 1A),该方法使用柠檬酸盐还原和稳定金前驱体,从而产生 15 纳米球形 AuNP [3, 10, 23, 33, 36 , 37]。该方法通过改变柠檬酸盐与金前体含量的比例进一步改进,导致 AuNP 的尺寸直径范围为 15-150 nm(图 1B)[10, 24]。还引入了许多还原剂,如硼氢化钠、十六烷基三甲基溴化铵 (CTAB) 和抗坏血酸。不幸的是,一些化学还原剂具有毒性 [33, 34, 36],通常通过在其表面添加稳定剂(例如聚乙二醇 (PEG)、阿拉伯树胶、多糖和生物活性肽)来钝化 [37, 38]。
<图片>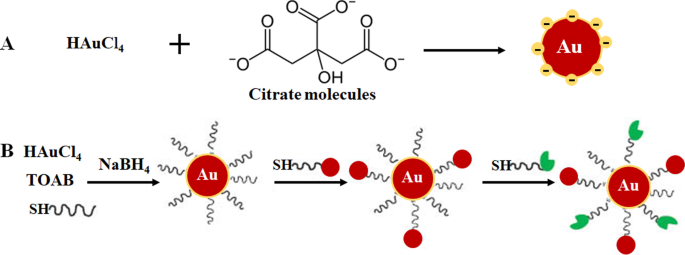
通过柠檬酸还原(A ) 和两相系统还原,然后通过配体交换反应稳定和功能化,Brust-Schiffrin 方法 (B )。经许可转载[36]。版权所有 2013,德格鲁伊特。 TOAB 四丁基溴化铵,SH 硫醇化分子
图>已经在合成 AuNP 中探索了更环保的方法,例如微波诱导液体中等离子体 (MWPLP) 和绿色纳米技术,以避免使用有毒化学还原剂。 MWPLP 使用微波产生金属纳米粒子的成核,不需要任何还原剂,合成所需的能量非常低 [34]。另一方面,绿色纳米技术使用源自植物和微生物的天然化合物作为合成生物金纳米粒子的还原剂来源 [12, 33, 39,40,41]。绿色纳米技术被认为是生态和环境友好的,因此更适合生物医学应用。植物介导的合成比使用微生物更经济。而且,合成可以一步完成,NPs更容易纯化。此外,植物是可再生的;植物的各个部分,如叶、茎、树皮、根、花和果实,可以在不杀死植物的情况下收获并用于合成。从植物材料中制备的提取物含有植物化学物质、蛋白质和酶,可作为还原剂、稳定剂和封端剂 [10, 12, 24, 34, 35, 40, 42]。绿茶中的表没食子儿茶素 [42] 和芒果中的芒果苷 (MGF) [12, 43] 是已广泛用于合成 AuNPs 的植物衍生化合物 [34]。有关这些方法的更多信息在以下参考文献 [10, 24, 34, 35] 中进行了广泛的回顾。
金纳米粒子的生物应用
AuNPs 在医学科学中的作用和重要性无疑变得越来越明显,这得益于越来越多的研究证明其在广泛的生物医学领域的多方面应用。 AuNPs 的生物相容性归功于黄金在治疗人类疾病方面的悠久历史,可以追溯到公元前 2500-2600 年。中国和印度人用黄金治疗阳痿、癫痫、梅毒、风湿病和肺结核。中国发现了红胶体金的延年益寿作用,印度仍将其作为阿育吠陀医学的一部分用于恢复活力和振兴。朱砂金(也称为 Makaradhwaja)在印度用于提高生育能力。在西方国家,黄金已被用于治疗神经紊乱和癫痫症。在体外和体内研究中均未报告其使用毒性 [8, 44, 45]。从那时起,口服和注射金化合物继续被用作关节炎的治疗方法 [9, 46],并且还被证明具有抗癌作用 [8]。 AuNPs 也有类似的报道,在某些情况下也有改善的效果,它们正在成为疾病诊断 [47,48,49] 和治疗 [3, 29, 50, 51] 的有前景的药物。
AuNPs 具有更大的表面积,可用于生物医学应用,通过附着各种生物分子来适应所需的功能。这些可以包括靶向部分以帮助识别疾病特异性生物标志物、用于生物成像的造影剂和用于治疗疾病的治疗剂 [24, 25]。与其他纳米材料相比,使用 AuNP 的优势在于它们可以使用各种化学物质轻松实现功能化,如图 2 [4, 26] 所示。 AuNPs 对硫醇化分子具有高亲和力,硫醇-金结合是将分子吸附到 NP 表面的最常用方法 [4]。还使用基于亲和性的化学反应,例如生物素-链霉亲和素结合和碳二亚胺偶联。 AuNPs 用于生物医学的三个主要领域:药物递送、诊断和治疗目的 [24, 35],并在这些领域显示出巨大的潜力,如下所述。
<图片>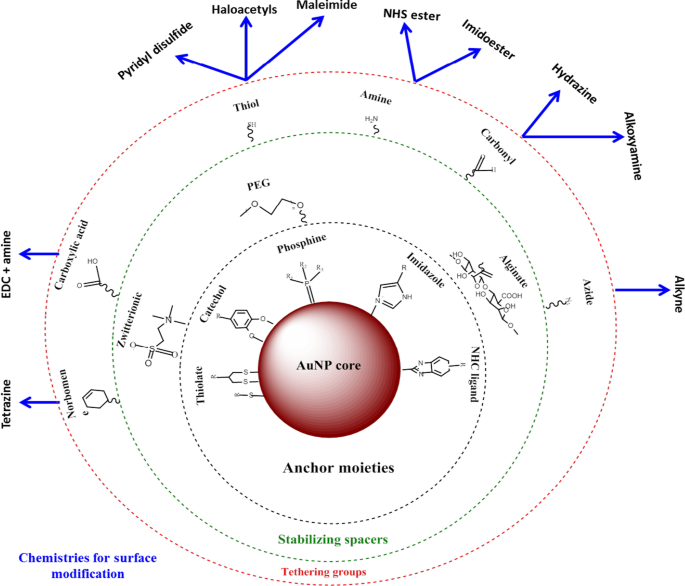
AuNPs的合成和功能化。具有官能团的生物分子首先通过金硫醇亲和力吸附在 NP 表面。然后,其他官能团(例如胺基团)可用于结合带有羧基的分子以连接靶向或药物部分。改编自 [32]
图>AuNPs 作为药物递送剂
AuNPs 最常见的应用是作为药物 [11, 18, 52]、疫苗 [53] 和基因治疗 [24, 32] 的载体。 AuNPs 具有的特性可以解决与传统疗法相关的大多数问题,例如耐药性、药物分布低、生物降解和早期药物清除 [11]。 AuNPs 可以显着减少药物剂量、治疗频率并能够运输疏水性和不溶性药物。它们被认为是生物惰性的,可以掩盖其货物免受免疫细胞的攻击,保护药物在通过循环系统时免受蛋白水解降解,从而增加药物循环时间。这些因素可以通过将药物集中并保留在患病组织中而对正常组织影响很小或没有影响而很容易地提高药物的疗效[25]。
金纳米粒子在癌症治疗中的应用已得到广泛研究 [17, 37, 54],多年来,它已扩展到其他疾病,如肥胖 [50, 55, 56] 和痤疮 [18]。基于纳米的系统比大多数细胞成分小,并且可以通过利用对患病组织脉管系统的增强渗透性和保留 (EPR) 效应被动地穿过细胞屏障 [25]。病理状态下的 EPR 的特点是血管生成过多,通透性介质分泌增加,可以增强病变组织对 AuNP 的吸收。这些特征仅与病理状态相关,与正常组织无关,这为选择性靶向 AuNP 偶联物提供了机会 [25]。 AuNPs 作为药物载体很有吸引力,因为它们可以同时携带多个分子,进一步使其特性多样化。这是大多数 AuNP 生物应用所依赖的医学中的理想特性,因为 AuNP 可以针对特定的生物医学功能进行定制。这有助于控制它们与细胞器相互作用的方式,因此为未来开发各种疾病的有效诊断和治疗方式提供了希望 [4]。
基于 AuNP 的诊断系统
与传统诊断测试相比,纳米技术的出现提高了开发快速、稳健、灵敏且具有高度竞争力的检测系统的立场[48]。纳米材料通常集成在现有的生物传感平台中,用于检测与疾病发展有关的气体、DNA 和蛋白质标记物 [47]。在用于诊断的各种纳米材料(包括金属、聚合物、磁性和半导体纳米粒子)中,金纳米粒子已广泛用于生物传感器、电化学传感器和显色分析,以检测或感知疾病生物标志物的存在 [49]。它们的定域 SPR (LSPR)、荧光共振能量转移 (FRET)、表面增强拉曼散射、电导率、氧化还原活性和量化充电效应使它们成为目标分子成像和检测的理想工具 [10, 24]。它们的电子和光学特性以及散射可见光和近红外 (NIR) 光的能力与各种技术兼容并可以测量,例如显微技术(电子、共焦和暗场光散射)[57]、计算机断层扫描 (CT) , PT外差成像技术,UV-Vis和拉曼光谱[24, 35]。
基于 AuNP 的诊断系统的开发涉及 AuNP 表面的修饰,例如,通过连接识别疾病生物标志物的生物分子 [3, 24, 58]。侧向流分析 (LFA) 可能是基于纳米技术的诊断工具最著名的例子。 LFA 通常使用约 30-40 nm 的 AuNP,因为较小的颗粒具有非常小的消光截面,而较大的颗粒通常在这些测定中使用时不稳定 [59]。此外,还包括可以触发 AuNPs 的 SPR、电导率和氧化还原变化的其他分子/酶。这些指示剂在分析物与 AuNP 偶联物结合后给出可检测的信号 [24],信号的缺乏或存在将反映目标分子或疾病的存在或不存在。当用于不同的测试形式时,AuNPs 产生的信号具有化学稳定性、持久性和一致性:试管、试纸条、体外和体内 [24]。因此,它们的应用显着提高了诊断检测的速度和成功率。
基于 AuNP 的比色分析
在比色分析中,AuNP 会产生视觉信号(通常是颜色变化),无需使用高级仪器即可用肉眼检测到。通常,AuNPs 的胶体溶液呈红宝石色至葡萄色,高度依赖于颗粒间距离 [60, 61]。分析物与用分子生物识别元素(例如,抗体、肽、适体、酶等)修饰的 AuNP 结合会导致 LSPR 发生明显变化,从而导致颜色从红宝石色变为蓝色 [60 , 62, 63]。颜色的强度与分析物的浓度成正比,用于确认疾病的存在和状态。基于 AuNP 的比色诊断已成功用于检测甲型流感病毒 [64]、寨卡病毒 [65]、T7 噬菌体 [66]、结核分枝杆菌 [67] 以及最近用于检测严重急性呼吸系统综合症冠状病毒-2 (SARS-CoV-2) [60, 68]。
展示了基于比色 AuNP 的测定方法的一个例子,用于检测 SARS-CoV-2 [60],一种导致 2019 年高传染性冠状病毒病 (COVID-19) [60, 68] 的病毒。通过这种分析,病毒的存在是通过简单的颜色变化来报告的;不需要任何仪器来进行诊断。目前对该病毒的临床诊断测试要么使用逆转录酶实时聚合酶链反应 (RT-PCR) 检测,该检测需要 4-6 小时,而快速即时 (PoC) 系统检测的抗体可能需要几天就出现在血液中。相比之下,如图 3 所示,基于比色 AuNP 的检测更可靠、更快速。在 SARS-CoV-2 RNA 样本存在的情况下孵育用反义寡核苷酸 (ASO) 标记的 AuNP 导致在细胞内形成蓝色沉淀。 ~ 10 分钟。在 SARS-CoV-2 阳性测试中,ASO 与病毒核衣壳磷蛋白中的 N 基因结合导致肉眼检测到的蓝色。该测试非常灵敏,SARS-CoV-2 RNA 的检测限为 0.18 ng/μL [60]。
<图片>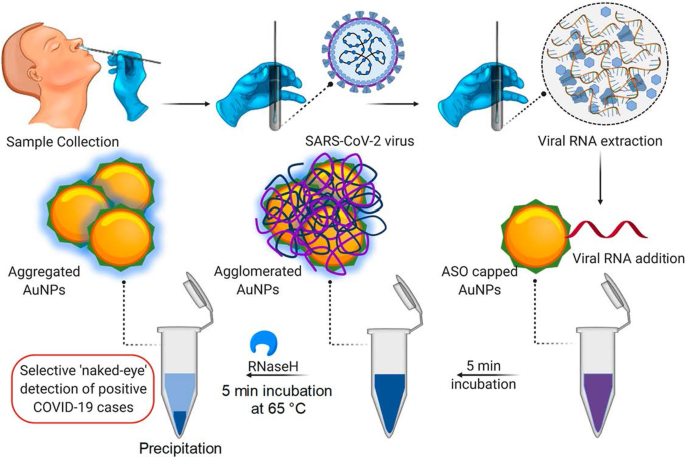
基于 AuNP 的比色诊断系统。通过 ASO 加帽的 AuNPs 选择性肉眼检测 SARS-CoV-2 RNA。经许可转载[60]。版权所有 2020, ACS Nano
图>基于 AuNP 的 LFA 遵循与图 3 所示相同的原理;然而,当存在分析物时,测试条上会形成一条可见线,而不是溶液中的颜色变化。在分析物存在的情况下,AuNPs 被捕获在测试线上并形成一条明显的红线,肉眼可见。线的强度由吸附的 AuNP 的数量决定 [69]。图 4 显示了一个简单快速的基于 AuNP 的 LFA 的示例,用于检测 Pneumocystis jirovecii (P. jirovecii ) 人血清中的 IgM 抗体。 40 nm AuNPs 与 P 的重组合成抗原 (RSA) 结合。吉罗维西 ,主要表面糖蛋白或 kexin 样丝氨酸蛋白酶,用作指示是否存在 P。吉罗维西 .在阳性测试中,P.吉罗维西 IgM 被 AuNP-RSA 偶联物在偶联物垫上捕获。 AuNP-RSA/IgM 复合物然后流向分析膜,在那里它结合抗人 IgM(测试线),多余的部分移动到抗 RSA 抗体(对照线),产生两条红线。阴性测试在控制线上将只有红色 [70]。一项独立研究使用基于 AuNP 的 LFA 选择性检测 SARS-CoV-2 IgM,这通过测试线和对照线中红线的出现得到证实 [68]。两种系统均在 15 分钟内通过肉眼目测颜色,每次测试仅需要 10-20 μL 血清样品 [68, 70]。
<图片>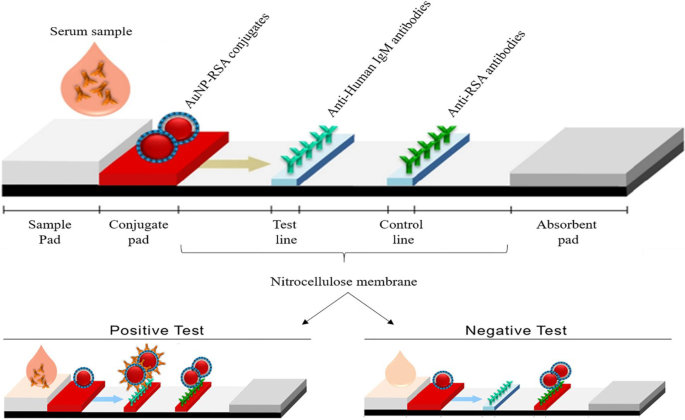
基于 AuNP 的 LFA 用于检测 IgM P。吉罗维西 抗体。 P 的存在(阳性测试)或不存在(阴性对照)。吉罗维西 抗体可以通过 AuNP 微红色在测试线和对照线中进行区分,或者仅在对照线中进行区分。经许可转载[70]。版权所有 2019,微生物学前沿
图>使用 AuNP 作为 LFA 上的信号探针的第一个例子是用于检测拉莫斯细胞; TE02 适体用作捕获探针,TD05 适体用作检测探针。适配体-AuNP 生物传感器可以在 15 分钟内在没有任何仪器的情况下视觉检测至少 4 000 个 Ramos 细胞和使用便携式条形阅读器的 800 个 Ramos 细胞。使用这种夹心检测生物传感器,该测定成功地检测到人血中掺入的拉莫斯细胞 [71],并被用作开发快速、灵敏和低成本的循环癌细胞定性和定量检测系统的概念证明。从那时起,各种基于 AuNP 的 LFA 被设计用于诊断多种传染病,包括由肺孢子菌肺炎引起的疾病 [70]、埃博拉病毒 [72]、HIV、丙型肝炎病毒和结核分枝杆菌 [73] 以及最近的 SARS-CoV-2 病毒 [68]。
基于 AuNP 的成像系统
由于 AuNPs 能够吸收和散射与其共振波长相匹配的光,高达 10 5 比传统荧光团多倍 [74]。 AuNP 具有更高的原子序数和电子密度(79 和 19.32 g/cm 3 ) 与传统的碘基试剂(53 和 4.9 g/cm 3 ),从而证明是更好的造影剂 [24]。 AuNPs 聚集在患病细胞或组织上,并引起强烈的 X 射线衰减,使目标部位高度清晰且易于检测。 AuNPs 附着在化学部分和分子生物识别剂上,可以选择性地靶向特定抗原以诱导 CT 成像的不同和目标特异性对比度 [75]。
体外靶向分子 CT 成像系统是通过使用与前列腺特异性膜抗原 (PSMA) 结合的 RNA 适配体功能化的 AuNPs 实现的。与缺乏靶受体的 PC-3 前列腺细胞相比,AuNP-PSMA 适体偶联物显示出表达 PSMA 的前列腺 (LNCaP) 细胞的 CT 强度超过四倍 [76]。类似地,AuNP-泛影酸-AS1411 适配体偶联物定位于 CL1-5(人肺腺癌)细胞和 CL1-5 荷瘤小鼠。 AS1411 适配体靶向细胞表面 CL1-5 细胞表达的核仁蛋白 (NCL) 受体,而泛影酸是一种碘基造影剂。 AuNP-泛影酸-AS1411适体偶联物具有线性衰减曲线,斜率为0.027 mM Au Hounsfield单位(HU -1 ) 表明 AuNPs 在肿瘤部位的积累 [77]。 AuNPs表现出更长的血管停留时间,延长了它们在血液中的循环时间[77,78,79]并改善了泛影酸的CT信号[77]。
图 5 显示了冠状动脉的体内 CT 血管成像,使用 AuNP 与胶原结合粘附蛋白 35 (CNA35) 偶联,用于在啮齿动物的心肌梗塞中靶向胶原 I。静脉内 (i.v) 给药 6 小时后,血液中仍能检测到 AuNP 信号,这显着高于碘基药物的半衰期(5-10 分钟)[79]。这些效果通过使用绿色合成的甘露聚糖封端的 AuNP 来复制,在甘露糖表达(DC 2.4 和 RAW 264.7)细胞中显示出受体介导的吸收和无毒性。注射到小鼠后腿后,甘露聚糖封端的 AuNPs 选择性地靶向体内腘淋巴结 [38]。基于 AuNP 的 CT 成像可以为各种疾病的诊断提供重要信息,不仅限于冠状动脉和癌症 [76, 77, 79,80,81]。 AuNPs 作为造影剂的使用已在其他成像系统中显示出潜力,如光声、核成像、超声和磁共振成像。这些系统在别处进行了广泛的审查 [82, 83]。
<图片>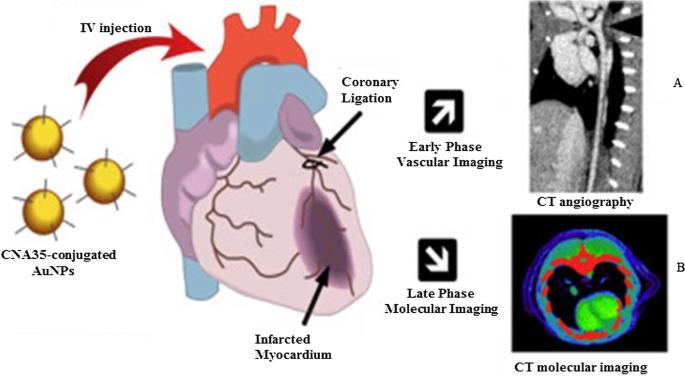
使用 AuNPs 作为 CT 造影剂的体内 CT 成像。甘露聚糖覆盖的 AuNPs 及其淋巴结 CT 成像 (A ) 和 CNA35 共轭 AuNPs 心肌瘢痕负荷的 CT 成像 (B )。经许可转载[79]。版权所有 2018,爱思唯尔
图>基于荧光的检测系统中的 AuNPs
AuNP 在基于荧光的检测系统中用作荧光剂或荧光猝灭剂。在尺寸 ≤ 5 nm 时,AuNP 显示出量子点 (QD) 的特性,并且可以代替它们使用。 1981 年推出的 Au55(PPh3)12Cl6 纳米团簇由于其量子尺寸行为可能是研究最深入的 [7]。从那时起,各种量子尺寸的 AuNPs (AuNPsQ),如 Au25(SR)18、Au38(SR)24 和 Au144(SR)60 [84] 主要用于电化学传感,因为它们是优良的电子导体和氧化还原介质。 85]。
AuNPsQ 薄膜电极用于制造用于检测前列腺特异性抗原 (PSA) 的超灵敏电化学免疫传感器。该免疫传感器的灵敏度为 31.5 μA mL/ng,对于 10 μL 未稀释人血清中的 PSA 的检测限为 0.5 pg/mL。在生物标志物浓度低于与癌症存在相关的水平的情况下,免疫测定比先前报道的包含多个部分的碳纳米管森林免疫传感器的性能好八倍。因此,它可用于测量正常和患病状态下的测试生物标志物。免疫传感器的性能与参考 ELISA 方法相当 [86]。 AuNPsQ 也被掺入多孔结构的 CaCO3 球体中,形成荧光 CaCO3/AuNPsQ 混合物,用于检测神经元特异性烯醇化酶,这是创伤性脑损伤和肺癌的诊断和预后生物标志物。传感器的检测限为 2.0 pg mL -1 [87]。迄今为止,已有几种基于 AuNP 的荧光检测系统被报道用于检测与乙型肝炎 [73, 88]、甲型流感 [89]、癌症 [90] 和心脏损伤 [91] 相关的分析物。
AuNP 也是出色的基于 FRET 的猝灭剂 [92]。它们独特的光学特性(稳定的信号强度和抗光漂白性)、尺寸和修改能力使它们成为荧光传感平台中有吸引力的探针 [93, 94]。较大的 AuNP(≥ 10–100 nm)具有低量子产率,不适合直接荧光传感;然而,它们在相对高激发能状态下猝灭荧光染料的能力使它们成为有效的光致发光猝灭剂 [94]。原则上,荧光纳米探针由供体荧光团(染料或量子点)和受体 AuNPs 组成,当靠近时,所选荧光团的荧光被 AuNPs 淬灭 [94, 95]。在缺乏荧光信号所指示的靶标不存在的情况下,核酸探针杂交并形成环状结构,使荧光团和其相对末端的猝灭剂紧密接近;而分析物与核酸探针的结合会取代 AuNP 中的荧光团,从而产生荧光信号 [24, 94, 96]。利用上述特性,AuNPs 被掺入分子信标中,用于体外(金纳米球,AuNSs)和体内(金纳米棒,AuNRs)检测肿瘤细胞上的基质酶表达。这两个分子信标由作为 AuNP 和荧光团之间的接头的 Matriptase 切割位点组成。 The AuNS–molecular beacon was constructed with the fluorescein isothiocyanate (FITC), and the AuNR–molecular beacon had a NIR fluorescent dye (mercaptopropionic acid, MPA). In the absence of the target, the AuNSs and AuNRs, respectively, blocked the FITC and MPA fluorescence. Cleavage of either FITC or MPA from the AuNP–molecular beacons in the presence of matriptase exhibited a quantifiable fluorescence signal. The fluorescent signal of the MPA–AuNR–beacon in the nude mice bearing HT-29 tumors lasted for 14 h in the tumor site, while the signal gradually disappeared from the non-tumor site over time [97].
The AuNPs were reported to have comparable or higher fluorescence quenching efficiency than organic quenchers such as 4-((4′-(dimethyl-amino)phenyl)azo)benzoic acid (DABCYL) [94, 98] and Black Hole Quencher-2 [99]. The fluorescence quenching efficiency of 1.4 nm AuNPs was compatible with the four commonly used organic fluorophores (FITC, rhodamine, texas red and Cy5). The fluorescence quenching efficiency of the AuNPs was similar to that of DABCYL, and unlike DABCYL, the AuNPs showed consistency in both low and high salt buffers [98]. In a competitive hybridization assay, 10 nm AuNPs showed superior (> 80%) fluorescence quenching efficiency for Cy3 dye than the commercial Black Hole Quencher-2 (~ 50%). The assay had a limit of detection of 3.8 pM and a detection range coverage from 3.8 pM to 10 nM for miRNA-205 in human serum, and it was able to discriminate between miRNAs with variations in their nucleotide sequence [99]. The competitive sensor arrays were not only sensitive [96, 99] but were able to differentiate between normal and diseased cells, as well as benign and metastatic cancers [96].
AuNP-Based Bio-barcoding Assay
AuNP-based bio-barcoding assay (BCA) technology has become one of the highly specific and ultrasensitive methods for detection of target proteins and nucleic acids up to 5 orders of magnitude than the conventional assays [100]. The assay relies on magnetic microparticle probes, which are functionalized with antibodies that bind to a specific target, and AuNP probes encoded with DNA that recognizes the specific protein target and antibodies. Upon interaction with the target DNA, a sandwich complex between the magnetic microparticle and AuNPs probes is formed. The sandwich is then separated by the magnet followed by thermal dehybridization to release the free bar-code DNA, enabling detection and quantification of the target [101, 102].
The AuNP-based BCA assay was able to detect HIV-1 p24 antigen at levels that was 100–150-fold higher than the conventional ELISA [103]. The detection limit of PSA using these systems was 330 fg/mL [104]. The versatility of AuNPs for the development of a BCA-based platform was further demonstrated by measuring the concentration of amyloid-beta-derived diffusible ligands (ADDLs), a potential Alzheimer's disease (AD) marker found in the cerebrospinal fluid (CSF). ADDL concentrations were consistently higher in the CSF taken from the subjects diagnosed with AD than in non-demented age-matched controls [105]. These results indicate that the universal labeling technology can be improved through the use of AuNPs to provide a rapid and sensitive testing platform for laboratory research and clinical diagnosis.
AuNP-Based Therapies
Metal-based drugs are not new to medicine; in fact, they are inspired by the existing metallic drugs used in clinical treatment of various diseases [9, 106,107,108,109]. The widely studied and clinically used metal-based drugs were derived from platinum (e.g., cisplatin, carboplatin, tetraplatin for treatment of advanced cancers), bismuth (for the treatment of infectious and gastrointestinal diseases), gold (for the treatment of arthritis) and gallium (for the treatment of cancer-related hypercalcemia) [108, 109]. The approval of cisplatin in 1978 by the FDA for the clinical treatment of cancer [107] further inspired research on other metals (such as palladium, ruthenium, rhodium) [32, 106, 110].
Owing to the bioactivities, which included anti-rheumatic, antibacterial and anticancer effects, and the biocompatibility of bulk gold [8, 9, 46, 111], AuNPs are extensively investigated for the treatment of several diseases. AuNPs displayed unique and novel properties that are superior to its bulk counterpart. AuNPs are highly stable and have a distinct SPR, which guides their application in medicine [112], as drug delivery and therapeutic agents. AuNPs have a lot of advantages over the conventional therapy; they have a longer shelf-life and can circulate long enough in the system to reach their targets [25] with [11, 49, 113] or without targeting molecules [14, 15, 24, 25, 114]. AuNPs can provide localized and selective therapeutic effects; some of the areas in which AuNPs were used in therapy are described below.
Therapeutic Effects of Untargeted AuNPs
The as-synthesized (i.e., unmodified or uncapped) AuNPs have been shown to have diverse therapeutic effects against a number of infectious [115, 116], metabolic and chronic diseases [3, 29, 50, 51]. Their antioxidant, anticancer, anti-angiogenic [3, 32], anti-inflammatory [3, 51] and weight loss [29, 50, 112] effects are beneficial for diseases such as cancer, rheumatoid arthritis, macular degeneration and obesity [5, 25, 113, 117]. The above-mentioned diseases are characterized by a leaky vasculature and highly vascularized blood vessels [5, 113], which provides the NPs an easy passage into the diseased tissues and increase the susceptibility of cells to their effects. Through the EPR effect, uncapped AuNPs can passively accumulate in the vasculature of diseased cells or tissues. Hence, AuNPs have been specifically designed to have anti-angiogenic effects in diseases where angiogenesis (the growth and extension of blood vessels from pre-existing blood vessels) spins out of control like cancer, rheumatoid arthritis, macular degeneration and obesity [5, 25, 113, 117]. Targeting and destroying the defective blood vessels prevent oxygen and nutrients from reaching the diseased cells, which results in their death. The pores in the blood vessels at the diseased site (especially in cancer and obesity) are 200–400 nm and can allow materials in this size range to pass from the vasculature into the diseased tissues and cells [14, 15, 25, 114].
The cellular uptake, localization, biodistribution, circulation and pharmacokinetics of the uncapped AuNPs rely strongly on size and shape [49]. Although these effects are applicable to all AuNPs, the biological effects of citrate-capped AuNPs (cAuNPs) are extensively studied and reviewed. Spherical cAuNPs demonstrated selective in vitro anticancer activity that was size and concentration dependent on murine and human cell lines [3, 51]. Different sizes (10, 20 and 30 nm) of cAuNPs showed differential effects in human cervical carcinoma (HeLa), murine fibroblasts (NIH3T3) and murine melanoma (B16F10) cells. The 20 and 30 nm cAuNPs showed a significant cell death in HeLa cells starting at the lowest concentration of 2.2 µg/mL, while the 10-nm NPs was toxic at concentrations ≥ 8.75 µg/mL. The activity of these NPs was negligible in the noncancerous NIH3T3 cells, especially the 10 and 20 nm. The 20 nm reduced viability by ≤ 5% at the highest concentration (35 µg/mL), and ~ 20% for the 10 and 30 nm. The IC50 values for 10, 20 and 30 nm cAuNPs in the Hela cells were 35, 2.2 and 4.4 μg/mL, respectively, while the IC50 values for noncancerous cells were higher than 35 µg/mL [3]. Using a concentration range of 0.002–2 nM, 13 nm cAuNPs induced apoptosis in rabbit articular chondrocytes and no effects were observed for 3 and 45 nm cAuNPs under the same conditions. The 13 nm cAuNPs induced mitochondrial damage and increased reactive oxygen species (ROS); these actions could not be blocked by pre-treatment with a ROS scavenger, the N-acetyl cysteine [51]. Size-dependent effects were also observed in vivo after injecting cAuNPs of various sizes (3, 5, 8, 12, 17, 37, 50 and 100 nm) into mice (8 mg/kg/week) for 4 weeks. The 8, 17, 12 and 37 nm were lethal to the mice and resulted in tissue damage and death after 14 days of treatment; the other sizes were not toxic and the mice survived the experimentation period. On the contrary, the same-size AuNPs at a concentrations up to 0.4 mM were not toxic to HeLa cells after 24 h exposure [118].
The cAuNPs can interact and accumulate nonspecifically within various tissues and organs in the body, especially in the reticuloendothelial system (RES) organs (blood, liver, spleen, lungs) [55, 119]. This was evident in high-fat (HF) diet-induced obese Wistar rats [55] and Sprague–Dawley rats [119] following acute (1 dose for 24 h) [55] and chronic (1 dose; 0.9, 9 and 90 µg/week over 7 week period) [119] exposure to 14 nm cAuNPs, respectively. Majority of the i.v injected cAuNPs were detected in the liver, spleen, pancreas, lungs, kidneys [55, 119] including the skeleton and carcass of the rats [119].陈等人。 observed that after intraperitoneal (i.p) injection of a single dose (7.85 µg/g bodyweight) of 21 nm cAuNPs in lean C57BL/6 mice, they accumulated in the abdominal fat tissues and liver after 24–72 h [29], as well as the spleen, kidney, brain and heart in the HF-induced obese mice that were injected with the same dose daily for 9 weeks [50]. The cAuNPs reduced the abdominal WATs (retroperitoneal and mesenteric) mass and blood glucose levels 72 h post-injection [29]. In the diet-induced obese mice, the 21 nm cAuNPs demonstrated anti-inflammatory and anti-obesity effects [50]. They also improved glucose tolerance, enhanced the expression of inflammatory and metabolic markers in the retroperitoneal WATs and liver [50]. Both the 14 and 21 nm cAuNPs showed no sign of toxicity or changes in the markers associated with kidney and liver damage [29, 55, 119].
Similar findings were reported for plant-mediated AuNPs, without targeting molecules they can access, ablate tumors [40, 120] and obese WATs [121] in rodents. Differential uptake, distribution and activity of biogenic AuNPs also vary depending on the size and shape of the NPs. While certain sizes can pass through the vascular network and be retained at the site of the disease; others can be easily filtered out of the system through the RES organs and the mononuclear phagocytic system as shown in Fig. 6 [15, 114]. NPs can be removed by tissue-resident macrophages (TRMs) before they reach the disease cells. Those that escape the TRMs and do not reach the disease site, especially smaller NPs (≤ 5 nm), are excreted through glomerular filtration in the kidney [25, 114]. Pre-treatment with clodronate liposomes depleted the TRMs in the liver and spleen before exposure to 50, 100 and 200 nm AuNPs. This reduced uptake of the AuNPs by the liver, increased their half-life in the blood as well as their accumulation at the tumor site [122]. However, TRMs are not the only obstacle that the AuNPs that rely on EPR effect for uptake must overcome. EPR effect alone can only ascertain ≤ 1% AuNP uptake [15, 114], and depletion of the TRMs prior to treatment resulted in just ≤ 2% of NPs reaching the target [122]. The success of non-targeted AuNPs depends on their ability to reach and accumulate in the diseased tissues, of which passive targeting through the EPR effect might not be efficient. The NPs also need to circulate longer, escape early clearance, and most importantly show reduced bystander effects [25, 123]. These qualities can increase bioavailability and ensure selectivity and efficacy of the AuNPs. These can further be improved by changing the surface chemistry of the AuNPs as discussed below [15, 124].
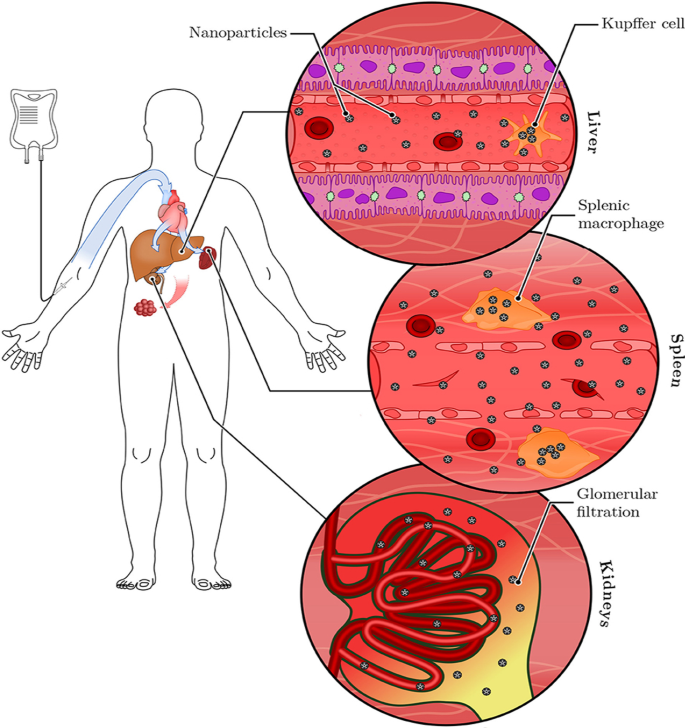
RES-based clearance of systemic administered AuNPs depends on their size. Large AuNPs accumulate in the liver, while smaller AuNPs are likely to end up in the spleen or be excreted in the urine via glomerular filtration. The AuNPs that escape the TRMs could accumulate in the diseased tissues. Reproduced with permission [114]. Copyright 2019, Frontiers in Bioengineering and Biotechnology
图>Therapeutic Effects of Surface-Functionalized AuNPs
The common strategy in AuNP-based therapeutics involves modifying the AuNP surface with therapeutic agents [3, 124,125,126]. The therapeutic agents can be drugs already used for the treatment of a particular disease or biomolecules with known inhibitory effects on cell signaling. In some instances, the therapeutic AuNPs have also been designed to have molecules that facilitate active targeting of the AuNPs toward specific cells and tissues. The molecules can easily adsorb on the AuNP surface by thiolation, chemical modification using chemistries such as 1-ethyl-3-(3-dimethylaminopropyl) carbodiimide (EDC), streptavidin/biotin binding [3, 124,125,126,127] and ionic interactions based on opposite charges between the NP surface and the biomolecules [124,125,126]. Functionalization of the AuNP surface influences their physicochemical properties and can affect their safety, biocompatibility and mobility. To ensure that the cargo carried by the AuNPs is delivered to the intended site, consideration should thus be given to both the physical and chemical properties of the AuNPs [124,125,126]. It is especially the size, shape, charge and the capping agents of the AuNPs that play an important role in the functionality of the AuNP conjugates [124] and can completely alter the pharmacokinetics of the AuNP-based therapeutics.
Functionalization allows for the development of customized nanosystems to reduce undesirable bystander effects often associated with traditional medicine. Functionalization of AuNPs can also prevent nonspecific adsorption of proteins onto the AuNP surface which can result in the formation a protein corona, resulting in the early clearance of the AuNPs through opsonization by the phagocytic cells [49, 123]. The surface charge of NPs can have a major influence on the behavior of NPs within biological environments. AuNPs with a neutral surface charge are unreactive and have a higher rate of escaping opsonization than charged AuNPs. Hydrophilic NPs will also behave differently to those with hydrophobic surfaces [49, 123]. PEG is one of the polymers most often used to mask AuNPs from phagocytic cells and has been shown to stabilize and enhance the biocompatibility of the AuNPs in numerous in vivo studies [49, 55, 123]. Pegylation improved the biocompatibility of 8.2 nm AuNPs by preventing neutrally and negatively charged AuNPs to bind to cell membranes or localize to any cellular components in African green monkey kidney (COS-1) cells [49]. And when the pegylated AuNPs were functionalized with a polyarginine cell penetrating moiety, the AuNPs were visualized on the cell membrane and inside the COS-1 cells [49]. Cell-penetrating peptides such as nuclear localization signal from SV40 virus, Tat from HIV and polyarginine peptides have been explored in translocation of AuNPs inside all cell type, normal or diseased. However, high specificity is required for clinical applications and can be achieved by taking advantage of the physiological differences between malignant and normal cells. This has been achieved by functionalizing the AuNPs with targeting molecules that recognize cell-specific receptors that are exclusively or overexpressed on the surface of target cells. This way, the AuNPs can be directed and delivered only to cells that express the target receptor. Therefore, conjugation of targeting moieties to the AuNPs (active targeting) will provide more selectivity, reduced bystander toxicity and enhanced efficacy since the AuNPs will be confined only to malignant tissues that express the target receptors [49, 55, 57, 113, 126, 127].
A good example to demonstrate the versatility of AuNPs is shown in Fig. 7, where four different molecules were conjugated onto the AuNPs to target two independent markers and mechanisms [11]. The multifunctional AuNPs were used for the treatment of leukemia (K562DR) cells that are resistant to doxorubicin (Dox). The 40 nm AuNPs were modified with two targeting moieties (folate and AS1411 aptamer) and two therapeutic agents (Dox and anti-miRNA molecules/anti-221). Folate molecule and AS1411 aptamer, respectively, recognize the folate and NCL receptors that are overexpressed on the cell surface and through receptor-mediated endocytosis will traffic the AuNP-conjugate into the cells. The AS1411 aptamer had dual functions, by also targeting the NCL receptor that is expressed inside the cells. After the AuNP-conjugate has been shuttled into the cells, the cargo (AS1411 aptamer, anti-221 and Dox) is off-loaded which independently act on three mechanisms that will synergistically bring about the demise of the cells. AS1411 aptamer together with anti-221 prevented leukemogenesis by suppressing the endogenous NCL and miR-221 function in the NCL/miR-221 pathway, thereby sensitizing the cells to the effects of Dox [11].
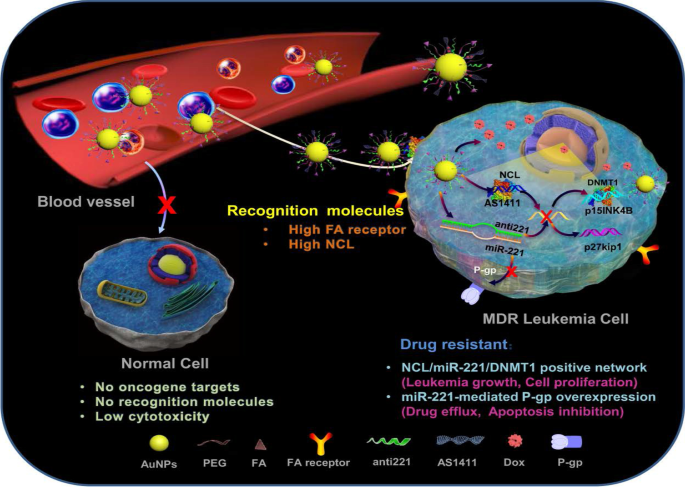
Multifunctional AuNPs in the treatment of multidrug-resistant (MDR) leukemia cells by increasing the sensitivity of the cells to Dox. Reproduced with permission [11]. Copyright 2019. Springer Nature. Folate (FA) receptor
图>Interestingly, similar dual targeting and treatment effects were achieved with green synthesized AuNPs without any additional molecules. With natural products acting as reducing agents, the biogenic AuNPs might also be more biocompatible than the chemically synthesized NPs [12, 40, 41, 43, 120]. MGF-AuNPs selectively targeted the laminin receptors in prostate (PC-3) and triple-negative breast cancer (MDA-MB-231) cells, and their xenografts in severe combined immunodeficiency (SCID) mice bearing these tumors [12, 40, 120]. In the normal SCID mice, the majority (85% at 30 min increasing to 95% after 24 h) of the i.v-injected MGF-AuNPs accumulated in the liver. Less than 10% were detected in the blood (2.7%), spleen (5%), lungs (0.6%), stomach, intestines and kidneys. When intra-tumorally injected in SCID mice-bearing prostate tumors, only 11% of the MGF-AuNPs were detected in the liver 24 h post-injection, while ~ 80% was in the tumor. Negligible amounts were found in the stomach, carcass and the small intestines. Some of the AuNPs were excreted through the renal and hepatic pathways in the urine and feces after 24 h [40, 120]. Nano Swarna Bhasma, a mixture consisting of AuNPs synthesized from mango peel extracts and phytochemicals from mango, turmeric, gooseberry and gum arabic, showed reduced toxicity toward normal endothelial cells after 48 h compared to the MDA-MB-231 cells [12].
Several studies have demonstrated that AuNPs have potential for clinical application. In combination with conventional drugs, it can be used to sensitize diseased cells to the drug effects [12, 128] and also prevent or reduce drug-related bystander effects [12]. AuNPs improved the pharmacokinetics of chemotherapeutic drugs, such as Dox [43, 129] and 5-fluorouracil (5-FU) [128]. Great improvements were mostly seen in the permeability and retention of drugs in the diseased cells, resulting in enhanced efficacy [130]. Dox-loaded AuNPs, which were non-toxic toward normal mouse fibroblast (L929) cells, also demonstrated selective toxicity toward fibrosarcoma tumors in mice [129]. 5-FU conjugated to the cAuNPs had better activity than 5-FU on its own in colorectal cancer cells [128]. AuNP co-treatment with chemotherapeutic drugs was highly efficient in improving the efficacy of chemotherapeutic drugs [12, 43, 128, 129, 131]. Orally ingested Nano Swarna Bhasma in combination with Dox and Cyclophosphamide reduced tumor volumes in SCID mice-bearing breast tumor cells and also showed acceptable safety profile and reduced bystander effects of the chemotherapeutic drugs in stage IIIA/B metastatic breast cancer patients [12]. Active targeting alone can ensure that the AuNPs are directly delivered into the desired targets, achieving a balance between efficacy and toxicity while minimizing damage to healthy tissues [14, 15, 49]. Controlled drug release is also among the many advantages offered by the AuNP-based systems and is crucial as it allows for localized and selective toxicity [49]. The AuNPs can be designed in such a way that their conjugates respond to internal (glutathione displacement, enzyme cleavable linkers, pH) or external (light, heat) stimuli to function [24, 25, 34, 128].
AuNPs as Transfection Agents in Gene Therapy
The use of AuNPs in gene therapy has shown promising outcomes by facilitating the delivery of genetic material to cells to silence or enhance expression of specific genes [24, 32, 132]. Thus, AuNPs can be used as transfection reagents in gene therapy for the treatment of cancer and other genetic disorders. AuNP conjugates have demonstrated higher transfection efficiency than experimental viral and non-viral gene-delivery vectors including polycationic reagents that has been approved for clinical use [24].
AuNPs are highly conductive and well suited for use as microelectrodes during electroporation for intracellular delivery of biomolecules for disease treatment. AuNPs significantly enhanced the performance of electroporation systems and have been used successfully for the delivery of DNA into hard-to-transfect cells such as the K562 cells [133]. To prevent cell loss which is often associated with electroporation, targeting moieties can be conjugated to the AuNPs to facilitate cellular uptake of AuNP conjugates through receptor-mediated mechanisms [133]. The use of AuNPs to transfect cells with oligonucleotide molecules also has the added advantage of increasing the half-life of these biomolecules and their efficacy [24, 32].
Untargeted AuNP conjugates are passively transported into cells and rely on the surface charge and AuNP shape for efficient transfection [24, 36, 134, 135]. The charge of the biomolecules that are conjugated onto AuNP surface plays a crucial role in their transfection efficiency; for instance, AuNPs functionalized with cationic molecules produce higher transfection efficiency than AuNPs functionalized with anionic molecules. Positively charged amino acids (lysine) can be attached on the NP surface to increase the rate of transfection. AuNSs [24] and AuNRs [36, 134, 135] are commonly used for transfections, and relative to the conventional transfection reagents (X-tremeGENE and siPORT), they inhibited the expression of target gene by > 70% in vitro [134] and in vivo [135]. In these studies, transfection efficiency was quantified based on target expression using RT-PCR and immunostaining [134, 135]. As transfection reagents, AuNPs provide long-lasting effects, localized gene delivery and higher efficacy [36, 134, 135]. Other types of nanomaterials (e.g., polymeric, liposomes, ceramic and carbon nanotubes) had received more attention for use in gene therapy than AuNPs. Six clinical trials using either polymeric or lipid-based nanomaterials for delivery of siRNA in solid tumors have been completed [36, 134, 136]. All of which suffer from low loading efficiency, low stability, and insufficient payload release [36, 136]. On the other hand, transfection systems based on AuNPs make use of easy chemistry that ensures efficient loading capacity and formation of stable complexes [36, 135]. Their safety can be controlled by manipulating their shape, size distribution and surface composition [36].
Antimicrobial Effects of AuNPs
MDR microbes are a major health concern and a leading cause of mortality, worldwide [21, 137,138,139,140,141]. These microorganisms have become resistant to conventional antimicrobial agents, due to over-prescription and misuse of these drugs [142]. No new antibiotics have been produced in over 40 years, mainly because the big pharmaceutical companies have retreated from their antibiotic research programs due to the lack of incentives [143]. As such, new and effective antimicrobial agents are urgently required to combat what could be the next pandemic, the antimicrobial resistance, and avoid surge in drug-resistant infections.
AuNPs are among the new generation of antimicrobial agents under review. They have shown broad antimicrobial (bactericidal, fungicidal and virucidal) effects against a number of pathogenic and MDR microorganisms and thus have potential to overcome microbial drug resistance [21, 142, 144]. Their antimicrobial effects are dependent on their physicochemical properties, especially their size, surface composition, charge and shape [21, 144]. Due to their small size, AuNPs can easily pass through the bacterial cell membrane, disrupt their physiological functions and induce cell death [35]. The exact antimicrobial mechanisms of AuNPs are not yet fully elucidated; despite this, some of the reported modes of actions that results from the interaction of various nanostructured materials (NSMs) with the bacterial cells are illustrated in Fig. 8. The highlighted mechanisms are also implicated in antimicrobial activity of AuNPs, they include induction of microbial death through membrane damage, generation of ROS and oxidative stress, organelle dysfunction, and alteration of gene expression and cell signaling [141].
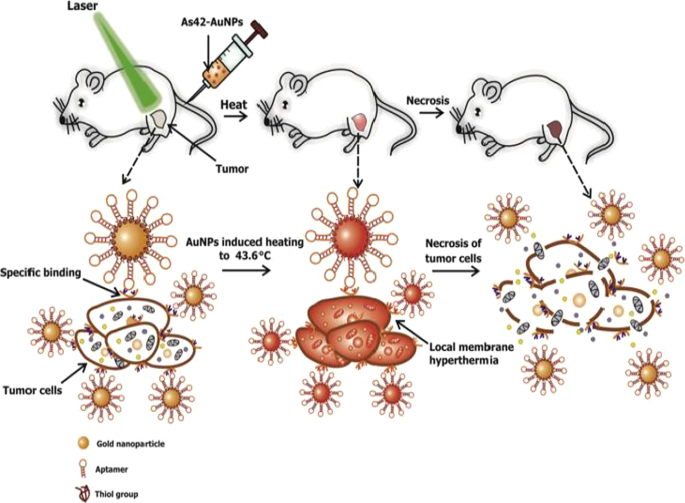
Antimicrobial mode of actions of the NSMs. Various NSMs can induce cell death by altering various biological functions, X represents alteration of cell signaling by de-phosphorylation of tyrosine residues in proteins as one of the mechanisms. Reproduced with permission [141]. Copyright 2018, Frontiers in Microbiology
图>AuNPs have multiple roles to play toward the development of antimicrobial agents, aside from being antimicrobial agents by themselves; they can serve as drug sensitizers and drug delivery vehicles [35, 58, 132, 145]. These features are applicable to both the chemical and green synthesized AuNPs, which have been reported to have antimicrobial effects against a number of human [21, 145,146,147] and waterborne [148] pathogenic strains. Generally, the test bacteria had shown low susceptibility toward the chemically synthesized AuNPs, i.e., the cAuNPs [21, 146, 147] and the NaBH4-reduced AuNPs [149]. This was due to the repulsive forces between the negative charges on the AuNP surfaces and bacterial cells, thus preventing the interaction between AuNPs and the bacteria [21]. The activity of chemically synthesized AuNPs is based on their size, shape, concentration and exposure time. As an example, one study reported that NaBH4-reduced AuNPs had no activity against Staphylococcus aureus (S. aureus ) and Escherichia coli (E. coli ) at 500 µg/mL for the duration of 6 h [149]. In contrast, another study showed a significant dose (1.35, 2.03 and 2.7 μg/mL) and size (6–34 nm vs 20–40 nm) dependent antibacterial effects of the NaBH4-reduced AuNPs on Klebsiella pneumonia , E. coli , S. aureus and Bacillus subtilis [145].
The AuNPs are either used alone or in combination with other antimicrobial agents to treat microbial infections [35, 58, 132, 145]. When used in combination with other antimicrobial agents, the AuNP conjugates resulted in synergistic antimicrobial effects that surpassed the individual effects of the AuNPs and drugs [21, 35, 58, 132, 150]. These drugs were conjugated onto the AuNPs by either chemical methods [4, 151] or the drugs were used as reducing and capping agents [21, 149]. By so doing, the AuNPs improved drug delivery, uptake, sensitivity and efficacy. Some of the FDA-approved antibiotics and non-antibiotic drugs that were loaded onto the AuNPs are shown in Table 1 [4, 21, 149, 152]. Ciprofloxacin [152], cefaclor [149], lincomycin [4], kanamycin [21], vancomycin, ampicillin [151] and rifampicin [32] are among the antibiotics loaded on the AuNPs and demonstrated the versatility of AuNPs. These strategies were successful with various sizes and shapes of AuNPs, including gold silica nanoshells [152], AuNP-assembled rosette nanotubes [151] and AuNPs encapsulated in multi-block copolymers [153]. For instance, cefaclor-reduced AuNSs inhibited the growth of S. aureus and E. coli within 2–6 h depending on the concentration (10–50 µg/mL), while complete bacterial growth inhibition by the drug alone was only observed at 50 µg/mL after 6 h. The minimum inhibitory concentration (MIC) of the treatments was 10 µg/mL and 50 µg/mL for cefaclor-AuNPs and cefaclor, respectively [149].
图>AuNPs have presented properties that make them ideal candidates as alternative antimicrobial agents; the most important being their broad antimicrobial activity [21, 35, 58, 132, 150]. Owing to their biocompatibility and easily modifiable surface, microorganisms are less prone to developing resistance toward AuNPs [21]. For example, the kanamycin (Kan)-resistant bacteria (S . bovis , S . epidermidis , E . aerogenes , P . aeruginosa and Y . pestis ) showed increased susceptibility toward Kan-reduced AuNPs. The MIC values for Kan-AuNPs on the test bacteria were significantly reduced to < 10 µg/mL when compared to the MIC values for Kan alone at 50–512 µg/mL. This shows that AuNPs can restore the potency of antibiotics toward the drug-resistant strains by facilitating the uptake and delivery of the antimicrobial agents [21]. AuNPs can enhance drug-loading capacity and control the rate at which the drugs are released. AuNP hybrids with the multi-block copolymers increased the loading capacity of rifampicin and the drug’s half-life to 240 h. By sustaining the drug in the system for that long, ensured slow release of rifampicin from AuNPs at the target sites after oral administration of the AuNP conjugates to rats for 15 days. The drug on the surface was released within 24 h followed by the drug trapped in the polymer matrix after 100 h. And lastly, the drug entrapped between the AuNPs and the polymer matrix took over 240 h to be released in the interstitial space [153].
The AuNP hybrids also allow for the conjugation of multiple molecules with independent but synergistic functions. This was demonstrated by co-functionalization of the AuNPs with antimicrobial peptide (LL37) and the pcDNA that encode for pro-angiogenic factor (vascular endothelial growth factor, VEGF) and used in the treatment of MRSA-infected diabetic wounds in mice [132]. The AuNPs served dual functions, as a vehicle for the biomolecules, and also as transfection agent for the pcDNA. After topical application of the AuNP conjugates on the wound, the LL37 reduced MRSA colonies, while the pcDNA promoted wound healing by inducing angiogenesis through the expression of VEGF [132].
AuNPs have been shown to confer activity and repurpose some non-antibiotic drugs toward antimicrobial activity. The examples of repurposed drugs, which were used for the treatment of diseases other than bacterial infections, include 5FU [58], metformin [147] and 4,6-diamino-2-pyrimidinethiol (DAPT) [13, 112]. AuNPs as drug carriers are able to transport the drugs into the cells and allow direct contact with cellular organelles that resulted in their death [58, 147]. 5FU is an anti-leukemic drug, when attached to AuNPs was shown to kill some bacterial (Micrococcus luteus , S. aureus , P. aeruginosa , E. coli ) and fungal (Aspergillus fumigatus , Aspergillus niger ) strains [58]. While bacteria are resistant to DAPT, DAPT-AuNPs displayed differential antibacterial activity against the Gram-negative bacteria. Furthermore, conjugation of non-antibiotic drugs (e.g., guanidine, metformin, 1-(3-chlorophenyl)biguanide, chloroquine diphosphate, acetylcholine chloride, and melamine) as co-ligands with DAPT on AuNPs exerted non-selective antibacterial activity and a two–fourfold increased activity against Gram-negative bacteria [13]. When used in vivo, orally ingested DAPT-AuNPs showed better protection by increasing the intestinal microflora in E. coli -infected mice. After 4 weeks of treatment, the DAPT-AuNPs cleared the E. coli infection with no sign of mitochondrial damage, inflammation (increase in firmicutes ) or metabolic disorders (reduction in bacteroidetes ) in the mice [112].
The virucidal effects of the AuNP-based systems have been reported against several infectious diseases caused by influenza, measles [154], dengue [155, 156] and human immunodeficiency [115] viruses. Their anti-viral activity was attributed to the ability of AuNPs to either deliver anti-viral agents, or the ability to transform inactive molecules into virucidal agents [154, 156]. AuNPs synthesized using garlic water extracts inhibited measles viral growth in Vero cells infected with the measles virus. When the cells were exposed to both the virus and AuNPs at the same time, they blocked infection of Vero cells by the measles virus [154]. The AuNPs were nontoxic to the Vero cells up to a concentration of 100 µg/mL but inhibited viral uptake by 50% within 15–30 min at a concentration of 8.8 μg/mL [154]. Based on the Plaque Formation Unit assay, the viral load was reduced by 92% after 6 h exposure to 8.8 μg/mL of the AuNPs. The AuNPs interacted with the virus directly and blocked its transmission into the cells [154]. Modification of the AuNP surface with ligands that bind to the virus [156] or anti-viral agents [115, 155] protected them from degradation, enhanced their uptake and delivery onto the cells. The charge of the AuNPs also played a role, with cationic AuNPs being more effective in the delivery and efficacy of the AuNPs than the anionic and neutrally charged NPs. Cationic AuNPs complexed with siRNA inhibited dengue virus-2 replication in dengue virus-2-infected Vero and HepG-2 cells and also the virus infection following pre-treatment of the virus with AuNPs [155]. Inactive molecules are transformed into highly potent anti-viral agents after conjugation to AuNPs. One such example is the transformation of SDC-1721 peptide, a derivative of TAK-779, which is an antagonist of CCR5 and CXCR3 receptors for HIV-1 strain. SDC-1721 has no activity against the HIV-1, but when conjugated to the AuNPs it inhibited HIV-1 infection of the human phytohemagglutinin-stimulated peripheral blood mononuclear cells. The inhibitory effects of SDC-1721-AuNPs were comparable to the TAK-779 [115].
AuNPs as PT Agents
Diseased cells are sensitive to temperatures above 40 °C; cancer cells in particular appear to be even more sensitive to these high temperatures. Studies have shown that high fevers in cancer patients either reduced the symptoms of cancer or completely eradicated the tumors as a result of erysipelas infections [33, 157, 158]. Historically, fevers induced by bacterial infections, hot desert sand bath, or hot baths were used to increase the body temperature in order to kill the cancer cells [157]. These findings gave birth to PT therapy (PTT), which is mostly used for the treatment of cancer. PTT makes use of organic photosensitizers (indocyanine green, phthalocyanine, heptamethine cyanine) that are irradiated by the external source to generate heat energy that will increase the temperature to 40–45 °C (hyperthermia) in the target cells. Hyperthermia then triggers a chain of events (such as cell lysis, denaturation of the genetic materials and proteins), resulting in the destruction of the diseased cells [57, 158,159,160].
The organic dyes are used alone, or in combination with chemotherapy and radiotherapy for enhanced efficacy [157, 160]. Ideally, the effects of the PT agents must be confined to target cells and display minimal bystander effects. However, the organic PT dyes have several limitations such as toxic bystander effects, susceptibility to photobleaching and biodegradation [159]. In recent years, AuNPs are being explored as alternative PT agents as they exhibit strong plasmonic PT properties, and depending on their shape, they can absorb visible or NIR light. Absorption of light in the NIR spectrum is an added advantage that can allow deep tissue PTT [158, 161, 162]. Unlike organic dyes, AuNPs operate in an optical window where the absorption of light by interfering biological PT agents such as hemoglobin, melanin, cytochromes and water is very low [158, 161, 162].
The practicality of AuNP-based PTT has been demonstrated through in vitro and in vivo studies [158, 162, 163]. When the AuNPs are exposed to light, they can convert the absorbed light energy into thermal energy within picoseconds [57, 158, 159], consequently activating cell death via necrosis or apoptosis in the target cells or tissues. AuNP-based hyperthermia in diseased cells has been reported to occur at half the amount of the energy required to kill normal cells, thus perceived to be safer and better PT agents than the conventional dyes [33, 160]. AuNPs can be easily modified to have localized and enhanced PT activity by targeting and accumulating in only diseased cells through either active or passive targeting. And since the tumor environment is already hypoxic, acidic, nutrient starved and have leaky vasculature, the tumors will be most sensitive to the AuNP-based hyperthermia than the surrounding healthy cells and tissues [33, 160].
AuNP-based PTT has been extensively studied [158, 161, 162] and established that AuNPs (e.g., AuNRs, nanocages and nanoshells) that absorb light in the NIR spectrum are best for in vivo and deep tissue PTT [161]. While the ones that absorb and emit light in the visible spectrum (AuNSs and hollow AuNPs) have been demonstrated to treat diseases that affect shallow tissues (up to a depth of 1 mm), which could be of benefit to superficial tumors [158, 161, 162], ocular surgery [164, 165], focal therapy and vocal cord surgery [158, 165]. Although the PTT effects of AuNSs are limited in vivo or for use in deep tissues, combination therapy or active targeting can be incorporated to facilitate target-specific effects [158, 161, 163]. The AuNPs in the combination therapy will serve dual functions as both drug sensitizer and a PT agent, and was shown to enhance anticancer effects of chemotherapeutic drugs [158, 162, 163]. AuNS-Dox combination demonstrated enhanced cancer cell death after laser exposure when compared to the individual effects of the AuNSs and Dox with and without laser treatment [158].
Active targeting on its own can also improve AuNP uptake, localization and target-specific PT effects, which can be viewed in real time by adding fluorophores. AuNSs (25 nm) loaded with transferrin targeting molecules and FITC were shown to accumulate and destroy human breast cancer cells at a higher rate than in non-cancer cells and had better efficacy than the untargeted AuNSs [57]. An independent study also demonstrated that DNA aptamers (As42)-loaded AuNSs (As42-AuNP) induced selective necrosis in Ehrlich carcinoma cells that express HSPA8 protein, a receptor for the aptamers. None of these effects were observed in blood and liver cells mixed with target cells, or cells treated with the AuNSs without laser treatment [163]. The PT effects of the As42-AuNP were replicated in mice transplanted with Ehrlich carcinoma cells in their right leg. As shown in Fig. 9, tail-vein injections of As42-AuNPs followed by laser irradiation resulted in targeted PT destruction of the cancer cells. The As42-AuNPs reduced tumor size in a time-dependent manner; cell death was attributed to increased temperature up to 46 °C at the tumor site. The tumor in mice treated with As42-AuNPs without laser treatment and the AuNPs conjugated with nonspecific DNA oligonucleotide continued to grow but at the lower rate compared to mice injected with PBS. This suggests that the AuNPs were also localized in the tumor [163]. In cases where AuNSs are not efficient for deep tissue PTT, other shapes such as nanocages, nanoshells and AuNRs can be used [158]. Alternately, the visible light absorption of the AuNSs can be shifted to NIR by using processes such as two-photon excitation [57].
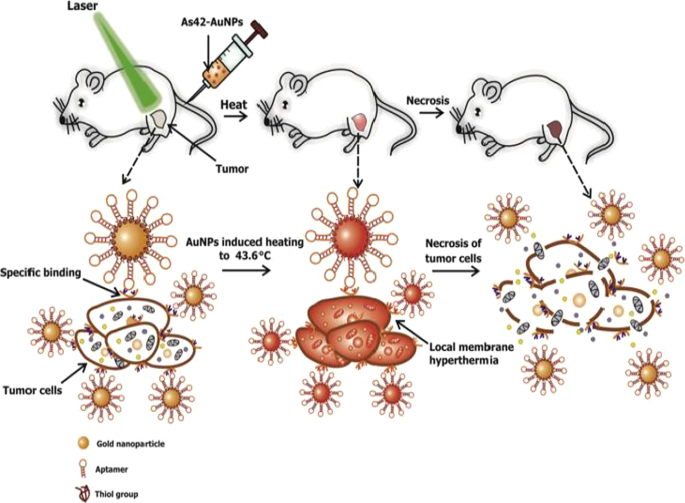
In vivo plasmonic PT therapy of cancer cells using targeted AuNSs. As42-AuNPs localized in HSPA8-expressing tumor cells after i.v injection. Exposure to laser treatment resulted in hyperthermia that caused cancer cell death. Reproduced with permission [163]. Copyright 2017, Elsevier
图>The PT effects of the AuNPs have also been reported for the reversal of obesity [52, 56], using hollow AuNSs (HAuNSs) [52] and AuNRs [56] for the PT lipolysis of the subcutaneous white adipose tissue (sWAT) in obese animals. The HAuNSs were modified with hyaluronate and adipocyte targeting peptide (ATP) to produce HA–HAuNS–ATP conjugate [52]. Hyaluronate was used to ensure topical entry of the HA–HAuNS–ATP through the skin [52, 166], while ATP will recognize and bind to prohibitin once the HAuNSs are internalized. Prohibitin is a receptor that is differentially expressed by the endothelial cells found in the WAT vasculature of obese subjects [5, 52, 55]. The HA–HAuNS–ATP was topically applied in the abdominal region of the obese mice, and through hyaluronate were transdermally shuttled through the epidermis into the dermis where the ATP located the sWATs (Fig. 10) . Illumination of the target site with the NIR laser selectively induced PT lipolysis of the sWAT in the obese mice and reduced their body weight [52]. The AuNRs were used in the photothermolysis-assisted liposuction of the sWATs in Yucatan mini pigs. The untargeted PEG-coated AuNRs (termed NanoLipo) were injected in the sWATs through an incision, followed by laser illumination to heat up the sWATs, which was then aspirated using liposuction. The amount of fat removed from NanoLipo-treated porcine was more than the one removed with conventional suction-assisted lipectomy (SAL). NanoLipo-assisted fat removal had several advantages over the conventional SAL; it took less time (4 min) for liposuction compared to 10 min for SAL, the swelling in the treated site healed faster, and the weight loss effects lasted over 3 months post-liposuction [56].
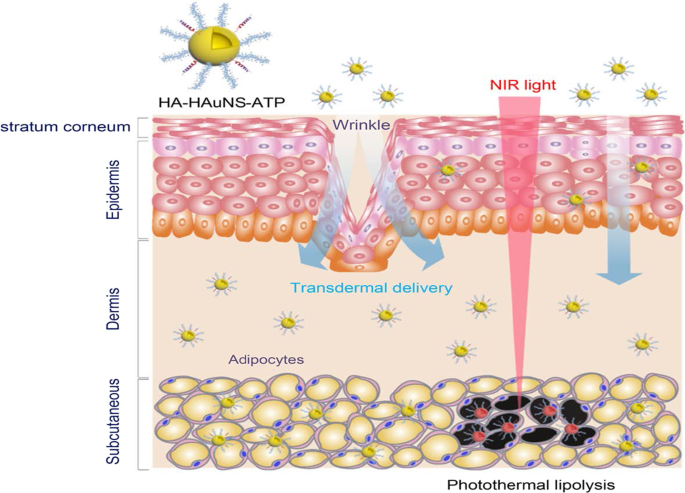
PT lipolysis of the sWATs using HA-HAuNS-ATP. The ATP was conjugated to the AuNSs for targeted delivery and destruction of the prohibitin-expressing sWATs after NIR laser exposure. Reproduced with permission [52]. Copyright 2017, American Chemical Society
图>AuNP-based PTT clearly offers a lot of advantages compared to the conventional agents. Their biocompatibility allows for broader applications both in vitro and in vivo. Moreover, they can be customized based on their shapes for shallow (AuNSs) [158, 161, 162] or deep tissue (AuNRs and stars) PTT [158, 161]. At 1–100 nm diameter, AuNPs and its conjugates can circulate long enough to reach and accumulate in the target tissues, with or without targeting moieties [159, 167]. Active targeting can be used to ensure localized PT effects through various routes of administration and might be effective for solid and systemic diseases. AuNP-based PTT can also be used to sensitize cancer cells when administered in combination with chemotherapy, gene therapy and immunotherapy [159]. Therefore, AuNP-based PTT has potential for treatment of chronic diseases [161].
Toxicity of AuNPs
AuNPs can play an important role in medicine, as demonstrated by the preclinical and clinical studies under review. Their full potential in clinical application as both diagnostic and therapeutic agents can only be realized if they do not pose any health and environmental hazards. While their use in vitro appears to be inconsequential, in vivo application can be hampered by their potential toxicity, which could be detrimental to human health. A major concern with their clinical use is that AuNPs are non-biodegradable and their fate in biological systems has not been fully studied [5, 30]. Although AuNPs are considered to be bio-inert and compatible, their properties (size, shape, charge and composition) raise concerns as they can alter their pharmacokinetics when used in biological environment [27, 34, 118]. The toxicity of AuNPs of varying sizes and shapes has been demonstrated in animals [27, 118]. These NPs can accumulate in the RES organs where they induce damage.
AuNPs are 1–100 nm in diameter which makes them smaller than most of the cellular components. At these sizes, AuNPs can passively transverse cellular barriers and blood vessels by taking advantage of the EPR effect in pathological cells. AuNPs with smaller diameters (1–2 nm) can easily penetrate cell membranes and biologically important cellular organelles such as mitochondria and nuclei [7, 168]. Accumulation of AuNPs in these organelles induces irreversible damage that can cause cellular demise. On the contrary, AuNPs larger than 15 nm are restricted to the cytoplasmic spaces and unable to penetrate internal organelles [168]. These features are desirable for targeting pathological cells, however, AuNPs can also be taken up by healthy cells and alter their physiology [118]. Administration of AuNP-based therapeutics can be done via different routes (i.e., intranasal, oral, transdermal, i.p or i.v) and transported through blood vessels into different tissues and organs [34, 118]. They are able to pass through the blood brain barrier and the placental barrier [34]. Toxicity is size dependent, with certain sizes of AuNPs being well tolerated, while others could be lethal to healthy tissues. Unfunctionalized AuNSs at 8, 17, 12, 37 nm caused physical changes (i.e., change the fur color, loss of bodyweight, camel-like back and crooked spine) within 14 days of treatment (2 doses of 8 mg/kg/week) in rats [118]. Most (> 50%) of the rats died within 21 days (i.e., after 3 doses), and abnormalities in the RES organs (liver, lungs and spleen) were observed. On the contrary, mice treated with 3, 5, 50 and 100 nm AuNPs were not affected by the NPs and no adverse effects or death occurred throughout the duration (50 days) of the study [118]. In diet-induced obese rats that received i.v injections of 14 nm cAuNPs, the NPs were detected in various tissues after 24 h and were mostly confined to the RES organs [55].
The shape, charge and surface chemistry of AuNPs can influence their toxicity. These factors can determine how AuNPs will interact with the biological systems, their cellular uptake and effects on the cells. AuNSs are readily taken up by cells and proven to be less toxic than other shapes such as rods and stars. AuNP surfaces are charged and will influence how they interact and behave within a biological environment [169]. Cationic AuNPs are likely to be more toxic compared to neutral and anionic AuNPs, as their charge allows these NPs to easily interact with negatively charged cell membranes and biomolecules such as DNA. Both the positively and negatively charged AuNPs have been associated with mitochondrial stress, which was not observed with the neutrally charged AuNPs [34, 35].
The shell that forms on the surface of the AuNP core can also influence the functioning of the NPs. These are usually reducing and/ or stabilizing agents such as citrate and CTAB, and once subjected to a biological environment, these molecules can cause either the desorption or absorption of biomolecules found in the biological environment. This can result in the formation of a corona or cause the NPs to become unstable. Citrate- and CTAB-capped AuNPs are highly reactive, which can facilitate the attachment of biocompatible polymers such as PEG, polyvinyl-pyrrolidone, poly (acrylic acid), poly(allylamine hydrochloride), and polyvinyl-alcohol) or biomolecules such as albumin and glutathione to prevent the formation of AuNP-corona with serum proteins. These molecules serve as a stabilizing agent and form a protective layer that can mask the AuNPs from attacks by phagocytes [7, 29, 34, 170] and prevent off-target toxicity [7]. As discussed in “AuNP-Based Therapies” section, AuNPs can be functionalized with targeting and therapeutic agents to define their targets and effects [34].
In addition to their physicochemical properties, the dosage, exposure time and environmental settings also influence the activity of AuNPs. Lower doses and short-term exposure times might render AuNP as nontoxic, while increasing these parameters will lead to cytotoxic effects [34]. Moreover, in vitro studies do not always simulate in vivo studies. At times, AuNPs that seem to be nontoxic in cell culture-based experiments end up being toxic in animal experiments. Many factors could be responsible for these discrepancies [118], and some steps have been identified that can guarantee the safety of AuNPs in biomedical applications. The biocompatibility and target specificity of AuNPs can be improved by modifying the surface of the NPs. Attaching targeting moieties on the AuNPs can channel and restrict their effects to specific targets or pathological cells [5, 55, 127]. Modification of AuNP surface with bio-active peptides provides a platform for developing multifunctional AuNPs with enhanced specificity, efficacy and potentially sustainable effects [11, 127]. All of these effects will be instrumental in the design and development of AuNP-based systems for clinical applications.
Clinical Application of AuNPs
Nanotechnology has the potential to shape the future of healthcare systems and their outcomes. Its promise of creating highly sensitive and effective nanosystems for medicine has been realized with the introduction of organic nanoformulations for cancer treatment. These systems have already paved the way for nanomaterials into clinical applications:doxil and abraxane have been in the market for over two decades and demonstrated the potential of nanotechnology in medicine [1, 2]. More recently, this technology has been used for the development of the SARS-CoV-2 lipid NP-based vaccine to fight against the COVID-19 pandemic [171]. Inorganic nanosystems such as AuNPs offer many advantages over their organic counterparts, yet few of these systems are used clinically (Table 2) [19, 32].
图>While several AuNP-based drugs are some of the inorganic nanomaterial-based drugs that were tested in clinical trials, they are not progressing at the same rate as organic liposome-based nanodrugs. Aurimune (CYT-6091) and aurolase were the first of AuNP-based formulations to undergo human clinical trials for the treatment of solid tumors. CYT-6091 clinical trials started in 2005 for delivery of recombinant TNF-α as an anticancer therapy in late-stage pancreatic, breast, colon, melanoma, sarcoma and lung cancer patients. CYT-6091 consists of 27-nm cAuNPs loaded with TNF-α and thiolated PEG. The CYT-6091 nanodrug has achieved safety and targeted biologic response at the tumor site at a dose lower than that required for TNF-α alone [16, 17]. CYT-6091 is approved and yet to start phase II clinical trials in combination with chemotherapy. Based on phase II clinical trial strategy, several variants of CYT-6091 have been developed and tested in preclinical studies. All the nanosystems contain TNF-α with either chemotherapy (paclitaxel, dox and gemcitabine), immunotherapy (Interferon gamma) or apoptosis inducing agents attached to the 27 nm cAuNPs [14,15,16]. The AuNP conjugates preferentially accumulated in the tumor sites after systemic administration through the EPR effect and vascular targeting effects of the TNF-α. The AuNPs were not detected in the healthy tissues, and the anti-tumor effects of TNF-α were restricted to the tumor environment [14, 16, 19].
The first clinical trial for the PT treatment with AuroLase® for refractory and/or recurrent head and neck cancers was completed. Information on the outcome of this trial is still pending. The second trial is set to evaluate the effects of AuroLase® on primary and/or metastatic lung tumors in patients where the airway is obstructed [19]. The number of human trials based on AuNP-based formulation is increasing, covering the treatment of a wide range of medical conditions including skin, oral, heart and neurological diseases. AuNP-formulation (150 nm silica-gold nanoshells coated with PEG), which is similar to AuroLase®, was approved for PT treatment of moderate-to-severe inflammatory acne vulgaris. The nanoshells were topically applied on the acne area and transdermally delivered into the follicles and sebaceous ducts through low-frequency ultrasound or massage. Nanoshells applied through massage were effective in penetrating the shallow skin infundibulum (90%) and the sebaceous gland (20%), while the low-frequency ultrasound can penetrate both shallow and deep skin tissues. NIR laser treatment resulted in focal thermolysis of the sebaceous glands in the affected area and disappearance of the acne [18, 167]. The gold–silica nanoshells were well-tolerated, showed no systemic toxic effects with minor side effects (reddiness and swelling) at the treatment site [18]. AuNPs offer many health benefits based on their unique properties but at the same time have raised a lot of political and ethical issues, and resulted in termination of some clinical studies (NCT01436123).
Conclusion and Future Perspectives
Applications of AuNPs in biomedicine are endorsed by their unique physicochemical properties and have shown great promise as theranostic agents. The increasing interest in biomedical applications of AuNPs is further encouraged by the biocompatibility and medical history of bulk gold, which suggests that the gold core in AuNPs will essentially display similar or improved properties [3]. But at the same time their small size can infer unique properties that will completely change their pharmacokinetics [144]. The diverse biomedical applications of AuNPs in diagnostics and therapeutics herein discussed demonstrate their potential to serve as adjunct theranostic agents. They can be used as drug delivery, PTT, diagnostic and molecular imaging agents [12, 33, 128]. In time, and with better knowledge of mechanisms of action, more AuNP-based systems will obtain approval for clinical use. However, the excitement of these biomedical applications of AuNPs should unequivocally be balanced with testing and validation of their safety in living systems before any clinical applications.
In conclusion, more work needs to be done to taper the toxicity of AuNPs. This can be achieved by introducing biocompatible molecules on their surface [14, 15, 58, 159], and developing new and better synthesis methods, such as the use of green chemistry to produce biogenic NPs. All these developments may further broaden the applications of AuNPs in nanomedicine. AuNPs are non-biodegradable, and off-target distribution could result in chronic and lethal effects. All these concerns must be addressed before clinical translation; the existing trials will soon provide some clarity on their impact in human health. Should their health benefits outweigh their potential risks as is the case with the existing clinical drugs, it is a matter of time before they are approved for clinical use.
Availability of Data and Materials
All the information in this paper was obtained from the studies that are already published and referenced accordingly.
Abbreviations
- 5-FU:
-
5-Fluorouracil
- AD:
-
Alzheimer's disease
- ADDLs:
-
Amyloid-beta-derived diffusible ligands
- ASOs:
-
Antisense oligonucleotides
- AuNPs:
-
Gold nanoparticles
- AuNPsQ:
-
Quantum-sized AuNPs
- AuNRs:
-
Gold nanorods
- AuNSs:
-
Gold nanospheres
- BCA:
-
Bio-barcoding assay
- cAuNPs:
-
Citrate-capped AuNPs
- COVID-19:
-
Corona virus disease 2019
- CSF:
-
Cerebrospinal fluid
- CT:
-
Computed tomography
- CTAB:
-
Cetyltrimethylammonium bromide
- DABCYL:
-
4-((4′-(Dimethyl-amino)-phenyl)-azo)benzoic acid
- DAPT:
-
4,6-Diamino-2-pyrimidinethiol
- Dox:
-
Doxorubicin
- EDC:
-
1-Ethyl-3-(3-dimethylaminopropyl) carbodiimide
- EPR:
-
Enhanced permeability and retention
- FA:
-
Folate
- FDA:
-
Food and Drug Administration
- FITC:
-
Fluorescein isothiocyanate
- FRET:
-
Fluorescence resonance energy transfer
- HAuNSs:
-
Hollow AuNSs
- HF:
-
High-fat
- HU −1 :
-
Hounsfield unit
- LFAs:
-
Lateral flow assays
- LSPR:
-
Localized surface plasmon resonance
- MDR:
-
Multidrug resistant
- MGF:
-
Mangiferin
- MPA:
-
Mercaptopropionic acid
- MWPLP:
-
Microwave-induced plasma-in-liquid process
- NCL:
-
Nucleolin
- NIR:
-
Near-infrared
- NSMs:
-
Nanostructured materials
- P. jirovecii :
-
Pneumocystis jirovecii
- PEG:
-
Polyethylene glycol
- PSA:
-
Prostate-specific antigen
- PSMA:
-
Prostate-specific membrane antigen
- PT:
-
Photothermal
- PTT:
-
Photothermal therapy
- QDs:
-
Quantum dots
- RES:
-
Reticuloendothelial system
- ROS:
-
Reactive oxygen species
- SARS-CoV-2:
-
Severe acute respiratory syndrome-coronavirus-2
- SCID:
-
Severe combined immunodeficiency
- SPR:
-
Surface plasmon resonance
- sWAT:
-
Subcutaneous white adipose tissue
- TOAB:
-
Tetrabutylammonium bromide
- TRMs:
-
Tissue-resident macrophages
- VEGF:
-
Vascular endothelial growth factor
- WAT:
-
White adipose tissue
纳米材料
- 用于化学传感器的金纳米粒子
- 用于癌症治疗的纳米粒子:当前的进展和挑战
- 用于合成和生物医学应用的荧光纳米材料的进展和挑战
- 用于超级电容器应用的石墨烯和聚合物复合材料:综述
- Au@TiO2 蛋黄-壳纳米结构的制备及其在亚甲基蓝降解和检测中的应用
- 改性超支化聚甘油作为分散剂,用于控制和稳定碳氢化合物中的金纳米粒子
- PPy 包覆的 MnO2 混合微材料的制备及其作为锂离子电池阳极的改进循环性能
- 用于生物医学应用的球形共轭金-鸟蛤壳衍生的碳酸钙纳米颗粒的制造、表征和细胞毒性
- 银纳米结构的合成方法和应用的最新进展
- 桔梗皂苷(桔梗)用于金和银纳米颗粒的绿色合成
- 圆形金纳米粒子:粒径和浓度对拟南芥根系生长的影响
- 用 Au 纳米粒子定制的二氧化硅硅藻壳可以对用于生物、安全和环境应用的分子进行灵敏分析