通过快速分解阳极氧化制备的二氧化钛纳米管,用于在紫外线和自然日光下对有机染料进行光催化脱色
摘要
二氧化钛纳米管 (TNT) 粉末在高氯酸电解液中通过快速击穿阳极氧化 (RBA) 制备。在紫外线和自然阳光照射下,通过阴离子和阳离子有机染料(即甲基橙 (MO) 和罗丹明 B (RhB) 的脱色)测试了在 250 到 550 °C 之间退火的制备和粉末的光催化效率, 作为模型污染物。纳米管的管状结构在高达 250°C 时仍保持不变,而在 350°C 及以上时,纳米管会转化为纳米棒和纳米颗粒。根据退火温度,TNT 由锐钛矿、混合锐钛矿/板钛矿或锐钛矿/金红石相组成。所制备的纳米管的带隙为 3.04 eV,并且在退火时向可见光区域移动。 X 射线光电子能谱 (XPS) 结果显示 TNT 表面存在二氧化钛和包括氯在内的杂质。退火后的 TNT 的 Ti/O 原子比保持不变,但氯的浓度随温度降低。光致发光 (PL) 表明所制备的 TNT 具有高电子空穴复合,这可能是由于结构中的残留杂质、低结晶度和空位,而在 450°C 退火的 TNT 样品中观察到最高光电流。 TNTs 在紫外光下会引起染料的小幅降解;然而,与之前的报道相反,在阳光下观察到染料完全脱色。所有 TNT 样品在日光照射下的脱色率均高于紫外光下。在阳光下使用 RhB (κ 1 =1.29 h −1 )。这归因于纳米管的带隙、比表面积和晶体结构。所制备的 TNT 对 RhB 的脱色最有效,并且在阳光照射下的性能优于参考锐钛矿粉末。这可能归因于大量的反应位点、更高的比表面积和 RhB 的降解机制。这些 RBA TNT 光催化剂粉末展示了对阳光光谱的更有效利用,使其可用于环境修复。
背景
一维二氧化钛纳米管(TNT)在过去十年中引起了广泛关注。由于它们具有良好的电学和光学特性以及高比表面积,它们已被研究用于许多前瞻性应用[1]。 TNT 最有前途的研究已在气体传感、光催化、染料敏化太阳能电池、电致变色器件和生物医学应用方面进行 [2]。 TNT 可以通过多种方法合成,包括电化学阳极氧化、水热处理、化学处理、模板辅助和溶胶-凝胶方法 [1,2,3,4]。在无模板方法中,Zwilling 等人。 [5] 1999年介绍了钛箔电化学阳极氧化制备TNT阵列。钛阳极氧化的研究通过改变电解质、电解质pH值、温度、外加电位等参数继续控制TNT阵列的形貌和尺寸,电流密度和阳极氧化时间 [6, 7]。然而,制造更大批量的 TNT 阵列,以进一步从 Ti 基板上剥离阵列以获得粉末 [8],是非常耗时的。因此,开发了一种称为快速击穿阳极氧化 (RBA) 的更快方法,在电解液中使用氯离子和高氯酸根离子 [7, 9]。在 RBA 中,金属钛在施加电压后的几秒钟内转化为金属氧化物纳米管。当施加电压时,金属表面的天然氧化物上会生长一层薄薄的氧化物,该氧化物层会迅速受到卤化物离子的攻击,在金属表面形成局部凹坑[10]。点蚀过程受施加的电压、温度和卤化物浓度的影响 [10]。点蚀后,O 2− 向内迁移,在凹坑内形成氧化层 离子从电解质到金属表面 [11]。氧化的金属离子 (Ti 4+ )向外迁移,形成的TiO2层被氯离子腐蚀形成水溶性的[TiCl6] 2− 离子 [9]。当在氧化物生长和氧化物的化学蚀刻中保持平衡时,就会形成纳米管 [9, 11]。纳米管束在几秒钟内以随机方向在凹坑周围生长。一段时间后,由于金属和氧化物纳米管之间的高应力,纳米管束落入电解质中 [9]。然后从电解质中收集纳米管粉末 [11]。由 RBA 制备的 TNT 粉末已被研究用于许多潜在的应用,例如 DSSC [12, 13]、制氢 [14,15,16,17,18,19],在紫外光照射下光催化降解污染物 [20 ,21,22] 和生物医学植入物 [23, 24]。
二氧化钛已被用于去除水流中的有机污染物,因为它是无毒、热稳定、廉价且易于获得的材料 [25]。已经使用不同的染料作为模型污染物研究了 TNT 对水中有机污染物的光催化降解 [25,26,27]。 TNT 具有高比表面积,根据合成过程的不同,可以是无定形或晶体结构。可以通过退火来改变晶体结构,同时影响纳米管的形态、带隙、组成和比表面积 [3, 11]。据报道,TNT 的带隙在 3.0-3.2 eV [2] 范围内,具体取决于纳米管的晶体结构。纳米管的晶体结构取决于合成条件,可以修饰为 TiO2 的三种多晶型,即锐钛矿、金红石和板钛矿。与金红石和板钛矿相的直接带隙相比,锐钛矿具有间接带隙并且具有更长的电子-空穴寿命[28]。纳米管由于其管状形态、更大的比表面积和更宽的带隙 [29] 在紫外光照射下有望提高光催化效率。因此,许多研究报告了 TNT 阵列通过紫外线照射降解有机染料的功效 [25,26,27]。由于紫外线只是太阳光光谱的一小部分,更有效地利用太阳能进行环境修复还需要使用可见光范围。然而,TNTs 在可见光照射下是低效的光催化剂 [29]。为了提高TNTs在可见光范围内的光催化效率,通常通过阴离子(非金属离子)或阳离子(金属离子)掺杂来改变其电子性质[27, 30,31,32]。
在本研究中,未掺杂的 TNT 粉末由 RBA 制备,并在空气中从 250 到 550°C 退火 3 小时。通过对罗丹明 B (RhB) 和甲基橙 (MO) 染料的脱色测定其效率,将所得 TNT 粉末作为光催化剂在紫外线和自然阳光照射下进行检测。染料稳定并具有独特的颜色,已用于造纸、纺织、化妆品和照相行业 [20]。已经对 TNT 阵列进行了大量光催化研究 [32];然而,关于用 RBA 制备的 TNT 粉末光催化降解有机污染物的报道很少 [20, 21, 33]。然而,据我们所知,尚未对 RBA 制备的 TNT 在自然阳光下进行染料降解研究。在这项研究中,我们发现由 RBA 制备的未掺杂 TNTs 在自然阳光下比在紫外线下更有效,并且在自然阳光照射下有机染料完全脱色。这表明可以有效利用太阳光谱进行环境修复,例如工业废水净化。
方法/实验
TNT 的准备
TNT 粉末是通过使用 0.1 M HClO4 电解质(Sigma-Aldrich,70%)和 20 V 的施加电压制备的,如前所述 [11]。对制备和退火的粉末(250-550°C,3 h)进行表征,以探索纳米管的形态、组成、晶体结构和比表面积。
表征方法
通过透射电子显微镜(TEM;Tecnai F-20G2 FEG S-twin GIF)在 200 kV 的工作电压下检查形态。使用帕纳科 X'pert Pro 衍射仪进行 X 射线衍射 (XRD) 表征以研究 TNT 的晶体结构,并在 20°–110° 的两个θ范围内进行测量,工作电压为 40 kV 和通过使用波长为 0.179 nm 的 Co-Kα 辐射,电流为 40 mA。拉曼光谱是使用 Horiba Jobin-Yvon 的 Labram HR 拉曼光谱仪获得的,该光谱仪配备了 50 mW 的 514 nm 氩激光激发。使用× 50 物镜(Olympus BX41)对 TNT 粉末进行测量。 UV /Vis/NIR光谱用于获得用于计算带隙能量的吸收光谱。使用带有积分球的 Agilent Cary 5000 设备进行漫反射光谱 (DRS) 测量。测量在 200-800 nm 范围内进行,并使用 Spectralon 校准样品进行基线校正。傅里叶变换红外光谱 (FTIR) 在衰减全反射 (ATR) 模式下进行,光谱范围为 525-4000 cm -1 , 分辨率为 4 cm −1 ,使用 Nicolet 380 FTIR。 X射线光电子能谱(XPS)用于分析表面化学成分。使用配备单色 Al Kα (1486.6 eV) X 射线源的 Kratos Analytical AXIS Ultra 系统进行测量,并使用 C 1s (284.8 eV) 作为结合能参考。使用配备 20 W 氙灯的 Perkin Elmer LS 50B 发光光谱仪在 330 nm 激发下对 TNT 粉末进行光致发光光谱 (PL)。
对于光电流表征,通过在掺氟氧化锡 (FTO) 玻璃上沉积 TNT 膜来制备电极。通过使用 TNT 粉末在乙醇中的悬浮液在 FTO 玻璃上进行滴注,然后在 70°C 的烘箱中在空气中干燥 20 分钟来制备薄膜。在薄膜上加入几滴 Nafion(Sigma-Aldrich;在低级脂肪醇和水中的 5wt.%),并将样品在烘箱中在 70°C 的空气中再次干燥 20 分钟。使用 Jaissle IMP83 PC-T-BC 恒电位仪在 500 mV 的电压下进行光电流表征。使用三电极装置进行测量,使用 Ag/AgCl 参比电极、Pt 作为对电极和沉积在 FTO 玻璃上的 TNT 膜作为工作电极。 0.1M Na2SO4(Sigma-Aldrich;≥ 99.0%)用作电解质。样品由 Oriel 6365 150 瓦氙灯在 250-600 nm 范围内照射,其中使用 Oriel Cornerstone 130 1/8 米单色器选择入射波长。使用 Gamry Referece 600+ 恒电位仪 (Gamry Instruments) 进行电化学阻抗谱 (EIS)。在测量中使用具有 Ag/AgCl 参比电极(+ 0.199 V vs RHE,辐射计分析)、TNT 工作电极和 Pt 线对电极的三电极设置。 EIS用于研究外球氧化还原探针Ru(NH3)6 2 +/3 + 的电子转移 (5 mM 在 1 M KCl 中)。使用 15 mV 的交流信号从 200 kHz 到 100 mHz 执行 EIS。用循环伏安法测定的形式电位用作测量中的直流电位。所有测量均在室温下进行。
TNT 的光催化
通过在紫外线和阳光下作为模型污染物的阴离子甲基橙(MO,Fluka,Reag.Ph.Eur)和阳离子罗丹明 B(RhB,Sigma,~ 95%)染料的水溶液脱色来研究 TNT 粉末的光催化效率辐照。使用 TNT 作为催化剂对 MO 和 RhB 染料进行光催化脱色的示意图如图 1 所示。UV 实验在配备两台飞利浦 PL-S 11W/10/2P 的内部反应器中进行紫外线灯。紫外灯的波长范围为 350–400 nm,强度为 1 mW cm −2 .通过将 100 毫克 TNT 粉末添加到 100 毫升 10 毫克 L -1 中来制备用于光催化实验的悬浮液 MO 或 RhB 水溶液。为了检查催化剂的吸附能力,将分散体在黑暗条件下放置 3 小时。然后将分散体用紫外线或自然阳光照射 3 小时以测试光催化活性。锐钛矿 TiO2 粉末(Sigma-Aldrich,99.8%)用作参考材料。还进行了空白测试以测量在紫外线和阳光下 MO 和 RhB 的光解。在t照射前测量悬浮液的初始浓度(C0) =0,即暗吸附 3 小时后。通过使用 0.45 μm 尼龙注射器过滤器和必要时离心将催化剂与染料溶液分离,以给定的时间间隔测量样品的浓度 (C)。浓度变化 (C/C0) 与吸光度变化 (A/A0) 成正比,其中 A 0 是 t 处的初始吸光度 =0. MO (λ max =465 nm) 和 RhB (λ max =554 nm) 浓度是通过用 Hitachi U-5100 UV 记录吸光度来研究的 /可见分光光度计。最低检测限为 1.0 mg L −1 对于 MO 和 0.1 mg L −1 对于 RhB。自然日光实验在芬兰埃斯波(60°11′01.3″ N 24°49′32.2″ E)于2017年6月至7月正午左右完全晴天进行。
<图片>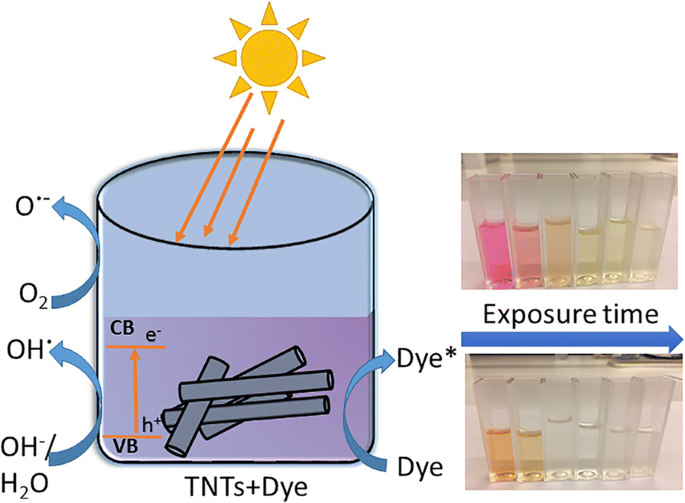
未掺杂TNTs在自然光照射下对有机染料脱色示意图
图>结果与讨论
形态和结构
在 RBA 过程中施加电压后的几秒钟内即可获得单壁 TNT 束。形成了顶部开口和底部封闭、孔径为 11-18 nm、束长在 18-35 μm 范围内的纳米管 [11]。当纳米管在 250、350、450 和 550°C 下退火 3 小时时,它们的形态和晶体结构会发生变化。这些变化在参考文献中有详细讨论。 [11]。简而言之,它们可以总结如下:当退火时,纳米管在高达 250°C 的温度下保持其形态。在更高的温度下,纳米管转变为纳米棒和纳米颗粒,如图 2a-d 所示。这种转变导致在 350°C 和更高温度下退火期间比表面积明显减小,如表 2 中所列。在更高温度(即 350-550°C)下获得的纳米棒形态也显示在附加文件中1:图S1a-c。
<图片>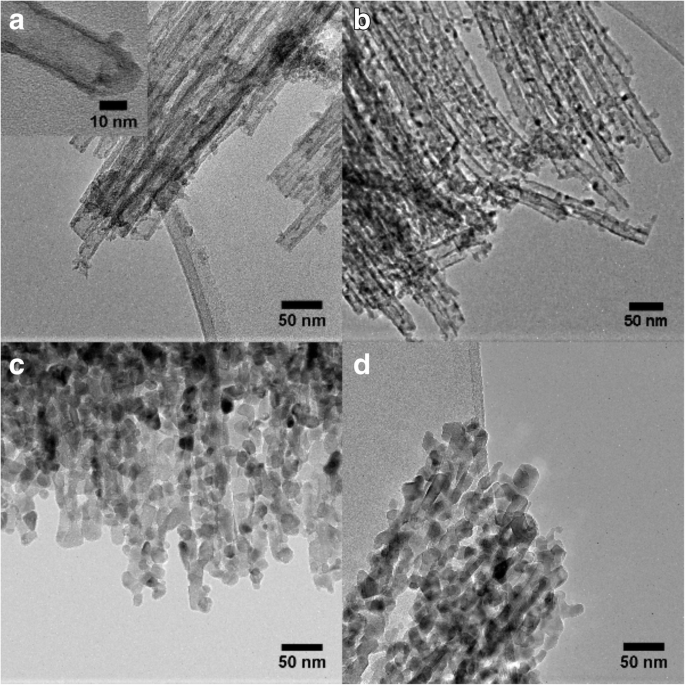
TNT 的 TEM 图像。 一 如插图所示,制备的 TNT 一端开放,另一端封闭(插图比例尺 =10 纳米),TNT 在 b 处退火 250°C,c 350 °C(纳米棒转化)和 d 450°C
图>图 3 显示了制备和退火的 TNT 的 XRD 数据。所制备的 TNT 是结晶的,并显示出锐钛矿相的衍射峰。这些结果与之前的发现非常吻合 [11, 18, 34]。对于在 250、350 和 450°C 下退火的样品,也发现了锐钛矿相。此外,TNT 450 和 TNT 250 也显示出来自板钛矿相的峰,如图 3 的插图所示。当样品在 550°C 下退火时,板钛矿峰消失。 TNT 550 显示锐钛矿和金红石相的峰,与之前的发现相似 [11]。应该指出的是,在之前的研究中退火后没有发现板钛矿峰 [34],但 Preethi 等人。 [17] 报道了用于制备 TNT 的板钛矿/锐钛矿相混合物。附加文件 1:图 S2 中显示的拉曼光谱也证实了 TNT 450 中存在板钛矿相和锐钛矿。拉曼数据显示制备的 TNT、TNT 250 和 TNT 350 中存在锐钛矿相,与之前的发现相似 [11]。 TNT 550 显示了金红石相的峰,支持 XRD 结果(附加文件 1:图 S2)。每种多晶型物的重量百分比由 Zhang 和 Banfield [35] 提出的经验公式近似得出。建议的公式如公式所示。 1a-c 低于 [16, 35, 36]。
$$ {W}_A=\frac{K_A{A}_A}{K_A{A}_A+{A}_R+{K}_B{A}_B} $$ (1a) $$ {W}_R=\frac{ A_R}{K_A{A}_A+{A}_R+{K}_B{A}_B} $$ (1b) $$ {W}_B=\frac{K_B{A}_B}{K_A{A}_A+{A }_R+{K}_B{A}_B}, $$ (1c)其中 W A , W B , 和 W R 代表锐钛矿、板钛矿和金红石的重量分数。 A A 是锐钛矿相的 (101) 峰强度,A B 代表板钛矿的强度(121),A R 代表金红石相的 (110) 峰强度,K A 和 K B 是修正系数 (K A =0.886 和 K B =2.721) [35, 36]。结果表明,TNT 450的锐钛矿相为64%,板钛矿相为36%,TNT 550为锐钛矿相为34%,金红石相为66%。
<图片>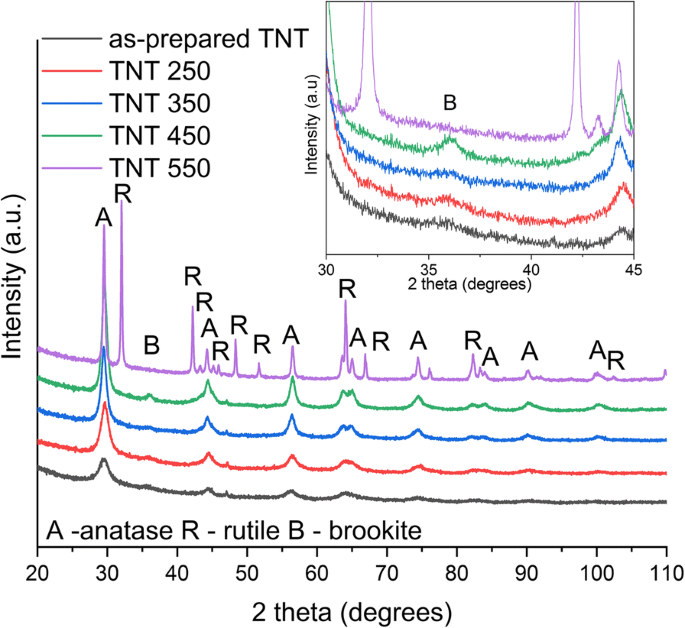
Co K-α辐射制备和退火的TNTs的XRD
图>TNT 样品的漫反射吸收光谱如图 4a 所示。漫反射光谱用于通过应用 Kubelka-Munk 方法计算带隙能量(图 4b)。所制备的纳米管的带隙约为 3.04 eV(表 2)。对于退火至 350°C 的纳米管,发现了类似的带隙尺寸。 TNT 450 和 TNT 550 的带隙能量分别为 3.14 和 2.88 eV。钛酸盐纳米管在退火时也观察到类似的可见光红移 [3]。这种向可见光范围的转变归因于退火时纳米管晶体结构的变化,因为除了锐钛矿相之外,在 550°C 还观察到金红石相 [11]。早先报道的金红石带隙值为 3.00 eV [2],板钛矿相带隙的实验结果在 3.1-3.4 eV [37] 范围内,这与我们的结果非常吻合。所制备的 TNT 的带隙和退火时带隙能量的缩小也与先前发表的结果非常吻合 [9, 34]。参考锐钛矿粉的带隙能量为 3.18 eV,与大块锐钛矿粉的带隙能量 (3.23 eV) [37] 相当。
<图片>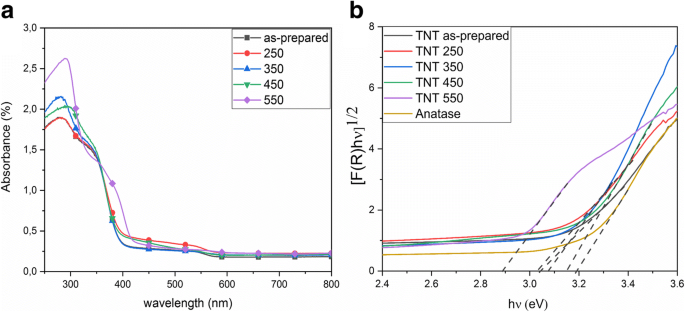
UV /Vis 漫反射 a 制备和退火 TNT 的吸收光谱 b 带隙估计的 Kubelka-Munk 函数
图>纳米管和参考锐钛矿粉末的 FTIR 如图 5 所示。突出的 O-H 弯曲(1620-1640 cm -1 ) 和伸缩振动 (3000–3500 cm −1 ) 观察到制备的 TNT,类似于之前的观察 [11]。还观察到退火 TNT 的 O-H 振动,其强度随退火温度而降低。对于参考锐钛矿粉末,没有观察到羟基振动。退火后羟基的减少与之前的结果相似 [3, 11]。应该注意到,从 2000 到 2500 cm − 1 的振动 是由于设备的人工制品造成的。
<图片>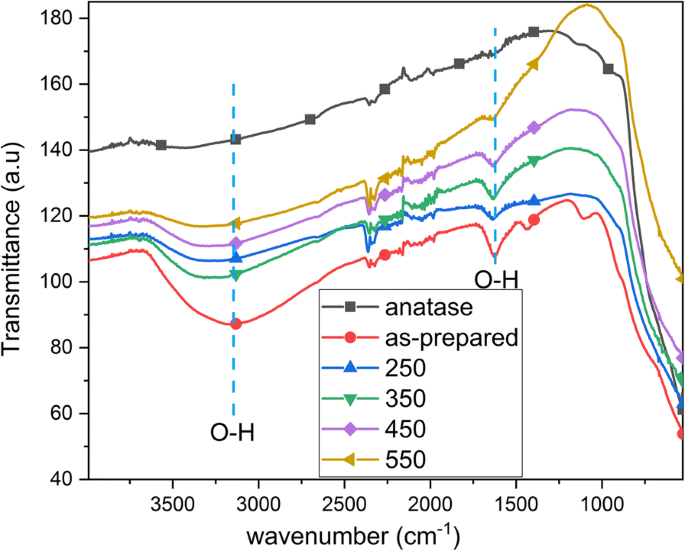
制备的 TNT、退火的 TNT(250、350、450 和 550°C)和参考锐钛矿粉的 FTIR
图>图 6 显示了从 TNT 表面获得的 XPS 光谱。调查光谱(图 6a)用于计算表 1 所示表面区域元素的原子百分比。表 1 中未显示相对量 C。图 6b 中所示的 Ti2p 光谱显示在来自对应于 TiO2 的 TNT 的 458.9 eV (Ti2p3/2) 和 464.6 eV (Ti2p1/2) [31, 38,39,40]。拟合是使用 40% 高斯峰获得的。在 TNT 中发现了这些峰,但当退火温度升高时,2p3/2 峰上的结合能逐渐向下移动 0.4 eV。这与从锐钛矿到金红石的相变一致,因为后者的 Ti2p 结合能略低 [41]。图 6c 显示了所有样品的 O1s 光谱,对 550°C 退火样品进行去卷积,显示出高斯/洛伦兹 (GL) 分量,结合能为 529.8 eV,与 O-Ti 键合[31, 38, 39, 42 ] 和 530.9 eV,这与羟基与 Ti (OH-Ti) 的存在有关 [39, 42]。 XPS 显示在制备的 TNT 表面上存在大量氯。图 6d 中的光谱有两个 Cl2p 双峰,一个在 198.6 eV (Cl2p3/2),另一个在 200.1 eV (Cl2p1/2) [43,44,45]。这些峰也存在于所有其他样品中,如图 6d 所示。氯是电解液中的残留物,退火后其含量会减少。杨等人。 [38] 还报道了在氟化物电解质中制备的 TNT 阵列退火后,氟离子的原子浓度降低。所制备的 TNT、TNT 250 和 TNT 350 在结合能 208.5 eV (Cl2p3/2) 处具有峰值,与 ClO4 - 有关 纳米管表面的离子键合 [46]。对于在较高温度下退火的样品,高结合能峰消失,如 TNT 450 和 TNT 550 的情况(图 6d)。表 1 显示了 TNT 表面元素的原子百分比和 Cl - 的还原 退火时从 2.3% 到 0.3%。 Ti/O 的比率保持不变,与之前的报告相反 [38]。
<图片>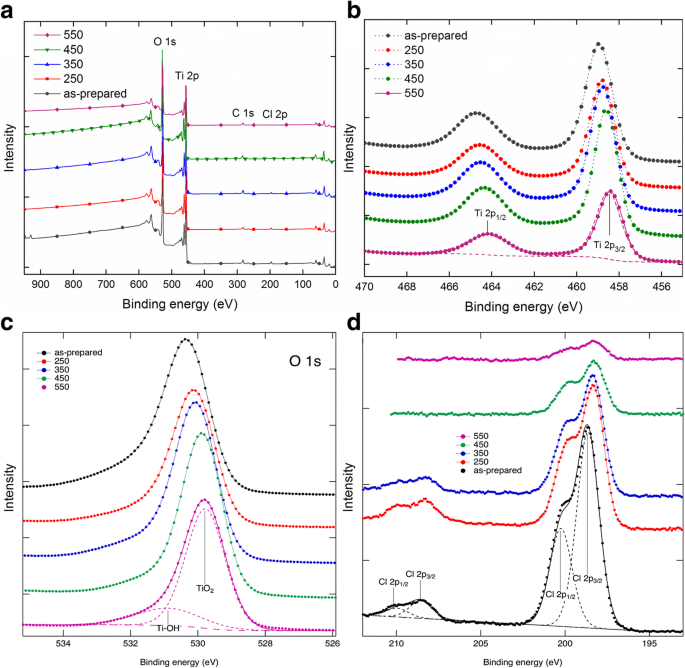
TNT a 的 XPS 光谱 b 的调查光谱和高分辨率扫描 Ti2p,c O1s 和 d Cl2p
图> 图>PL广泛用于探测光催化剂中的光生电子。图 7 显示了 TNT 粉末样品的 PL 光谱。除 TNT 550 外,所有 TNT 样品都具有以 399 nm (3.11 eV) 为中心的发射带。然而,所有样品在约 419 nm (2.96 eV) 处共享最大发射带。这两个发射带支持从漫反射光谱获得的带隙值,如图 4 所示。TNT 样品中发生的晶体结构变化也支持带隙的变化,因为 TNT 550 具有混合锐钛矿/金红石结构,和所有其他 TNT 样品具有锐钛矿或锐钛矿/板钛矿的晶体结构。安东尼等人。在混合锐钛矿/金红石 TNT 的带隙中发现了类似的红移 [34]。在大约 419 nm 处的带的 PL 强度可以按照制备的 TNT> TNT 350> TNT 250 ≈ TNT 450> TNT 550 的递减顺序排列。与其他样品相比,制备的 TNT 显示出更高的组合,可能是由于纳米管的更多缺陷和更低的结晶度。与 TNT 350 相比,TNT 250 和 TNT 450 的较低 PL 强度归因于混合锐钛矿/板钛矿结构,有利于电子从锐钛矿转移到板钛矿 [47]。与其他 TNT 样品相比,TNT 550 的强度还表明电子-空穴对的电荷分离增加和寿命更长。安东尼等人。 [34] 还报告了金红石/锐钛矿 TNT 的最低 PL 强度。然而,光致电荷载流子寿命高度依赖于金红石与锐钛矿的比率[48]。 468 到 700 nm 之间的发射带归因于 TiO2 的表面缺陷 [17]。所制备的 TNT 中的典型缺陷是 Ti 3+ 位点、氧空位和 Ti 4+ 的部分配位 [49, 50]。 Ti 4+ 的部分配位 可由阴离子杂质 (Cl − ) 源自制备过程 [51]。从 XPS 获得的结果(图 6)显示所有 TNT 样品中都存在氯污染;然而,杂质的浓度在退火时降低。这些杂质也有助于促进复合的晶体缺陷 [51]。 445 nm (2.79 eV) 和 484 nm (2.56 eV) 附近的发射带最大值可归因于扭曲八面体 TiO6 的表面状态 (O-Ti-OH) [34]。 539 nm (2.30 eV) [17] 和 527 nm (2.36 eV) [52] 附近的发射带与二氧化钛结构中的氧空位有关。 517 nm (2.40 eV) 附近的发射带与 Ti 4+ 相关 氧空位附近的离子[53]。
<图片>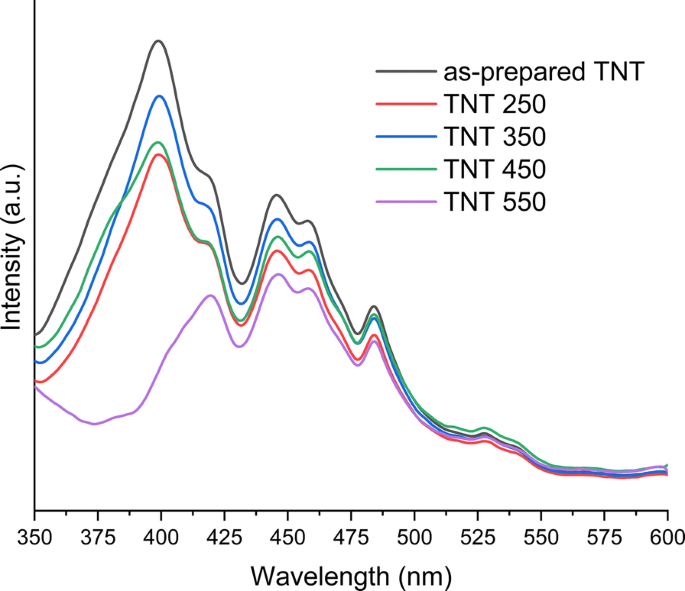
制备和退火的TNTs的光致发光发射光谱
图>图 8b 显示了 TNT 电极在 300-400 nm 辐照波长下的入射光子电流效率 (IPCE) 特性。 IPCE 值由下式计算
$$ \mathrm{IPCE}\%=\frac{1240\times {J}_{\mathrm{ph}}}{\lambda \times {I}_{\mathrm{light}}} $$ (2)其中 J ph 是测得的光电流密度 (mA cm −2 ), λ 是入射辐射波长,I light 是光源的强度 (mW cm −2 ) 在特定波长 (nm)。在辐照时获得 TNT 350、TNT 450 和 TNT 550 的光电流。在混合锐钛矿/板钛矿结构的情况下,TNT 450 获得了最高的光电流和 IPCE 值。板钛矿的负导带比锐钛矿多 0.14 eV,这有助于电子从板钛矿转移到锐钛矿 [47, 54]。 TNT 450(锐钛矿/板钛矿)的IPCE值比TNT 350(锐钛矿)高1.37倍,比TNT 550(锐钛矿/金红石)高3.95倍。与 TNT 350(锐钛矿)相比,TNT 550(锐钛矿/金红石)具有更小的光电流,这与之前的研究结果非常吻合 [49, 55]。先前的研究表明,高金红石/锐钛矿相含量会降低光电流效率 [56] 和光催化活性 [57]。这与我们的发现非常吻合,因为 TNT 550 由明显比锐钛矿更多的金红石组成。然而,对于所制备的和 TNT 250 样品,无法获得光电流。光电流受到样品中存在的陷阱态的限制 [49,50,51]。之前的研究报告了在 450°C 下退火的 TNT 中出现光电流 [2, 55, 58] 或来自所制备的 TNT 阵列的光电流很小 [55]。然而,在这些情况下,所制备的 TNT 阵列是无定形的。在本文中,对于在等于或高于 350°C 的温度下退火的 TNT 样品获得光电流。由于纳米管束的不均匀分布,与排列良好的 TNT 阵列相比,RBA TNT 的光电流效率通常较低 [59]。差异可能是由于电极制备造成的,可能会在 TNT 中留下乙醇残留物,或者是由于使用 Nafion 将粉末粘附到 FTO 玻璃上而产生的。 TNT 电极用于电化学阻抗谱 (EIS),结果和分析见附加文件 1:图 S3。
<图片>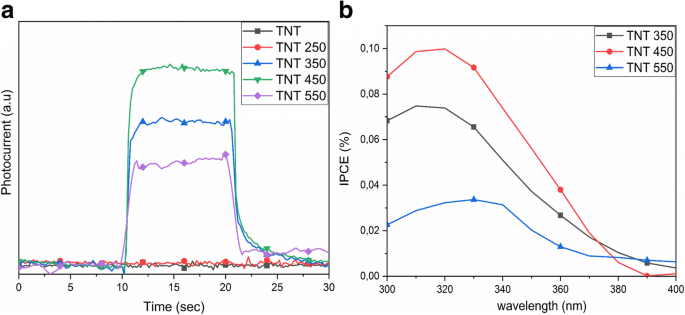
一 在 320 nm 的照射波长下获得的光电流。 b TNT 350、450和550的IPCE值
图>TNT 的光催化脱色
通过在紫外光和自然日光照射下,阴离子 MO 和阳离子 RhB 染料在水溶液中的光催化脱色来评估 TNT 粉末的吸附和光催化性能。光催化降解的基本机制涉及在照射下电子-空穴对的光生。如果照射波长大于材料的带隙,则电子从价带提升到导带,产生电子-空穴对 [26]。光生电子-空穴对与水反应形成活性羟基(OH·)和超氧自由基(O· − ),它们与有机化合物相互作用,将它们分解为 CO2 和水 [25]。附加文件 1:图 S4 中提出了单相降解机制的示意图。 The mixed crystal structure of anatase/brookite (TNT 450) and anatase/rutile (TNT 550) with a phase-junction allows the mobility of electrons to flow from brookite to anatase [54] and from rutile to anatase [57]. This allows low electron-hole recombination upon excitation, and a schematic illustration is provided in Additional file 1:Figure S5. The adsorption capacity of the samples was examined prior to irradiation by keeping the samples under dark conditions until adsorption-desorption equilibrium was reached, i.e., within 3 h. The adsorption is shown in Fig. 9 as the time before irradiation begun. The largest adsorption of ca 28% was found for MO on the as-prepared TNT powder. All other samples adsorbed 15% or less of dye for both MO and RhB (Fig. 9).
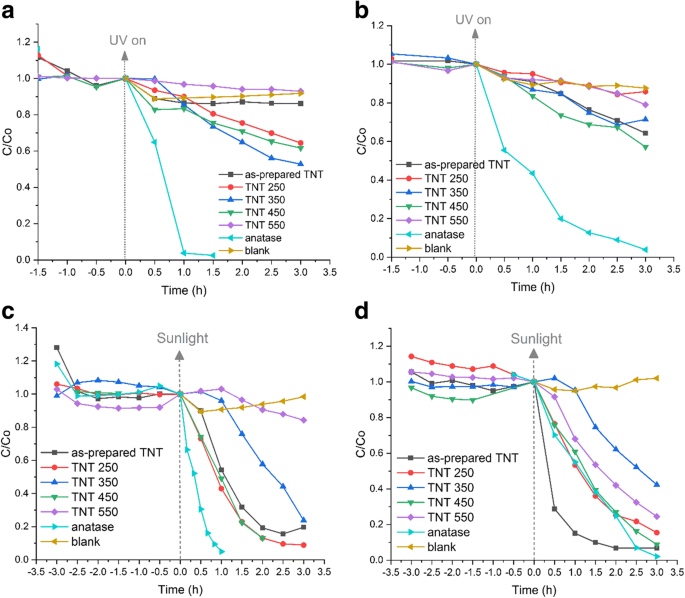
Photocatalytic decolorization of a MO and b RhB under UV light irradiation and c MO, and d RhB under sunlight irradiation for 3 h using the TNT powders
图>Control experiments (blank tests) were performed for both dyes without a catalyst under UV light and sunlight irradiation. They showed a slight decrease in dye concentration under UV light:RhB concentration decreased by 14% and MO by 8%. The blank tests under sunlight displayed a 2% variation in concentration, which is within the margin of error of the measurement.
Figure 9a–b displays the decolorization of MO and RhB under UV light irradiation. The anatase reference powder decolorized both dye solutions within 3 h, whereas the best TNT powder for MO under UV light was TNT 350 with 47% of MO decolorized. TNT 250 and TNT 450 performed quite similarly with 36 and 38% of MO decolorized, respectively. For RhB, TNT 450 performed most effectively of the TNT powders with 43% of RhB decolorized within 3 h, while the as-prepared TNT powder was next with 36% of RhB decolorized.
The decolorization of MO and RhB under natural sunlight using RBA TNT catalysts are presented in Fig. 9c–d. It is notable that MO was completely decolorized by the as-prepared TNT, TNT 250, and the reference anatase powder within the detection limit of 1.0 mg L −1 . TNT 450 decolorized MO up to 87% in 2 h. The fastest decolorization of RhB was observed using the as-prepared TNT, whereas the decolorization using TNT 250, TNT 450, and anatase reference powder was approximately equal. It is clear that the TNT powders perform significantly better under natural sunlight as compared to UV light.
The data were fitted to the pseudo-first-order kinetic equation, which is expressed as ln(C/C0) = −κ 1t , where κ 1 is the first-order rate constant. By plotting ln(C/C0) against time (t ), a straight line of which the slope equals the rate constant is obtained. All the determined rate constants and correlation factors (R 2 ) are given in Table 2, and the fitting is shown in Fig. 10. The highest rate constants are found for the anatase reference powder under UV light, i.e., 2.78 h −1 for MO and 1.05 h −1 for RhB. For the TNT powders, the highest κ 1 is found for TNT 350 (0.24 h −1 ) in MO and for TNT 450 (0.18 h −1 ) in RhB under UV light. The anatase reference powder displayed the highest rate constant at 3.02 h −1 in MO under sunlight, while TNT 450 yielded a κ 1 of 1.05 h −1 under the same conditions. However, the as-prepared TNT in RhB performed better (rate constant of 1.29 h −1 ; R 2 = 0.93) than the reference anatase powder (rate constant of 1.22 h −1 ; R 2 = 0.89). The high photocatalytic activity of reference anatase powder in sunlight is ascribed to the UV light in the natural sunlight spectrum since the results are analogous under both UV and sunlight. The as-prepared TNT and TNT 450 have the two highest decolorization rates of the TNT powders for RhB; TNT 450 has the highest rate under UV light although the difference between the as-prepared TNT (0.15 h −1 ) and TNT 450 (0.18 h −1 ) is negligible. The as-prepared TNTs are the most efficient catalyst for RhB under sunlight.
图>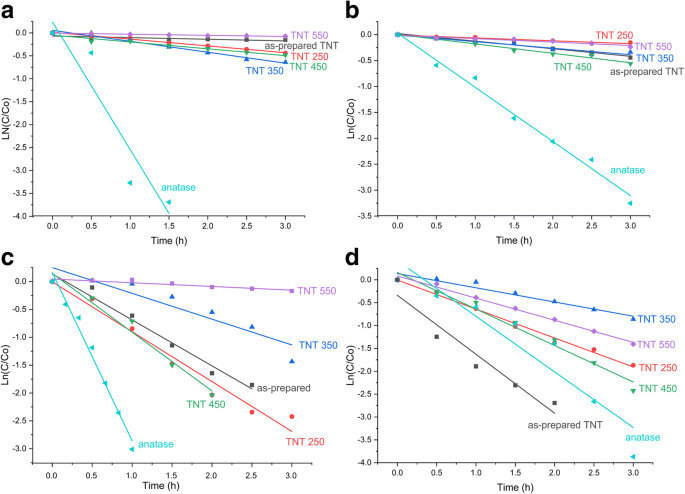
Pseudo-first-order plots of the decolorization of a MO and b RhB under UV light irradiation, and c MO and d RhB under natural sunlight irradiation
图>The main differences between the as-prepared TNT and TNT 450 powders are in their structure, specific surface area, the atomic concentration of chloride impurity, and electron-hole lifetime. The as-prepared TNTs are tubular consisting of anatase phase with the high specific surface area of 179 m 2 g −1 , while the TNT 450 are rods consisting of anatase and brookite phases with a specific surface area of 70 m 2 g −1 . The PL and IPCE results suggest better conductivity of TNT 450 as compared to as-prepared TNTs. The absorption spectra in Fig. 11 show that there is a clear difference in the photocatalytic mechanism between the as-prepared TNTs under sunlight (Fig. 11a) and UV light irradiation (Fig. 11b). Also, the degradation processes of RhB for TNT 450 (Fig. 11c) are clearly different from as-prepared TNTs. No peak shift (λ max = 554 nm) is observed under UV light, only a decrease in absorbance for the RhB peak. Two types of changes are observed under sunlight:First, the absorbance for RhB decreases, and second, the peak shifts to 498 nm. The peak at 498 nm is identified as Rhodamine, which is an N-de-ethylation product of RhB [60, 61]. N-de-ethylation only takes place under visible light, in this case, the visible light spectrum from the solar light irradiation, due to the excited RhB molecules [62]. The adsorbed and excited RhB molecules then transfer the excited electron to the conduction band of the TNT powder. This step also confirms the degradation of RhB to Rhodamine by N-de-ethylation and further to smaller components [62,63,64]. No other catalyst displayed Rhodamine as an intermediate product during decolorization of RhB. The N-de-ethylation reaction is dependent on the formation of OOH · and OH · , which is suggested to be more prominent in the reaction of the as-prepared TNTs with RhB. The as-prepared TNT carries more –OH functional groups on its surface (Fig. 5) and results in excellent dispersion of the as-prepared powder in the aqueous solution [62]. The N-de-ethylation only takes place on as-prepared TNTs in our case, similar to the study by Guo et al. [65], where they only observed N-de-ethylation on their titanate nanotubes. The justification for this phenomenon may be that nanorods have a lower adsorption capacity associated with the reduction of the specific surface area upon annealing [65]. The decolorization reaction of RhB using TiO2 materials under UV light is different and requires the formation of RhB +· and the presence of air [65]. The mechanism has been widely studied elsewhere [65].
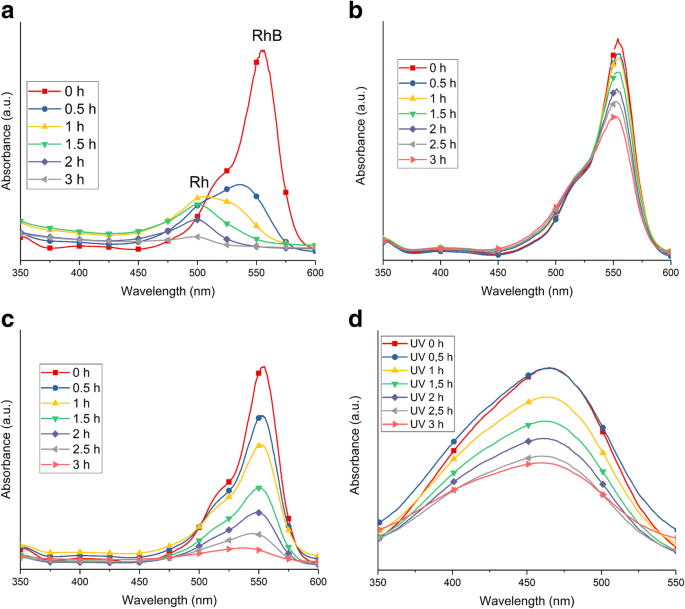
UV-Vis absorbance spectra of the decolorization of RhB using a the as-prepared TNT powder under sunlight, b the as-prepared TNT powder under UV light, c the TNT 450 powder under sunlight and the decolorization of MO by d TNT 350
图>The photocatalytic decolorization of MO using the TNT powders presents a different trend between the samples of which one has an increased decolorization effect. Under UV light, TNT 350 presented the most prominent kinetic rate of 0.24 h −1 , whereas for sunlight, the most prominent rate was found for TNT 450 with 1.05 h −1 ; however, the TiO2 anatase reference powder was superior in both cases. Despite the comparatively small specific surface area, TNT 450 with the mixed crystal structure (anatase/brookite) exhibited the best photocatalytic efficiency of the prepared TNTs. This is attributed to the improved electron-hole separation, apparent from high photocurrent obtained from TNT 450 in Fig. 8. This result is consistent with published reports [37, 47]. The adsorption spectra from the MO show only the decrease in absorbance with irradiation time (Fig. 11d). No blueshift is observed for the decolorization of MO for any TNT catalyst, suggesting the damage to the conjugated system made up of azo groups and aryl rings of MO dye [65].
Titanate nanotubes with different morphology and crystal structure have been tested under simulated solar light and have reached almost complete degradation of MO and RhB [65]. RBA TNT powders as photocatalysts have been investigated under UV light using MO [20] and acid orange 7 (AO7) dye [21]. Liao et al. [20] reported a reaction rate of 0.082 min −1 (4.92 h −1 ) which is significantly higher than the rates obtained in this study under UV light. However, it is noteworthy that the post-treatment destroyed the tubular structure of the TNTs into particles (Liao et al. [20]). Hahn et al. [21] reported decolorization rates for doped TNT layers up to 0.469 h −1 (undoped TiO2 0.385 h −1 ) using AO7 as a model dye under UV light irradiation. It is crucial to use a catalyst that can utilize the extended sunlight spectrum for efficient degradation of pollutants. RBA TNT powders prepared under different washing conditions and annealed at 500 °C, with a pure rutile phase have been reported as an efficient photocatalyst for RhB degradation under visible light illumination [33]. In the present report, the study of photocatalytic properties of TNT powders prepared by RBA is extended from UV to visible light range under sunlight irradiation, contrary to the general perception of titania only being active under UV light.
结论
The TNT powders were prepared by rapid breakdown anodization. By annealing the TNT powders at 250–550 °C, the bandgap narrowed from 3.04 eV down to 2.88 eV, and the tubes transformed from anatase phase to anatase/brookite and further to anatase/rutile phase mixtures. The tubes transformed to nanorods at 350 °C, reducing the specific surface area from 179 to 35 m 2 g −1 . The XPS results show the characteristic peaks of Ti2p and O1s and Cl2p in all TNTs; however, the atomic concentration of chlorine decreases upon annealing. The PL results for TNT 250 and TNT 450 suggest lower electron-hole recombination as compared to as-prepared TNT and TNT 350. The higher recombination in the as-prepared TNTs is attributed to the low crystallinity and the number of surface defects. The photocatalytic activity of the TNT powders was investigated by decolorization of MO and RhB dyes under UV and natural sunlight. The photocatalytic decolorization of both dyes improved under natural sunlight, contradicting the general perception of titania nanotubes being inefficient photocatalysts under visible light irradiation. The as-prepared TNTs, TNT 250, and TNT 450 performed the best of the TNT powder samples under natural sunlight using RhB and MO as model pollutants, whereas the as-prepared TNT powder outperformed the reference TiO2 anatase powder when using RhB as a model pollutant. This could be attributed to the higher specific surface area and different photocatalytic degradation mechanism of RhB on the as-prepared TNT. TNT 250 and TNT 450 displayed similar activity under sunlight irradiation, which is ascribed to the large surface area and reaction sites for TNT 250. The specific surface area is reduced for TNT 450, and the better photocatalytic activity is attributed to a favorable crystal structure and less electron-hole recombination. In addition, the highest IPCE values are obtained for TNT 450. These RBA TNTs may intensify the use of the natural sunlight spectrum for removal of organic contaminants from polluted waters.
缩写
- DRS:
-
Diffuse reflectance spectroscopy
- EIS:
-
电化学阻抗谱
- FTIR:
-
Fourier transform infrared spectroscopy
- FTO:
-
Fluorine-doped tin oxide
- IPCE:
-
Incident photon to current efficiency
- MO:
-
Methyl orange
- PL:
-
光致发光
- RBA:
-
Rapid breakdown anodization
- RhB:
-
罗丹明B
- SEM:
-
扫描电镜
- TEM:
-
透射电子显微镜
- TNTs:
-
Titania nanotubes
- XPS:
-
X射线光电子能谱
- XRD:
-
X射线衍射
纳米材料
- 太阳能电池的纳米异质结
- 用于灵敏快速检测卵巢癌细胞的柔性石墨烯生物传感器的演示
- 有机太阳能电池中纳米孔型和纳米柱型图案化金属电极的比较
- 用于最大太阳能收集的高效且有效的 InP 纳米线设计
- 凹凸棒石-TiO2-Ag3PO4 三元纳米复合材料在模拟太阳辐射下降解罗丹明 B 的光催化活性
- 形态和晶体结构对二氧化钛纳米管热导率的影响
- 纳米粒子等离子体增强有机太阳能电池的设计原则
- 使用双面金字塔光栅进行薄膜硅太阳能电池的有效光吸收
- 电化学自组织二氧化钛纳米管阵列综述:合成、修饰和生物医学应用
- Mg 掺杂对用于增强光催化评估和抗菌分析的 ZnO 纳米颗粒的影响
- 一种用于光伏器件的具有抗反射和超疏水特性的新型纳米锥簇微结构
- 纳米杂化物的材料、异质结构和取向对光催化活性的影响