用于肿瘤靶向成像和治疗的多功能金纳米粒子的一步、快速和绿色合成
摘要
金纳米粒子 (GNP) 一直被用作阿霉素 (DOX) 转运载体,用于肿瘤诊断和治疗;然而,这些载体的合成过程是先通过化学还原法制备GNPs,然后再与DOX或特定肽结合,因此这些方法面临着多步骤、成本高、耗时、制备复杂等共同问题。后期处理。在这里,我们首次提出了一种基于 DOX 化学组成制备 DOX 共轭 GNP 的一步策略。此外,我们借助 DOX、RGD 肽和核定位肽 (NLS) 具有的还原性官能团,一步法制备多功能 GNP(DRN-GNP),仅需 30 分钟。散射图像和细胞 TEM 研究结果表明 DRN-GNPs 可以靶向 Hela 细胞的细胞核。裸鼠肿瘤和尾静脉注射DRN-GNPs的抑瘤率分别为66.7%和57.7%,与对照组相比显着提高。多功能GNP的一步合成不仅节省时间、材料,而且符合绿色化学的发展方向,为不久的将来大规模应用奠定基础。我们的结果表明制备策略是有效的,我们制备的 DRN-GNPs 在生理系统中具有良好的胶体稳定性;它们是宫颈癌诊断和治疗的潜在造影剂和有效的DOX转运载体。
介绍
多柔比星(Doxorubicin, DOX)是一种常用的抗癌药物,已广泛应用于多种癌症化疗,如血液恶性肿瘤[1]、多种腺癌[2,3,4,5]、软组织肉瘤[6]。 ], 等等。但长期、高剂量服用DOX会导致耐药[7]、恶心[8]、脱发[9]、急慢性毒性[10],可能导致充血性心力衰竭[11]。因此,开发一种具有良好生物相容性和高载药能力的药物载体是非常必要的。近年来,大量纳米材料,如量子点[12、13]、壳聚糖[14、15]、硅纳米材料[16、17]、高分子纳米材料[18、19、20]、荧光纳米粒子[21、22、 23,24] 和金属纳米材料 [25,26,27,28,29,30] 被开发为用于肿瘤诊断和治疗的 DOX 转运载体。在这些纳米材料中,金纳米材料因其独特的化学和光学性质、低毒性、良好的生物相容性以及表面改性的控制能力而被广泛应用[31, 32]。到目前为止,将 DOX 结合到 GNP 上有三种方法。第一种是借助肼 [25]、1-乙基-3-[3-二甲氨基丙基]碳二亚胺盐酸盐 (EDC) [26]、pH 敏感剂 [27]、DCC/ NHS 系统 [28]。第二种是将 GNP 与 DOX 孵育至少 24 小时 [29]。第三种是用 DOX [30] 代替结合在金纳米粒子 (GNP) 表面上的柠檬酸盐。尽管这些 DOX 偶联的 GNP 已用于肿瘤治疗,但由于缺乏足够的特异性,其应用仍然受到限制。最近,El-sayed 的 [27] 将 GNP 与 DOX、RGD 肽和核定位信号 (NLS) 肽功能化。他们工作的亮点在于GNPs通过受体介导的RGD肽的内吞作用进入肿瘤细胞,然后借助NLS肽进入细胞核,这使得DOX有效干扰DNA合成。不幸的是,通过在 GNP 上逐层偶联肽或药物至少需要 120 小时,每个过程至少需要 24 小时。同样,上述所有方法都面临着步骤多、成本高、制备复杂和后处理等常见问题。因此,有必要开发一种简便、快速的多功能金纳米材料的合成方法。
在本文中,我们首次提出了一种基于 DOX 化学组成制备 DOX 共轭 GNP 的一步策略。此外,我们提出了一种方便的合成方法来制备多功能 DOX 转运载体,使 DOX 更好地发挥作用,可用于肿瘤诊断和治疗。我们与其他人的工作之间的主要区别在于,我们使用 DOX、RGD 肽和 NLS 肽作为还原剂和稳定剂来制造 GNP,并使所有这三种物质在 GNP 表面共轭形成 DRN-GNP同时。我们之前的工作 [33,34,35] 已经证明 RGD 和其他肽可用于还原金离子以制造金纳米材料。 NLS的N端可以通过使用半胱氨酸半胱氨酸酪氨酸(CCY)序列构建CCYNLS进行修饰,在减少金离子的同时仍然保持NLS的靶向作用[36]。我们还发现 DOX 具有两个酚羟基,在碱性条件下具有很强的还原性 [37, 38],因此它也可以还原金离子以形成 GNP。此外,DOX 的抗肿瘤作用可能不会受到影响,因为它通过碳 7 的氨基和碳 9 的羟基嵌入 DNA [39]。同时,肽和 DOX 上的硫、氧和氮可以与 GNP 结合以保持胶体稳定 [33]。结果表明,DRN-GNP 已成功制备,并且在肿瘤成像、诊断和治疗方面效果良好(方案 1)。据我们所知,这是首次通过一步法合成DOX、RGD和NLS肽缀合的多功能GNP及其在肿瘤诊断和治疗中的应用。
<图片>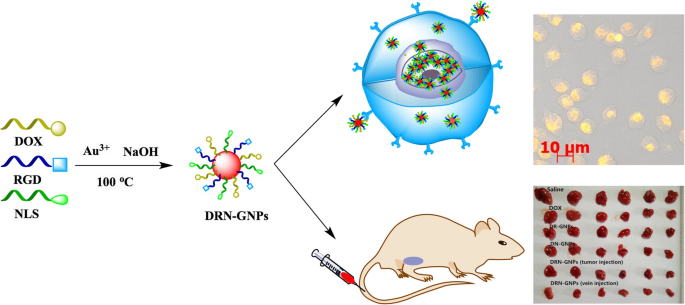
DRN-GNPs的合成、Hela细胞摄取及DRN-GNPs的肿瘤诊断示意图
图>材料和方法
材料
HAuCl4·4H2O购自国药集团化学试剂有限公司,环状RGD肽(氨基酸序列为精氨酸-甘氨酸-天冬氨酸-酪氨酸-谷氨酸(c(RGDyE)))和氢氧化钠与参考文献相同。 [35]。多柔比星购自生工生物技术(上海)有限公司。 CCYNLS肽(氨基酸序列为半胱氨酸-半胱氨酸-酪氨酸-脯氨酸-脯氨酸-赖氨酸-赖氨酸-赖氨酸-精氨酸-赖氨酸-缬氨酸,CCYPPKKKRKV)提供中国多肽有限公司(中国上海)。 Hela细胞系和MCF-7细胞系由广州友迪生物科技有限公司提供。胎牛血清(FBS)和Dulbecco改良Eagle培养基(DMEM)购自GibcoBRL Life Technologies。增殖和细胞毒性检测试剂盒(MTT)购自北京鼎果长盛生物科技有限公司
DOX-GNP、NLS-GNP、DR-GNP、DN-GNP、DRN-GNP 的合成
为了制备 DOX-GNP,在磁力搅拌下将 DOX 溶液(0.2 mg/mL,1.0 mL)加入到氯金酸溶液(0.86 mM,1.0 mL)中,然后加入 NaOH(2 M,5 μL)到调节pH值。为了制备 NLS-GNPs,在磁力搅拌下将 CCYNLS 肽溶液(0.5 mM,1.0 mL)加入氯金酸溶液(3.44 mM,1.0 mL)中,然后加入 NaOH(2 M,20 μL)调节 pH .为了制备 DR-GNP,在磁力搅拌下将 DOX 溶液(0.2 mg/mL,1.0 mL)和 RGD 肽溶液(1.0 mM,1.0 mL)加入氯金酸溶液(1.72 mM,2.0 mL)中,然后加入 NaOH加入 (2 M, 15 μL) 以调节 pH。为了制备 DN-GNP,在磁力搅拌下将 DOX 溶液(0.2 mg/mL,1.0 mL)和 CCYNLS 肽溶液(0.5 mM,1.0 mL)加入氯金酸溶液(1.72 mM,2.0 mL)中,然后加入 NaOH加入 (2 M, 20 μL) 以调节 pH。为了制备 DRN-GNP,将 DOX (0.2 mg/mL, 1.0 mL)、CCYNLS 肽 (0.5 mM, 1.0 mL) 和 RGD 肽 (1.0 mM, 1.0 mL) 的溶液加入氯金酸溶液 (1.72 mM, 3.0 mL) 在磁力搅拌下,然后加入 NaOH (2 M, 35 μL) 以调节 pH。所有反应在回流系统中在 100°C 下持续 30 分钟,pH 值为 10-12。通过以 55,000 rpm 离心 30 分钟(Optima MAX-TL,Beckman Coulter)来纯化所有 GNP。去除上清液后,将GNPs重新分散在超纯水中。
特征化
GNP 光谱的表征是通过使用 UV 3150 分光光度计(日本岛津)、Nicolet Avatar FTIR 330 型光谱仪(Thermo,美国)和 ESCALab250 X 射线光电子能谱(XPS)在相同的分析条件下进行的作为参考。 [35]。 GNPs的透射电子显微镜(TEM)图像是在200 kV加速电压下运行的TEM(JEM-2100F,JEOL,Japan)获得的。
动态光散射和 Zeta 电位测量
Zeta PALS Zeta 电位分析仪(Brookhaven Instrument Corporation)用于测量 DOX-GNP、NLS-GNP、DR-GNP、DN-GNP 和 DRN-GNP 的动态光散射 (DLS),其在 90°下工作在室温下与固态激光器 (λ =670 nm) 的角度。当配备 AQ-827 电极时,该分析仪用于测量 GNP 的 zeta 电位。
ICP分析
GNP中Au的定量分析与参考文献相同。 [35].
分散在 PBS、HCl、NaOH、NaCl 溶液中的 DR-GNP、DN-GNP 和 DRN-GNP 的稳定性
我们分别混合了 100 μL 沉淀的 GNP 和 500 μL 高浓度的 0.2 M PBS、0.5 M HCl、0.5 M NaOH 和 1.0 M NaCl 溶液。 12小时后,我们拍下了他们的照片并测试了他们的紫外吸收光谱。
细胞培养
Hela 和 MCF-7 细胞在培养箱中(用 5% CO2 平衡空气加湿)在 37°C 下培养 24 小时。培养基为含 10% FBS 的 DMEM。活细胞数由细胞计数板计算。
游离 DOX 和 DRN-GNP 的细胞毒性分析
将指数期的 Hela 和 MCF-7 细胞加入 96 孔板(约 5 × 10 3 细胞/孔)并分别孵育 24 小时。然后将浓度为 0、20、40、60、80 和 100 μg/mL(相应的 DOX 浓度为 0.0、7.8、15.6、23.4、31.2 和 39.0 μg/mL)的 DRN-GNP 与每个孔一起孵育,并且分别在 37°C 孵育 24 小时。对照组仅加入PBS缓冲液,生存力设为100%。每孔加入 MTT(20 μL,5 mg/mL),37 °C 孵育约 4 h。然后小心吸出培养基,每孔加入150 μL二甲亚砜10 min,直至晶体完全溶解。使用酶标仪 (Thermo Multiskan Mk3) 在 490 nm 处测量每个孔的 OD 值。每组设3孔,计算值以平均值±标准差表示
流式细胞术实验
将指数期的 Hela 和 MCF-7 细胞分别加入两块 12 孔板(约 5 × 10 4 细胞/孔)并分别培养 24 小时。然后,将 DRN-GNPs 溶液加入每个孔中(终浓度为 0、20、40、60、80 和 100 μg/mL),并分别在 37°C 下孵育 24 小时。去除培养基并将胰蛋白酶(含 EDTA)溶液加入孔中以消化细胞。然后从孔中取出胰蛋白酶溶液,在孔中加入培养基,并将细胞转移到离心管中。 1000 g 离心 5 min 后,除去上清液,收集每管中的细胞并在 PBS 溶液中计数。大约 5–10 × 10 4 的细胞被放入另一个离心管中,并再次以 1000 g 离心 5 分钟。去除上清液,向管中加入 100 μL AnnexinV 缓冲溶液。将 5 μL 的 AnnexinV-FITC 和 5 μL 的碘化丙啶加入管中,轻轻地与细胞混合。试管在室温(20-25°C)下避光孵育15分钟,然后用流式细胞仪(BD Accuri C6)检测。
细胞培养的光学暗场散射成像和透射电子显微镜研究
将Hela和MCF-7细胞植入8孔细胞培养玻片约7×10 3 每个孔的细胞,分别。将 DRN-GNPs 添加到每个孔中,终浓度为 35 μg/mL。孵育 24 小时后,我们通过用 PBS 溶液冲洗这些细胞 3 次来去除物理和游离吸收的 GNP。然后,我们用 4% 多聚甲醛固定 20 分钟,涂上几滴甘油,并用另一个盖玻片密封这些 8 孔细胞培养玻片。使用暗视野显微镜(Zeiss Imager Z1)在× 200 放大倍率和反射模式下评估细胞。 5 V电压下明场和暗场图像的曝光时间分别为1 ms和400 ms。
对于 TEM 研究,Hela 和 MCF-7 细胞分别与 DRN-GNP (35 μg/mL) 在细胞培养瓶中孵育 24 小时。培养并吸出培养基和DRN-GNPs的混合溶液后,我们用PBS溶液冲洗细胞3次,然后用胰蛋白酶消化。将细胞转移到含有少量 DMEM 培养基的离心管中。将细胞离心(1500 rpm)10 min,小心吸出DMEM培养基,小心加入3%戊二醛溶液(通常为材料体积的40倍),将细胞固定在管底。管 1 小时以上。通过加入 1% 四氧化锇 1 小时进一步固定细胞,用乙醇脱水,然后包埋在 Spurr(Sigma-Aldrich Co.,USA)中。将细胞从试管中取出,切片,用醋酸双氧铀和柠檬酸铅水溶液染色,然后固定在铜网格(300目)上。采用FEI TECNAI-12透射电子显微镜(工作电压120 kV)对试样进行测量。
动物实验
动物主题
无胸腺雌性裸鼠购自南方医科大学动物实验中心(中国广州)用于体内 肿瘤治疗试验。所有动物实验均按照中华人民共和国卫生部动物管理条例(2001年第55号文件)和中国药科大学实验动物护理和使用指南进行。>
游离 DOX 及其结合物对荷瘤小鼠的治疗效果
简而言之,将 36 只裸鼠(5-6 周龄,体重 16-18 g)随机分为六组。皮下注射 Hela 细胞 (5 × 10 6 PBS 中的细胞)进入右腋窝。 5天后,对照组小鼠尾静脉注射生理盐水(0.154 mol/L,0.2 mL)。游离DOX、DR-GNPs、DN-GNPs和DRN-GNPs组小鼠也尾静脉注射。为了比较DRN-GNPs在静脉注射和肿瘤注射之间的抗肿瘤效果,我们设置了一组在肿瘤部位注射等量的DRN-GNPs。这五组在每只小鼠中维持 0.3 mg/kg 等效游离或结合 DOX 的剂量。每只小鼠每天接受一次尾静脉或肿瘤注射。在 14 天内每天监测每只小鼠的体重和肿瘤体积。为了进一步研究 DOX、DR-GNPs、DN-GNPs 和 DRN-GNPs 的治疗效果,在治疗 14 天后切除 6 组的肿瘤进行病理分析。取小鼠肿瘤及心、肝、脾、肺、肾等脏器,用10%中性福尔马林固定,石蜡包埋。切片的肿瘤组织经苏木精伊红(H&E)染色,奥林巴斯光学显微镜检查。
结果与讨论
DOX-GNP、NLS-GNP、DN-GNP、DR-GNP、DRN-GNP 的合成和表征
在DOX-GNPs的合成中,当将NaOH溶液加入到DOX与氯金酸溶液的混合溶液中时,溶液的颜色立即由橙色变为浅紫色,然后在100℃下变成酒红色。一分钟内磁力搅拌下的回流系统,表明 DOX-GNP 的形成。结果表明,DOX 的还原能力很强,因为 DOX 具有两个酚羟基(6 号和 11 号碳),在碱性条件下具有很强的还原性。反应持续30 min直至颜色不再变化。
为了避免混淆 DOX 的橙红色和 GNP 的酒红色,我们通过表征它们的紫外吸收光谱提出了更多证据(如图 1a 所示)。 DOX在485nm附近有特征吸收峰,在530nm处有峰;然而,产品溶液光谱中 480 nm 处的峰消失,而 GNP 的 520 nm 特征表面等离子体共振 (SPR) 吸收峰出现了特征峰。即便如此,在 GNPs 的 520 nm 和 DOX 峰值的 530 nm 之间仍然没有完全确定 GNPs 的形成。考虑到GNPs会在高速离心时沉淀,而DOX分子不会,我们可以看到离心管底部的产物中有紫色沉淀物(图1a(3-4)的插图)和DOX两者都没有(图 1a (1-2) 的插图)。
<图片>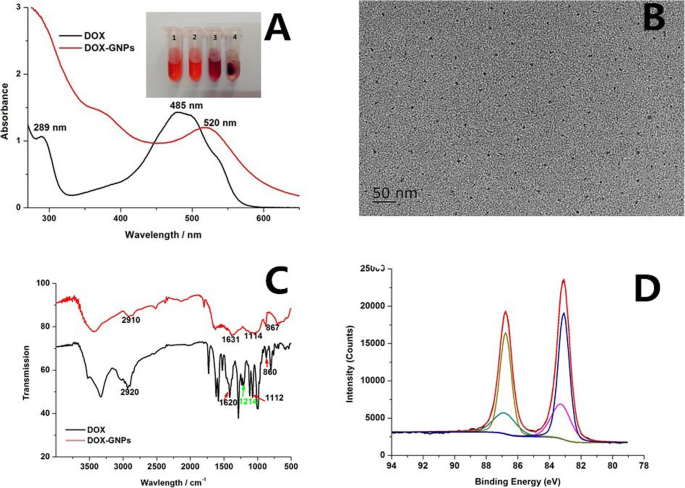
一 DOX 和制备的 DOX-GNPs 的紫外-可见吸收光谱,插图 (a ) 是离心前 (1, 3) 和离心后 (2, 4) 的 DOX (1, 2) 和 DOX-GNPs (3, 4) 的图像。 b DOX-GNP 的 TEM 图像。 c DOX 和制备的 DOX-GNP 的 FTIR 光谱。 d DOX-GNPs的XPS谱
图>DOX在365nm紫外光照射下有红色荧光;然而,GNPs 合成后 DOX 的荧光消失。有两个可能的原因;首先是 GNPs 的消光系数非常强,当它们靠近 GNPs 表面(<5 nm)时,它们往往会猝灭分子的荧光[40]。如果距离增加到 20 nm 或更多,纳米粒子的等离子体场太远而无法淬灭它们的荧光信号 [41]。另一个原因是DOX的荧光基团在起到还原剂的作用后被破坏了。如图1c所示,虽然DOX-GNPs的红外吸收峰数量明显少于DOX,但仍保留了DOX的许多特征,如2910 cm处的特征IR吸收峰 −1 (C-H, 伸缩振动) [42], 1631 cm −1 (羰基伸缩振动) [43], 1114 cm −1 (C-O, C-N 伸缩振动) [44], 和 867 cm −1 (N-H,C-H,面外弯曲振动)[45],分别。 DOX 的那些代表性峰也显示在 DOX-GNP 的光谱中,表明 DOX 成功地与 DOX-GNP 结合。有趣的是,峰值在 1214 cm −1 酚羟基 [46] 的 C-O 伸缩振动的特征峰在 DOX-GNP 的光谱中消失了。酚基可以将Au离子还原为GNPs,然后将其运输到羰基中。我们推测 DOX 的作用不受影响,因为它通过碳 7 的氨基和碳 9 的羟基嵌入 DNA [39]。
我们可以从 TEM 结果中观察到 DOX-GNP 的粒径约为 6 nm(图 1b)。从 DOX-GNP 的 XPS 光谱计算出的 Au (I) 比率为 31.93%(图 1d),该比率足以保持胶体的稳定性。然而,当DOX-GNPs的沉积物在去除上清液后高速离心分散在0.2M PBS缓冲溶液中时,该溶液的颜色变成紫黑色,并且有可见的不溶性絮凝物。这表明 DOX-GNPs 在高盐浓度环境中的稳定性不是很好。可以解释为DOX只有一个氨基而没有巯基,这不足以让DOX通过Au-S和Au-N键连接GNPs表面。因此,如果将DOX-GNPs作为造影剂和抗肿瘤材料用于肿瘤成像和治疗,还需要更多的努力。
我们用 DOX、RGD 肽和 NLS 肽作为还原剂和稳定剂合成了 DRN-GNP。 RGD肽可以特异性靶向一些过表达αvβ3整合素的肿瘤细胞,NLS肽可以特异性靶向细胞核。图 2a 显示了制备的 DOX-GNPs、NLS-GNPs、DRN-GNPs 的 UV-Vis 光谱;它们的表面等离子体吸收峰的特征是 520-530 nm [33]。从插图中还可以看出,GNP 显示出明亮的红酒色。 TEM图像(附加文件1:图S1)显示NLS-GNPs和DRN-GNPs的粒径约为10 nm和5 nm,且GNPs分布均匀,未发现明显凝聚。
<图片>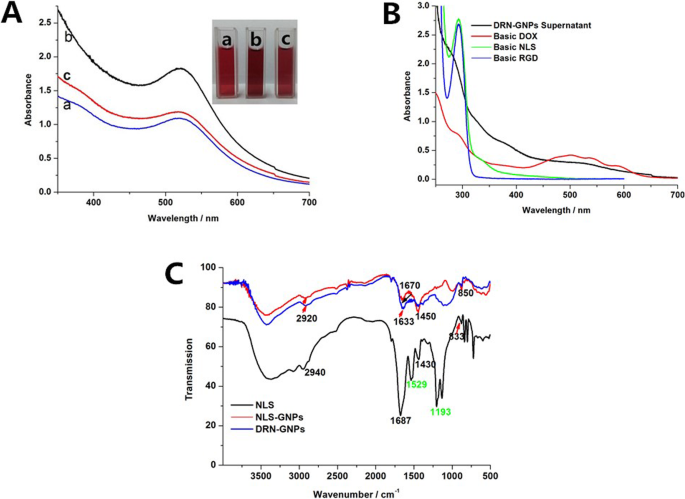
一 DOX-GNPs 的紫外-可见吸收光谱和照片图像 (a ), NLS-GNPs (b ) 和 DRN-GNP (c )。 b 碱性 DOX、RGD 肽、NLS 肽和 DRN-GNP 上清液的紫外-可见吸收光谱。 c NLS 肽、NLS-GNP 和 DRN-GNP 的 FTIR 光谱。 GNPs谱与NLS肽谱之间的距离明显放大
图>为了验证 NLS-GNP 和 DRN-GNP 的合成,我们在图 2c 中表征了 NLS 肽和 GNP 的 FTIR 光谱。在 NLS-GNPs 和 DRN-GNPs 的光谱中发现了一些 NLS 肽的 FTIR 峰,如 C-H 伸缩振动吸收峰 (2840–2972 cm -1 ) [47], C=O 伸缩振动吸收峰 (1630–1700 cm −1 ) [48], 和 =C-H 面内弯曲振动峰值 (1300–1475 cm −1 ) [49];这表明 CCYNLS 肽成功地缀合在 GNP 表面。我们还发现NLS肽中酪氨酸末端的苯骨架振动峰在1529 cm -1 和酚羟基C-O伸缩振动峰在1193 cm -1 [49] 在NLS-GNPs和DRN-GNPs的红外光谱中消失,表明酪氨酸末端的苯骨架在起到还原剂的作用后被转运为醌结构。
我们高速离心 DRN-GNP,收集几乎无色的上清液,然后通过图 2b 中的 UV-Vis 光谱测试是否有剩余的反应物。 RGD 和 NLS 肽的紫外吸收峰来源于酪氨酸残基,吸收峰位于 275 nm。然而,酚羟基在碱性条件下变成负氧离子,增加了苯环的电子密度,峰红移至292nm。我们在 DRN-GNPs 上清液的 UV-Vis 光谱中没有看到特征峰,这意味着两种肽都参与了反应。 DOX 在 450-600 nm 范围内具有三个吸收峰,但 DRN-GNP 的上清液没有这些峰。除此之外,DRN-GNPs的上清液中没有发现DOX,因为它的颜色是浅棕色,而DOX在碱性条件下的颜色是透明的浅紫色。可以得出结论,三种材料几乎完全反应。同样,DR-GNPs 和 DN-GNPs 也是这样解释的,见附加文件 1:图 S2。
我们使用 XPS 光谱来检查 Au 对 NLS-GNP 和 DRN-GNP 的化合价。如图 3a、b 所示,结合能约为 83.6 eV 和 87.0 eV 的两个峰与 Au 4f7/2 和 4f 5/2 光电子的发射一致 [33]。两个 GNP 的 Au 4f7/2 结合能位置接近 84.0 eV。对于 NLS-GNP,它可以解卷积为 Au (0) (83.5 eV) 和 Au (I) (84.1 eV),它们的峰面积比分别为 57% 和 43%。同样,对于DRN-GNPs,它可以解卷积为Au (0) (83.6 eV) 和Au (I) (84.4 eV),峰面积比分别为62%和38%,因此它们具有更高的比例Au (I)。电荷可以形成 Au (I)-S 复合物,这有助于保持 GNP 的胶体稳定性。有趣的是,我们在附加文件 1:图 S3 中的 NLS-GNP、DN-GNP 和 DRN-GNP 的 XPS 光谱中确定了三种类型的 S。蓝色和黑色曲线代表硫的氧化态,紫色曲线代表 S-H 键,绿色曲线代表 Au-S 键。 Au-S键的形成说明NLS肽成功地结合在这三种GNPs表面。
<图片>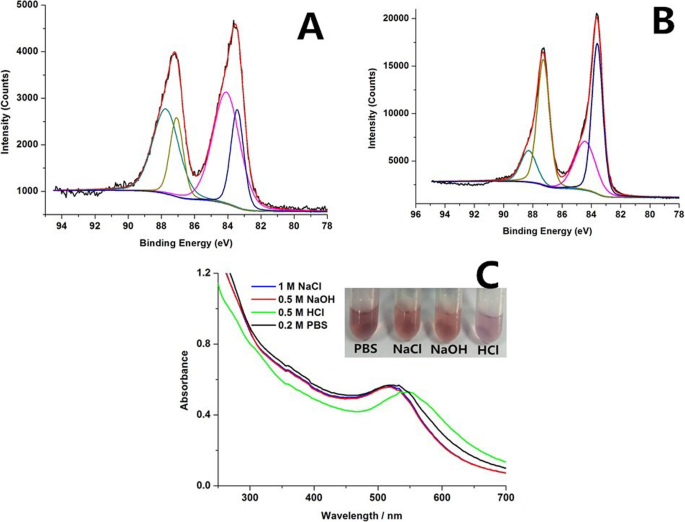
(a ) NLS-GNP,(b ) DRN-GNP。原始光谱(黑色)和拟合结果(红色)的 Au 4f7/2 结合能可以解卷积为两个分量,Au(0)-蓝色曲线和 Au(I)-紫色曲线。分散在 PBS、NaCl、NaOH 和 HCl 中的 DRN-GNPs 的紫外-可见吸收光谱和图像 (c ).
图>此外,XPS 光谱的 N 1s、C 1s 和 O 1s 峰表明肽和 DOX 附着在 GNP 上(附加文件 1:图 S4)。
XRD 谱(附加文件 1:图 S5)显示这些 GNP 的晶体结构是面心立方晶体结构,因为它们的所有 XRD 谱在 38.2°、44.5°、64.7° 和 77.8° 处都包含四个峰对应于(111)、(200)、(220)和(311)平面[33]。
DRN-GNP 的稳定性
这五个 GNP 的动态光散射 (DLS) 和 zeta 电位数据显示在表 1 中;它们的流体动力学尺寸大于 TEM 表征的尺寸,这可归因于水溶性 GNP 核心周围的一定数量的水合分子。由于 GNP 表面上共轭的羧基,它们的 zeta 电位为负。当zeta电位绝对值大于30 mV时,GNPs溶液表现出良好的胶体稳定性;绝对值越大,稳定性越好。从表1可以看出它们都具有良好的胶体稳定性。相比之下,NLS-GNPs的zeta电位值最高,这可能是因为它们的表面充满了NLS肽,可以通过硫醇基团。 DOX-GNPs的zeta电位绝对值接近30 mV;与前面提到的 DOX-GNP 分散在 PBS 缓冲溶液中时不是很稳定是一致的。光谱数据显示在附加文件1:图S6中。
图>GNP 的特征表面等离子体共振 (SPR) 带的变化可用于通过利用紫外-可见光谱法来确定 GNP 的聚集 [49]。当 DRN-GNP 分散在高盐(1 M NaCl 和 0.2 M PBS)、强酸(0.5 M HCl)和强碱中时,我们测量了它们的 UV-Vis 光谱并拍摄了它们的照片(图 3c)。 0.5 M NaOH) 溶液。除了在 HCl 溶液中,溶液颜色变化和紫外-可见光谱在各种恶劣条件下均未发生变化,颜色变为淡紫色,紫外吸收峰红移约 20 nm,这意味着 GNP 在强酸条件下轻微凝固.原因可能是带负电荷的GNPs胶体稳定性在强酸性条件下受到影响,而在中性PBS缓冲液和碱性环境中不受影响。我们可以得出结论,DRN-GNPs在高盐和高碱条件下高度稳定,表明我们合成的DRN-GNPs有望用于体外细胞成像和体内抗肿瘤治疗。
肿瘤细胞的等离子暗场散射成像和细胞 TEM 显微镜
对于DRN-GNPs肿瘤细胞的特异性靶向成像,我们选择Hela细胞作为试验组,MCF-7细胞作为对照组。原因是 Hela 细胞过表达整合素 αvβ3 [50] 而 MCF-7 细胞没有,所以它没有特异性结合 RGD;这是一个很好的对照组[51]。在暗场模型中通过直立荧光显微镜评估这两种细胞系在孵育 24 小时后对 DRN-GNP 的吸收,最终浓度为 35 μg/mL。在没有 GNP 的情况下处理的细胞不显示等离子体光散射(图 4 A-Hela 细胞、5C-MCF-7 细胞)。用 DRN-GNP 处理的 Hela 细胞的图像显示,来自 GNP 的散射信号严重集中在细胞核处(图 4b 和 5e)。然而,我们几乎看不到MCF-7细胞在与DRN-GNPs孵育后的散射光,尽管很少量的GNPs可能通过肿瘤细胞的被动增强渗透和保留效应(EPR)进入了肿瘤细胞。 effect) (Figs. 4d and 5f), the enhanced permeability and retention (EPR) effect is a unique phenomenon of solid tumors related to their anatomical and pathophysiological differences from normal tissues. For example, angiogenesis leads to high vascular density in solid tumors, large gaps exist between endothelial cells in tumor blood vessels, and tumor tissues show selective extravasation and retention of macromolecular drugs [52]. So they might not be clearly detected under the same experimental condition. The results demonstrated that the endocytosis of Hela cells via receptor-mediated endocytosis was facilitated by the RGD peptides on the GNPs’ surface. The NLS peptides could also maintain their activity to specifically targeted nucleus. So our synthesized DRN-GNPs could be a very potential contrast agent for tumor targeted imaging.
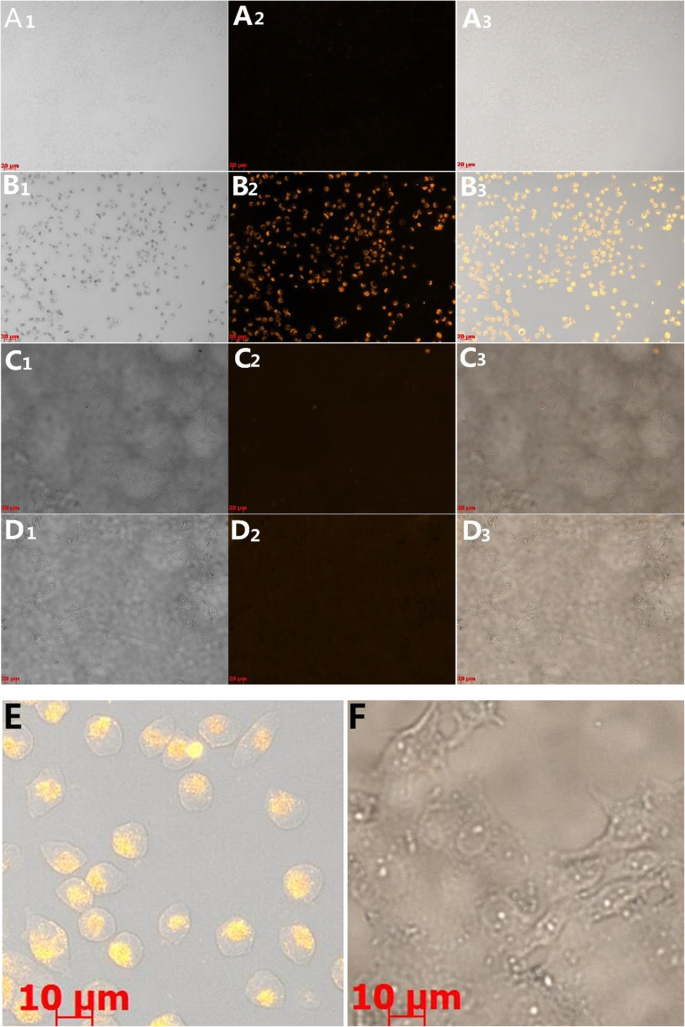
The contents of the rows are listed as follows:“PBS buffer” represents the cells incubate with PBS buffer, “DRN-GNPs” represents the cells incubate with DRN-GNPs. The subscript of “1” represents the bright field images of cells, “2” represents the dark field images of cells, and “3” represents the overlapping images (bright-field and dark-field) of cells. All of the scale bars are 20 μm. The picture of E is a small region chosen from B3 in Fig. 4, the picture of F is a small region chosen from D3 in Fig. 4, the scale bars are 10 μm
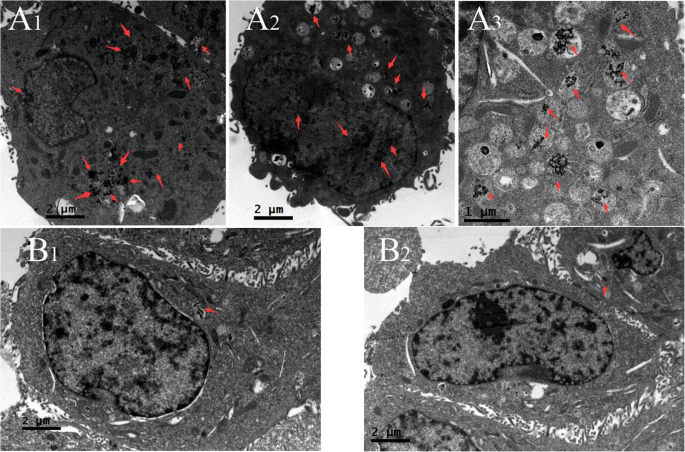
TEM images of DRN-GNPs incubated in Hela and MCF-7 cells for 24 h. A1, A2, and A3 are corresponding to HeLa cells while B1 and B2 are to MCF-7 cells
图>Table 2 shows the ratio values between the cells’ densitometric mean grey and the background in the same image, which has a positive correlation with the taken up amounts of DRN-GNPs by these two cell lines. The ratios of the cells incubated with PBS buffer and the MCF-7 cells were nearly 1.0, the brightness of cells was nearly close to the background. The ratio of Hela cells incubated with DRN-GNPs was 4.80, that means the synthesized DRN-GNPs could be specifically targeted and entered the tumor cells overexpressed the integrin αvβ3.
图>To confirm receptor-specific internalization of the DRN-GNPs, we investigated the cellular uptake of the DRN-GNPs by Hela and MCF-7 cells utilizing the TEM technique (see Fig. 5). There was a large amount of DRN-GNPs localized in the nucleus and cytoplasm regions of αvβ3-positive Hela cells (Fig. 5(A1- A3)). On the other hand, only a negligible amount of particles was found inside the cytoplasm regions of αvβ3-negative MCF-7 cells (Fig. 5(B1-B2)), they might be accumulated in the MCF-7 cells by means of the passive targeting EPR effect. We found that there were high contrast black continuous regions in the MCF-7 cells’ nucleus; they were uranyl acetate, which stained the nucleus, and they were different from the granular particles in the nucleus of Hela cells. The TEM images are consistent with the dark-field imaging results, indicating that the RGD and NLS peptides on the surface of GNPs facilitated the DRN-GNPs targeting Hela cells’ nucleus. So our synthesized DRN-GNPs could be used as a perfect contrast agent for tumor targeted imaging and diagnosis.
Cellular Therapeutic Efficacy Evaluation
To evaluate the therapeutic efficacy of DOX and DRN-GNPs at cell level, MTT assay was firstly carried out to study cell viability of Hela and MCF-7 cells under the treatment of these two samples for 24 h. DRN-GNPs demonstrated almost the same inhibition effect by killing nearly 70% of cells at the same DOX’s concentration (39.0 μg/mL, Fig. 6(A, B)). In line with the MTT results, when the concentration of DRN-GNPs was 40 μg/mL, the number and state of the Hela and MCF-7 cells were relatively good, so the concentration of 35 μg/mL was the suitable concentration for imaging. We observed that when the DRN-GNPs’ concentration was high to 60 μg/mL (Fig. 6(C3)), the Hela cells became necrosis obviously. When the concentration of DRN-GNPs goes high to 100 μg/mL, salient reduction of the number cells can be observed and clear cellular morphological change as the characteristic of necrosis was observed. The same concentration of conjugated DOX still exhibited nearly the same anti-tumor efficacy compared with the free DOX. These results indicated that our synthesized DRN-GNPs could be used not only as a contrast agent but also as a good drug delivery system.
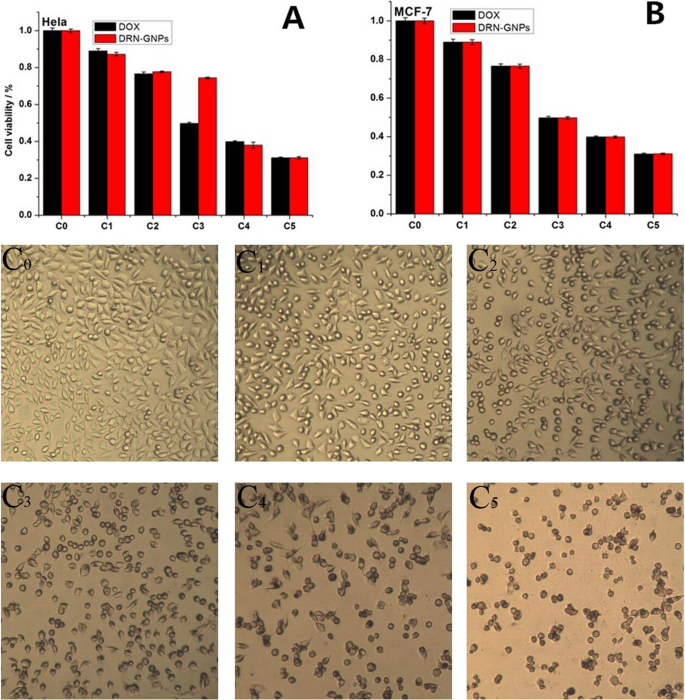
MTT assay was used to qualitatively display in vitro anti-tumor activity of DOX and DRN-GNPs on Hela (a ) and MCF-7 cells (b ) for 24 h (100%). The concentrations of C0-C5 are 0, 20, 40, 60, 80, 100 μg/mL for DRN-GNPs; the corresponding DOX’s concentrations are 0, 7.8, 15.6, 23.4, 31.2, 39.0 μg/mL. Data are represented as means ± standard deviations of triplicate samples (n =3). Images of Hela cells incubated with different concentration of DRN-GNPs (c )
图>The result detected by the flow cytometry in Fig. 7 also demonstrates the anti-tumor efficacy of DRN-GNPs. Viability of Hela cells when exposed to DRN-GNPs of different concentrations was measured by flow cytometry based assays. Two modes of cell death, apoptosis and necrosis, were measured using Annexinv-FITC and propidium iodide (PI) dyes, respectively (Fig. 7). Similar to the results of MTT assay, the data showed that DRN-GNPs induced cell necrosis was dose-dependent. The proportion of necrotic cells was 8% without incubating with DRN-GNPs; when the concentration was high to 100 μg/mL, the proportion of necrotic cells was 23.84%; that means our synthesized DRN-GNPs could kill Hela cells efficiently. The difference between the results of MTT and flow cytometry can be explained that the difference of cell number and the methods.
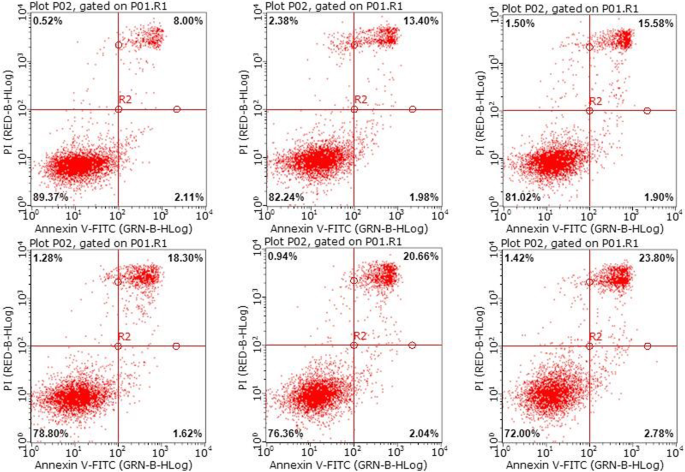
Representative two-dimensional contour density plots to determine fractions of live, apoptotic, and necrotic cells, when exposed to DRN-GNPs at different concentrations (0–100 μg/mL) for 24 h, respectively. Cell necrosis and apoptosis were measured by using PI and Annexinv-FITC dyes
图>Therapy Evaluation of DRN-GNPs in Tumor-Bearing Mice
To further determine whether DRN-GNPs induced a combined therapeutic effect on tumor cells in vivo, we evaluated the anti-tumor effect of DRN-GNPs in tumor-bearing mice for long-term intravenously injection of drugs. The saline group and the drugs of free DOX, DR-GNPs, and DN-GNPs intravenously injected groups were set as the control groups, in which the concentration of conjugated DOX was the same as that of the free DOX. We observed that the tumor sizes of DRN-GNPs-treated groups were obviously smaller than that of the saline, DOX, DN-GNPs, and DR-GNPs-treated groups (Fig. 8a). The tumor volume and body weight of the mice were monitored every 2 days. Significant variation of tumor volume and tumor weight among all of the treated groups is shown in Fig. 8d, f; the tumor volumes and tumor weight of DN-GNPs and DR-GNPs-treated groups were smaller than that of the free DOX-treated group, but still larger than that of the DRN-GNPs-treated groups because of the less targeted activity. That might be because DOX molecules are small, lack targeting, and they have a short half-life in vivo; therefore, they might be cleared out quickly. However, DRN-GNPs possess of strong stability in the blood system, long half-life, and highly targeted ability, so they can be targeted to the tumor site, and then play the anti-tumor effect of DOX. Between the DRN-GNPs intravenously injected and tumor-injected groups, the anti-tumor efficacy of the latter group is better than that of the former group because the drugs do not need a complex system of blood circulation into the focus. The tumor inhibition rate of DOX is less than 50%, and the rates of all of the DOX-conjugated GNPs are larger than 50%; they can be high to 66.7% and 57.7%, respectively. The HE stain results showed that there was a certain degree of damage for liver organs in the intravenous injected DRN-GNPs group (Fig. 9). Furthermore, the bio-toxicity of DOX, DR-GNPs, and DN-GNPs on tumor-bearing mice were also assessed by histological analysis. As shown in Fig. 8b, tumor cell volume of saline group is comparatively larger than that of treatment groups, and tumor cells demonstrate with more mitotic figures. All the results showed that the tumor inhibition effect of DRN-GNPs was the best among the GNPs. Thus, our synthesized DRN-GNPs could be used as a perfect DOX delivery system for tumor therapy.
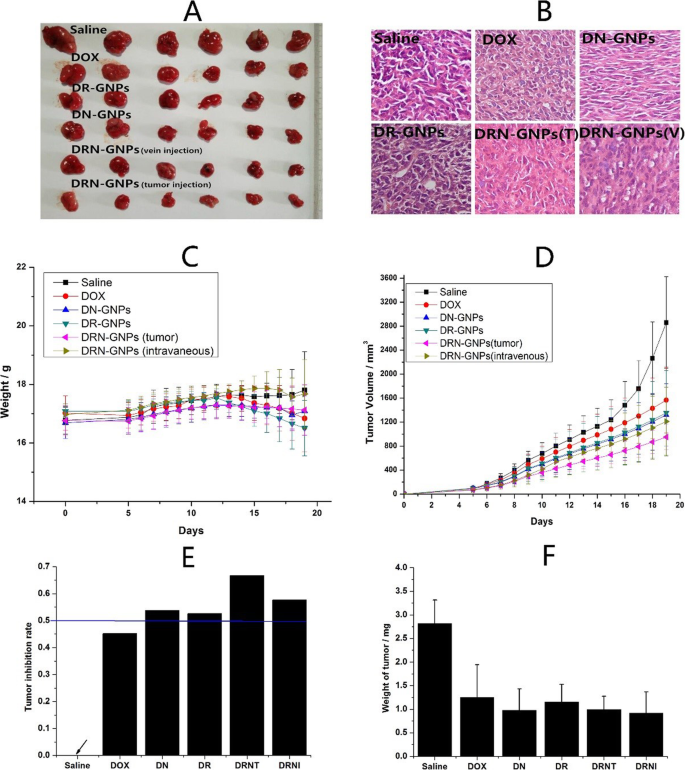
一 Tumor images isolated from tumor-bearing mice after treatment of saline, free DOX, DN-GNPs, DR-GNPs, and DRN-GNPs (tumor injection and intravenous injection). b The histological images of tumors of the mice after treated with saline, free DOX, DN-GNPs, DR-GNPs, and DRN-GNPs (tumor injection and intravenous injection). c Body weight, tumor volume (d ), tumor inhabitation rate (e ), and tumor’s weight (f ) of tumor-bearing mice during 14 days treatment; data are represented as means ± standard deviations (n =6)
图>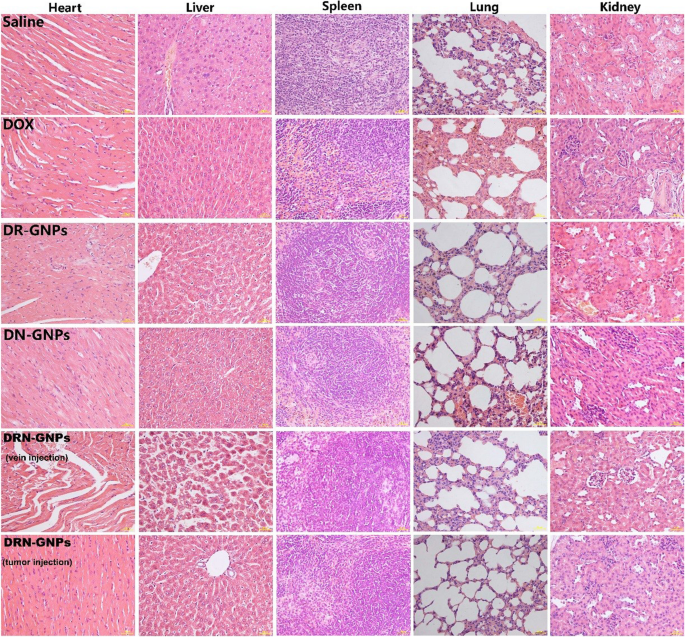
The histological images of the main organs (heart, liver, spleen, lung, and kidney) of the mice after treated with saline, free DOX, DR-GNPs, DN-GNPs, and DRN-GNPs (tumor injection and intravenous injection)
图>结论
In this study, we have successfully developed a one-step method to prepare the multifunctional DRN-GNPs in about half an hour. The GNPs have also been successfully applied to the tumor targeted imaging and tumor therapy both in vitro and in vivo. The success shows that it is possible to make a fully use of the reducing and stabilizing ability of the DOX, RGD peptides, and CCYNLS peptides, which will make the GNPs’ synthesis process simple and efficient. More importantly, the one-step synthesized DRN-GNPs still possess good colloidal stability in the physiological system, and the peptides conjugated on the surface remained the targeted ability and DOX still possesses its anti-tumor ability. To our knowledge, this is the first report for synthesis of DOX, RGD and CCYNLS peptides conjugated multifunctional GNPs with a one-step method, and their application in tumor imaging, diagnosis, and therapy. This strategy is in line with the development direction of green chemistry, and it would lay the foundation for large-scale applications within the near future. We expect that our report will provide a new way for the one-step synthesis of multifunctional nanomaterials used for tumor imaging and therapy.
数据和材料的可用性
所有数据集均在主要论文或附加支持文件中提供。
缩写
- CCYNLS peptides:
-
The sequence of amino acid is cysteine-cysteine-tyrosine-proline-proline-lysine-lysine-lysine-arginine-lysine-valine
- Cyclic RGD peptides:
-
The sequence of amino acid is arginine-glycine-aspartate-tyrosine-glutamic acid
- DOX:
-
阿霉素
- DR-GNPs:
-
DOX-RGD-GNPs, DN-GNPs:DOX-NLS-GNPs, DRN-GNPs:DOX-RGD-NLS GNPs
- GNPs:
-
金纳米粒子
纳米材料
- 用于化学传感器的金纳米粒子
- 用于改进诊断和治疗应用的多功能金纳米粒子:综述
- 用于癌症治疗的纳米粒子:当前的进展和挑战
- 用于 NIR-II 光热疗法的 BSA 涂层金纳米棒
- 用于体内 CT 成像和肾脏清除特性的新型生物相容性 Au Nanostars@PEG 纳米颗粒
- 用于细胞内蛋白质递送的二氧化硅纳米颗粒:一种使用绿色荧光蛋白的新型合成方法
- 改性超支化聚甘油作为分散剂,用于控制和稳定碳氢化合物中的金纳米粒子
- 用于光热疗法和光声成像的聚吡咯涂层铁铂纳米粒子的合成和体外性能
- 铜纳米粒子合成和稳定方面的环保能力:催化、抗菌、细胞毒性和抗氧化活性
- 桔梗皂苷(桔梗)用于金和银纳米颗粒的绿色合成
- 用于新型隐球菌诊断和光热治疗的抗体偶联二氧化硅修饰金纳米棒:体外实验
- 金属和金属氧化物纳米粒子的绿色合成及其对单细胞藻类莱茵衣藻的影响