用于整合超声成像和转移性乳腺癌协同治疗的低强度聚焦超声增强多功能纳米颗粒
摘要
乳腺癌的转移被认为对其预后有负面影响。得益于卓越的深度穿透性和无创特性,声动力疗法 (SDT) 展示了一系列导致癌症治疗的潜力。为了缓解单一疗法的局限性,已经探索了多功能纳米平台以实现协同治疗效率。在此,我们建立了一种新型多功能纳米系统,该系统将二氢卟酚 e6(Ce6,用于 SDT)、全氟戊烷(PFP,用于超声成像)和多西紫杉醇(DTX,用于化学疗法)封装在精心设计的 PLGA 核壳结构中。协同作用的 Ce6/PFP/DTX/PLGA 纳米粒子 (CPDP NPs) 具有优异的生物相容性和稳定性,主要使其进一步应用。在低强度聚焦超声 (LIFU) 照射下,增强的超声成像可以在体外和体内显示出来。更重要的是,与 LIFU 结合,纳米颗粒通过 Ce6 诱导的细胞毒性活性氧和 DTX 释放表现出有趣的抗肿瘤能力,从而产生协同的治疗效率。此外,这种治疗策略激活了强大的抗转移能力,从而显着减少了肺转移结节。结果表明,以SDT为导向的纳米平台联合化疗可作为提高有效协同治疗和抑制乳腺癌肺转移的一种有前景的方法。
介绍
作为最具威胁性的恶性肿瘤之一,乳腺癌多年来一直困扰着女性。由于高异质性和高转移能力,据报道,乳腺癌远处转移占其死亡率的 90% 以上,而晚期或转移患者的 5 年生存率仅为 26%,导致临床结果不佳 [1,2,3]。乳腺癌的阴性特征使其难以完全治愈,使得治疗策略在消除原发肿瘤和远处转移方面更具挑战性。
传统的治疗方法如手术和化疗仍被认为对治疗乳腺癌有效[4]。在所有化疗药物中,多西紫杉醇 (DTX) 在治疗转移性乳腺癌 (MBC) 和晚期乳腺癌 (ABC) 中发挥着重要作用 [5]。 DTX作为紫杉中化学物质合成的一线抗肿瘤药物,其抗肿瘤作用主要是通过破坏有丝分裂和细胞增殖来实现的[6]。由于其广泛的抗肿瘤效率,DTX 正在成为治疗乳腺癌的最有效的化疗药物之一 [7]。然而,化疗药物通常会引起不良反应和全身毒性,极大地限制了治疗效率[8]。此外,不同的临床阶段和各种个人情况表明,单一的治疗方法可能不足以满足乳腺癌治疗的所有期望。因此,在未来的应用需求中迫切需要抑制DTX的毒副作用,提高其治疗效果。
超声因其无辐射、无创和成本效益等非凡优势而首次在临床诊断中得到探索 [9, 10]。尽管具有上述独特的功能,但它在基于纳米结构材料的治疗前景中也获得了很多关注[11]。声动力疗法最初是通过超声触发的空化和“声孔效应”实现的。在这个过程中产生的 ROS 有效地诱导了对癌细胞的细胞毒性,导致肿瘤细胞的 DNA 快速损伤和凋亡 [9]。与光动力疗法 (PDT) 的表面应用不同,SDT 在通过低强度聚焦超声 (LIFU) 治疗深部肿瘤时获得了更令人愉悦的治疗效果,其优点是可以防止治疗过程中出现不希望的热损伤 [12, 13]。作为 SDT 的基本要素之一,声敏剂已在各种肿瘤治疗中进行了探索 [14]。氯 e6 (Ce6) 最初被用作一种常见的光敏剂,但其理想的治疗能力和对肿瘤组织的良好亲和力使其也能够用作声敏剂 [15]。作为氯家族的第二代,Ce6 因其理想的 ROS 生成而引起了肿瘤治疗的广泛关注 [16]。然而,Ce6 的单一应用不断引起不稳定以及不良的皮肤毒性,这都限制了 SDT 的广泛探索。
近年来,纳米技术的发展在生物传感、环境污染、污染物降解等诸多领域发挥了非常重要的作用[17,18,19,20,21,22,23]。基于纳米技术的纳米粒子具有许多优点,例如更小的直径、更大的外表面积和更低的内部扩散阻力[24]。此前,纳米粒子已经参与了各种材料的应用,如MOF[25]、二氧化钛[26]和石墨烯[21]。纳米技术与癌症治疗相结合的快速增长趋势也得到了广泛探索,为促进联合治疗策略铺平了可行的道路[27]。为了实现有效的肿瘤治疗应用,精心设计的纳米粒子主要通过增强渗透性和保留 (EPR) 效应来提高有毒化疗药物的运输效率,从而增加肿瘤部位的积累 [28, 29]。此外,还可以通过封装化学疗法来减少不需要的副作用。据报道,全氟戊烷(PFP)在辐照下具有出色的从液相转变为气相的能力,可作为一种新型的超声分子探针,特别是在超声成像和治疗中[30]。更重要的是,初始液相使 PFP 能够很容易地封装在各种材料中 [31]。此外,根据上述EPR效应,PFP的封装可以大大提高肿瘤部位的超声成像能力,避免较大微米级微泡引起的尺寸限制。除了肿瘤成像,多种治疗方法的结合在纳米系统导向的癌症治疗中具有巨大的价值。具体而言,包括声动力学疗法和化学疗法的整合在内的实践强调了对传统治疗效率的革命性改变。徐等人。 [32] 证明由于 SDT,化疗药物可以显示增强的治疗结果,从而导致线粒体靶向肿瘤细胞凋亡的激活。这种模式揭示了协同处理,在未来的利用中备受关注。
在此,受上述启发,我们打算利用多合一纳米粒子(CPDP NPs)建立一个诊断和治疗系统,该系统通过以 SDT 为导向的协同治疗结合化疗以及增强的超声成像。由于作为理想的纳米载体具有优异的安全性和理想的代谢稳定性,PLGA 使其有利于探索各种抗肿瘤能力 [33, 34]。因此,在该策略中,使用 PLGA 作为外层材料,PFP 可以通过其由 LIFU 触发的相移能力显着增强超声成像,而理想的第二代声敏剂 Ce6 也可以暴露于 LIFU 以诱导 ROS 生成。此外,伴随着DTX的释放,将实现化疗与SDT并存,最终实现协同治疗。重要的是,CPDP NPs 的核壳结构可以在不伤害正常组织或细胞的情况下稳定给药,并且还使纳米粒子具有相对较高的封装效率 [35]。此外,这种核壳结构中的内容物可以得到很好的保护 [36,37,38],尤其是 PFP,由于结构的存在,它可以有效地从液体转化为气体。使用这种核壳结构,可以以更稳定的方式同时展示协同策略。首先,协同策略有助于通过有效的包封显着降低DTX的副作用,这对于减轻治疗恶性肿瘤和侵袭性肿瘤的痛苦具有重要意义;其次,与单一化疗相比,SDT联合化疗已被证实是一种有前景的提高治疗效率的策略。第三,PFP实现的增强超声成像优化了诊断策略,也有助于验证抗肿瘤效果。最后但并非最不重要的是,整个系统安全稳定,具有出色的生物相容性。值得强调的是,在该策略中,肺转移和肿瘤生长在体外和体内均得到显着抑制。综上所述,该协同策略对乳腺恶性肿瘤及其远处转移具有良好的治疗效果,结合其超声成像能力,有望成为进一步临床应用的有前景的治疗策略。
材料和方法
材料
PLGA-COOH(Mw 12,000 Da)购自济南戴钢生物材料有限公司(中国济南)。全氟戊烷 (PFP) 和琼脂糖购自 Sigma-Aldrich Co., Ltd. (St. Louis, MO)。 Chlorin e6 (Ce6) 购自 Melone Pharmaceutical Co., Ltd.(中国大连)。 Cell Counting Kit-8 (CCK-8) 细胞毒性检测试剂盒购自 Dojindo Molecular Technologies(东京,日本)。 2',7'-二氯二氢荧光素二乙酸酯 (H2DCFDA) 购自 MedChemExpress Co., Ltd. (NJ, USA)。碘化丙啶 (PI) 购自 Solarbio Science and Technology Co. Ltd.(中国北京)。膜联蛋白 V-FITC/PI 从 BD Biosciences (USA) 获得。多西紫杉醇 (DTX) 购自 MedChemExpress Co., Ltd. (NJ, USA)。所有其他试剂均为分析纯产品,未经进一步纯化。 Roswell Park Memorial Institute 1640 培养基(DMEM)、胎牛血清和酪氨酸购自 Gibco(ThermoFisher Scientific,美国)和紫外分光光度计(UV-Vis,Hitachi,Japan)。
CPDP NPs 的合成
Ce6-PFP-DTX/PLGA 纳米粒子(CPDP NPs)是根据之前的报道[39, 40]通过 W/O/W 双乳液法制备的。简而言之,首先将 2 mg Ce6 溶解在 500 μL 甲醇中。接下来,将 50 毫克 PLGA-COOH 和多西他赛 (2 毫克) 溶解在 4 毫升二氯甲烷中,然后同时将之前的溶液加入其中。然后,将 200 μL PFP 添加到上述溶液中。因此,混合物由超声波探头(Sonics &Materials Inc.,美国)触发以获得第一个乳液(5 秒开和 5 秒关,3 分钟)。为了获得第二个乳液,将 8 mL 的聚(乙烯醇)(PVA)溶液(w/v =4%)加入到上述乳液中,使用相同的超声波探头持续 2 分钟。在最终乳液中加入 10 mL 2% 异丙醇后,将溶液在室温下机械混合至少 4 小时,使二氯甲烷完全挥发。最后,将 CPDP NP 离心 3 次(12,000 rpm,5 分钟),然后收集并储存在 4°C 以备进一步使用。除了Ce6之外,PDP NP以相同的方式制备。所有实验过程均在冰上进行,并严格在黑暗中进行。
CPDP NP 的特征
CPDP NPs 和PDP NPs 的粒径和zeta 电位由Malvern Zetasizer Nano 仪器(Malvern,UK)实现。通过透射电子显微镜(TEM)和光学显微镜表征形态。为了评估稳定性,将 CPDP NPs 溶解在磷酸盐缓冲溶液 (PBS) 中并分别测量 7 天的大小。每个样品一式三份测量。 CPDP NPs的包封率计算公式如下:
包封率(%) =(负载DTX或Ce6的重量/总DTX或Ce6的重量) × 100%。为了验证各种材料的封装,研究了各种样品的 UV-Vis 光谱(UH5300,Hitachi)。还通过TEM分析了有效封装。
CPDP NPs 的药物释放率
为了评估 Ce6 和 DTX 在 CPDP NPs 中的药物释放能力,使用具有不同 pH 值的两种溶液(磷酸盐缓冲溶液,PBS:7.4,乙酸盐缓冲溶液,ABS:5.6)来测试累积释放效率。简而言之,将混合物密封到透析袋中后,首先将 CPDP NPs 用 1 mL PBS 或 ABS 分散(Mw:10,000);然后将整个溶液转移到玻璃瓶中(总体积:150 毫升),其中加入 149 毫升 PBS 或 ABS 以保持溶液总体积为 150 毫升。然后将玻璃瓶放入 37°C 恒温摇床中,在不同时间(0.5、1、2、4、8、12、24、48、72 小时)收集溶液并立即补充介质的体积。每组重复3次。最后,分别在403和229 nm处通过Synergy Hybrid Multi-Mode Read(BioTek,美国)测量Ce6和DTX的浓度,并计算每个时间点的药物释放率。
体外超声成像
为了研究 CPDP NPs 的超声能力,乳液(1 mg/mL)首先由低强度聚焦超声(LIFU)换能器设备(中国重庆荣海超声医学工程研究中心)触发,导电模式为设置为 50% 占空比,不同强度下的 1 秒脉冲持续时间 (1–2 W/cm 2 ) 不同的持续时间。对于超声成像,使用飞利浦 EPIQ5 超声诊断仪(探头频率:12 MHz,MI:0.06)分别将辐照的 CPDP NP 添加到先前制备的琼脂糖模型中,以观察 CPDP NP 的 2D 和 CEUS 成像。同时应用ImageJ软件分析各组灰度值。
LIFU 辐照 CPDP NPs 的细胞摄取和体外 ROS 生成
鼠乳腺癌细胞系 4T1 获自中国科学院上海细胞库(中国上海),并在混合有 10% FBS 和 1% 链霉素/青霉素的 RPMI 1640 培养基中在 37°C 下在 5% CO2 中培养加湿培养箱。
4T1细胞首先以1 × 10 4 的密度按照先前的条件进行培养 每个培养皿中的细胞,以在不同时间间隔(1 小时、2 小时、4 小时、8 小时)使用 CLSM 测试细胞摄取。为了验证 ROS 的产生,将细胞分为以下 5 组:对照、CPDP NPs、LIFU、Ce6 + LIFU、CPDP NPs + LIFU。常规培养 24 小时后,分别用 CPDP NP(200 μL,0.8 mg/mL)或 Ce6 溶液(200 μL)代替培养基,并将细胞再共同孵育 3 小时。然后,LIFU 照射 (2 W/cm 2 , 120 s) 分别根据不同的组进行。共培养和LIFU处理后,加入100 μL稀释的DCFH-DA溶液,每组在之前的培养箱中培养15分钟。共聚焦激光扫描显微镜(CLSM)用于确认活性氧产生的结果,并通过ImageJ软件测量相应的荧光强度。
CPDP NPs 的体外细胞毒性和协同治疗能力
应用 CCK-8 测定来评估 CPDP NPs 的细胞毒性。简而言之,4T1鼠乳腺癌细胞在96孔板中培养,密度为1 × 10 4 每孔 24 小时。然后,CPDP NPs 用不同浓度的无血清 RPMI 1640 培养基稀释(0、0.2、0.4、0.6、0.8 mg/mL,n =3),有或没有 LIFU 照射 (2 W/cm 2 , 120 秒)。再经过24小时的共培养过程,进行4T1细胞的细胞活力。
为了评估协同处理的细胞凋亡效率,将 4T1 细胞与之前一样共培养 24 小时,然后分成以下五组:(1)对照(没有任何处理),(2)LIFU(仅在 2 W/cm 2 )、(3) CPDP NPs(仅使用 0.8 mg/mL 的 CPDP NPs 溶液)、(4) PDP NPs + LIFU 和 (5) CPDP NPs + LIFU。在各种纳米粒子共孵育 (200 μL) 和 LIFU 暴露后,每组用膜联蛋白 V (5 μL) 和碘化丙啶 (5 μL) 双染色 20 分钟,并通过流式细胞术方案进行分析。
细胞转移的体外抑制
为了研究对细胞转移能力的抑制,设计了伤口愈合试验和 transwell 试验。对于伤口愈合测定,4T1 细胞按常规在 6 孔板中培养。细胞生长至 80% 汇合后,使用移液器吸头 (10 μL) 沿着 6 孔板的中心进行人工划痕。然后,在上述相同的组中处理细胞。连续共孵育24 h后,用PBS洗涤细胞3次,光学显微镜(Olympus,Japan)下观察。
对于 transwell 测定,transwell 室(康宁,圣地亚哥,美国)的顶部隔室主要用于模拟体内的细胞外基质。 4T1细胞密度为1 × 10 5 将每孔细胞接种到无血清 RPMI 1640 培养基中的上室,而底部隔室填充有混合了 10% FBS 的完全培养基。然后,与上述组一样分离细胞并分别处理 24 小时。之后,底面的细胞用多聚甲醛固定并用结晶紫染色。结果用光学显微镜(Olympus,日本)观察。
体内超声成像
健康雌性 BALB/c 小鼠(4 周)和昆明小鼠(4 周)获自宁夏医科大学实验动物中心。所有动物实验均在宁夏医科大学动物福利伦理审查委员会批准的指导方针下进行。为建立小鼠荷瘤模型,BALB/c小鼠接种4T1乳腺癌细胞(1 × 10 7 /mL) 在右侧。肿瘤大小增长到 60-80 毫米后 3 , BALB/c 小鼠通过尾静脉静脉注射 CPDP NPs (200 μL, 1 mg/mL)。 24 h 后,用 LIFU (2 W/cm 2 , 120 s),然后通过前面提到的飞利浦 EPIQ5 超声诊断仪获取 2D 和 CEUS 成像。灰度分析采用ImageJ软件进行测量。
CPDP NPs 的体内协同治疗效率
4T1癌细胞接种后,每两天记录一次肿瘤的大小,按公式计算肿瘤体积:体积 =1/2 × 长度 × 宽度 2 .每 2 天记录一次肿瘤大小和小鼠体重,而每 3 天记录一次肿瘤生长的图片。当肿瘤体积达到60-80 mm 3 , 具有相似肿瘤大小的小鼠被随机分成相同的五组:对照、LIFU、CPDP NPs、PDP + LIFU 和 CPDP NPs + LIFU (n =3)。除对照组(用 200 μL PBS 代替)外,每组均通过尾静脉静脉注射各种 NPs(200 μL)。 24 小时后,用 LIFU 照射(2 W/cm 2 ) 120 秒。整个 SDT 给药每 3 天重复一次,持续 18 天。测量并计算小鼠的体重和肿瘤体积。治疗后处死小鼠,将肿瘤组织送至H&E、TUNEL和PCNA进行进一步的组织学分析。
CPDP NPs 体内生物安全
研究 CPDP 纳米粒子在体内的生物安全性,健康雌性昆明小鼠 (n =3) 分为以下 4 组:对照、5 mg/mL、10 mg/mL 和 20 mg/mL。 CPDP NPs (200 μL) 通过小鼠尾静脉注射;然后,小鼠可以自由进食和饮水,无需进一步给药。每 2 天测量一次小鼠的体重。 30 天后,处死小鼠并收集血样用于血细胞和生化分析。收集主要器官(心脏、肝脏、脾脏、肺和肾脏)并分别进行H&E染色研究。
肺转移的体内抑制
为了评价各组对肺转移的抑制作用,整个过程通过观察肺中转移结节的数量以及H&E染色组织学评估来进行。所有小鼠安乐死后,取出肺组织并固定;然后,拍摄癌结节照片,并用H&E染色进一步分析肺组织。
统计分析
测量数据均进行 3 次并表示为平均值 ± 标准偏差 (SD) 并通过单向方差分析或标准学生 t 进行分析 通过SPSS软件(版本:19.0)进行测试,而p 值 <0.05被认为具有统计学意义。
结果
CPDP NPs 的表征和药物释放效率
双乳液法被应用于制造CPDP NPs,它同时封装了相移材料PFP、声敏剂Ce6和化学药物DTX。当分散到 PBS 或去离子水中时,溶液呈现浅灰色外观。无论是通过光学显微镜还是透射电子显微镜观察,CPDP NPs 都表现出均匀的球形和清晰的核壳结构(图 1a、b)。 CPDP NPs 和 PDP NPs 的平均直径分别为 249.5 ± 77.46 nm 和 246.6 ± 81.01 nm,平均表面 zeta 电位分别为 -18.47 ± 0.55 mV 和 -3.67.46 mV 和 -3.68c。 CPDP NPs 的大小保证了它可以通过 EPR 效应被动地积累到肿瘤部位 [40]。 CPDP NPs 和PDP NPs 之间的不同电荷主要是由于带负电荷的Ce6 [41]。此外,CPDP NPs 的负 zeta 电位表明血浆蛋白吸附较低,验证了纳米颗粒的相对稳定性。粒度分布在 7 天内保持在 249.5 和 385.1 nm 之间(图 1e),证明了 CPDP NP 的相对稳定性。根据标准曲线,DTX和Ce6的包封率分别为83.84 ± 1.39%和60.54 ± 3.79%。
<图片>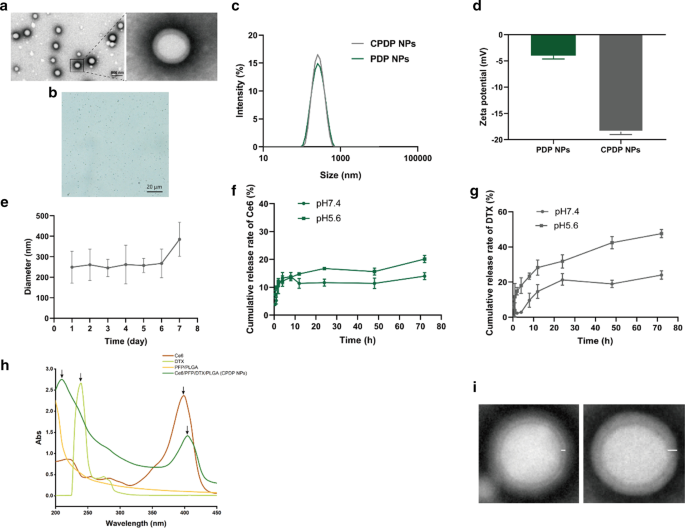
一 TEM(比例尺:500 nm)和 b CPDP NPs 的光学显微镜图像(比例尺:20 μm)。 c 尺寸分布和d PDP NPs 和 CPDP NPs 的 zeta 电位。 e 7 天内 CPDP NPs 的大小分布。 f Ce6和g的释放率 不同条件下 DTX 的释放率(pH 7.4 和 pH 5.6,n =3)。 h Ce6、DTX、PFP/PLGA 和 CPDP NPs 的紫外-可见光谱。 CPDP NPs 的箭头显示了 Ce6 和 DTX 的特征峰,表明两种材料的有效封装。 我 两种纳米粒子的 TEM 结果。 PFP/PLGA 纳米颗粒具有薄壳和圆形核(左)。包裹Ce6和DTX的CPDP纳米颗粒显示出更厚的外壳和椭圆形核(右)
图>如图 1f、g 所示,CPDP NP 中 Ce6 和 DTX 的药物释放效率与溶解在 pH 7.4 中的纳米颗粒相比,在 pH 5.5 下记录的 DTX 释放指数增加了近两倍,这表明药物释放是合理的在酸性肿瘤微环境中可以有效地实现 DTX 的速率。上述结果都表明,精心设计的CPDP NPs可以在酸性肿瘤环境中发挥稳定、及时的化疗药物释放作用,也是SDT制备的根本目标。
正如 UV-Vis 所显示的那样,DTX 和 Ce6 分别在 229 nm 和 403 nm 处显示出独特的吸收峰,相反,PFP/PLGA 没有显示出任何吸收峰。应该注意的是,CPDP NPs 的光谱在上述两个波长附近都表现出相似的峰值,而其余的则表现出 PFP/PLGA 光谱的相同趋势,表明各种材料的封装成功(图 1h)。为了进一步验证有效封装,图 1i 表明 PFP/PLGA 纳米颗粒具有更薄的壳和圆形核,而 CPDP 纳米颗粒由于 DTX 和 Ce6 的封装而显示出相对较厚的壳和椭圆形核。
体外超声成像
值得一提的是,PFP 具有出色的移相能力。液体到气体的转化不仅有助于纳米颗粒在肿瘤部位聚集,而且还使其能够提高超声成像的效率 [42]。为了证明这一点,LIFU 照射被用作引发 PFP 相变的触发器,即声学液滴汽化 (ADV) 效应 [43]。结果表明,在LIFU照射前灰度强度保持在较低水平,而在LIFU强度和照射时间增加后,2D和CEUS都显示出增强超声成像的趋势(图2a)。 ImageJ 的声学分析进一步证实了灰度值升高的结果(图 2b、c),这与成像结果一致。应该指出的是,当 LIFU 强度达到 2 W/cm 2 时,2D 和 CEUS 获得的结果最显着 并持续了 120 秒。以上结果表明,PFP成功地包裹在CPDP NPs中,并且在更高强度和更长的LIFU给药时间下超声成像能力得到显着提升。
<图片>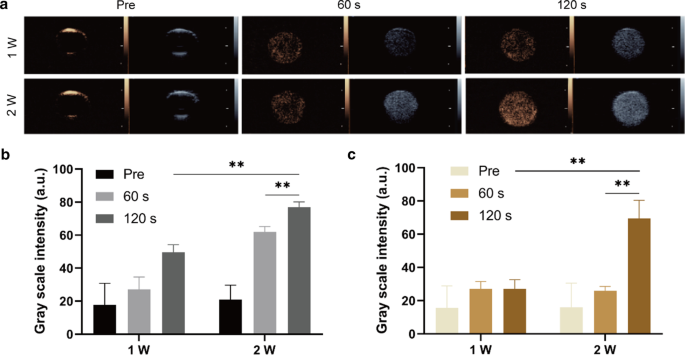
一 不同 LIFU 强度和持续时间下 2D 和 CEUS 的超声图像。 b 2D成像和c不同强度和时间对应的灰度强度 CEUS 成像 (**p <0.01, n =3)
图>LIFU 辐照 CPDP NPs 的细胞摄取和体外 ROS 生成
CLSM 结果如图 3 所示,CPDP NPs 的细胞摄取在不同的时间间隔内表现出增强的趋势,在 8 小时共孵育时达到最显着的聚集。
<图片>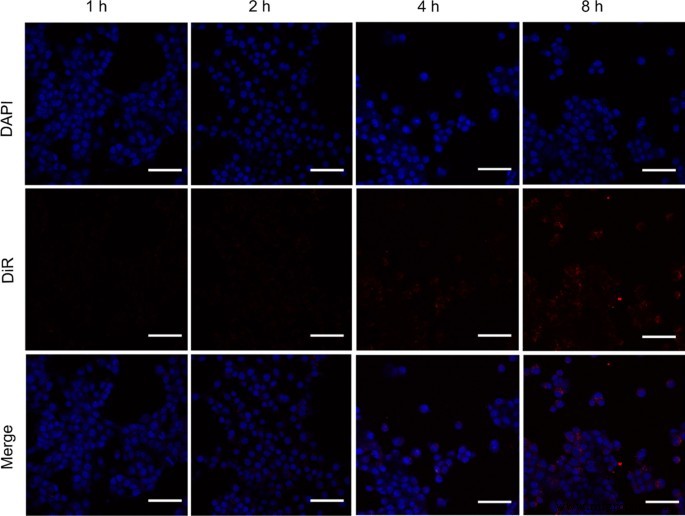
不同时间间隔内 CPDP NPs 的 4T1 细胞摄取(蓝色:DAPI 染色的 4T1 细胞。红色:DiR 标记的 CPDP NPs,比例尺为 50 μm)
图>声动力学疗法 (SDT) 的主要策略是产生 ROS——一系列单电子还原产物——以诱导癌细胞凋亡并抑制细胞增殖 [44]。需要强调的是,当暴露于超声波时,声敏剂很容易触发 ROS 的产生;同时,在整个过程中会释放大量的能量[45]。鉴于超声和声敏剂都是促进 SDT 的可有可无的元素,因此,设计和分析细胞内 ROS 的生成以研究不同组之间的差异。根据图 4a,游离 Ce6 加 LIFU 照射组产生的 ROS 量可以忽略不计,这可能是因为游离 Ce6 的快速代谢导致不令人满意的 ROS 产生。相反,CPDP NPs + LIFU组显示出最强的荧光强度。假设封装的 Ce6 受到良好保护,因此不会被代谢。因此,在 LIFU 刺激后,Ce6 被释放以产生丰富的 ROS。相比之下,在其他组中没有发现明显的荧光信号(图 4b)。
<图片>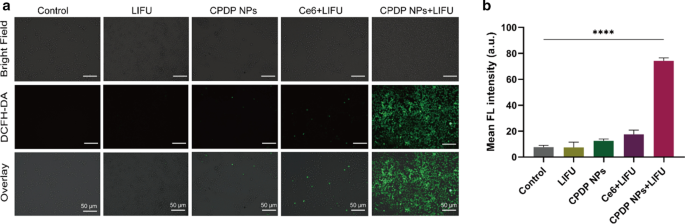
一 不同处理和 b ROS 生成的 CLSM 图像 相应的 FL 强度分析 (****p <0.0001, n =3)。比例尺为 50 μm
图>CPDP NPs 的体外细胞毒性和协同治疗能力
引入细胞计数试剂盒-8 (CCK-8) 分析来测试 CPDP NPs 的体外细胞毒性。在这方面,不同的组被设计为有或没有不同浓度的 LIFU 照射。结果表明,在没有 LIFU 暴露的情况下共孵育 24 小时后,即使在最高浓度(0.8 mg/mL)下,CPDP NPs 的存活率也没有明显影响,证明 CPDP NPs 具有理想的生物安全性(图 2)。 5a)。相比之下,LIFU照射后细胞活力显着降低,表明CPDP NPs和LIFU的结合显着引发了4T1细胞死亡,这与体外ROS的产生一致。
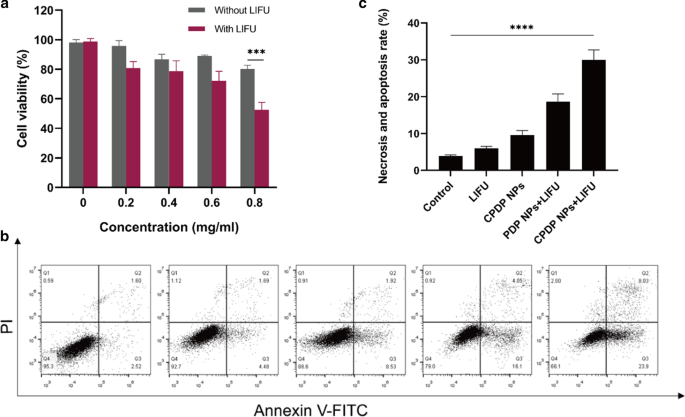
一 Relative cell viability with or without LIFU irradiation under different CPDP NPs concentrations. b 4T1 tumor cell apoptosis and necrosis by flow cytometry assay and c the data of corresponding necrosis and apoptosis rate analysis (****p < 0.0001, ***p <0.001, n =3)
图>To further evaluate SDT efficacy, a flow cytometry assay was introduced. As the results shown in Fig. 5b, c, the index of cell necrosis and apoptosis was highest observed in CPDP NPs + LIFU group, while other groups showed no obvious and necrosis and apoptosis. Notably, the necrosis and apoptosis rate of CPDP NPs + LIFU group was threefold higher than that of CPDP NPs only group, which ensured the significant tumor cell death efficiency of SDT from another respect. Intriguingly, compared with PDP NPs + LIFU group, cell necrosis and apoptosis rate in CPDP NPs + LIFU group was significantly increased, exhibiting the synergistic therapy efficiency of SDT and chemotherapy.
In Vitro Inhibition of Cell Metastasis
The invasive and migration capability of tumor cells are indispensable in tumor progression [46, 47]. As shown in Fig. 6a, the closure between the physical gap of CPDP NPs + LIFU group was significantly wider than other groups, indicating a relatively slower speed of migrating efficiency. According to the ImageJ software analysis (Fig. 6c), the migration rate of CPDP NPs + LIFU group was also remarkably reduced compared with other groups.
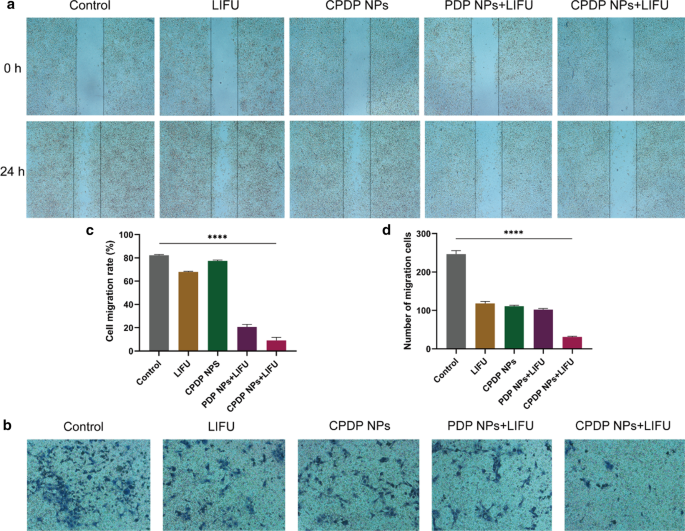
一 The wound healing and b The transwell assay after various treatments. c The corresponding migration rate of wound-healing assay. d The corresponding migration number of tranwell assay (****p < 0.0001, n =3)
图>Similarly in the transwell assay, compared with the swift migration speed of other groups, CPDP NPs + LIFU group revealed a significant reduction of cell number (Fig. 6b), which demonstrated an excellent anti-migration capability of the synergistic therapy. Specifically, with the absence of SDT (CPDP NPs only and LIFU only group), the number of tumor cells was mildly decreased (Fig. 6d). On the whole, due to the combination of SDT as well as chemotherapy, metastasis of 4T1 cells has been remarkably inhibited in vitro.
In Vivo Ultrasound Imaging
Since PFP was encapsulated in CPDP NPs, it is also necessary to evaluate the characteristic ultrasound imaging capability in vivo. After the injection via the tail vein of CPDP NPs, LIFU was then applied to the tumor site to acquire both 2D and CEUS imaging (Fig. 7a). The clear graphic difference between the two groups indicated that after LIFU irradiation, the corresponding intensity of CPDP NPs was elevated obviously compared with the pre-irradiation group. Further data of the average echo intensity also confirmed this result, which was also consistent with the in vitro imaging result previously (Fig. 7b, c).
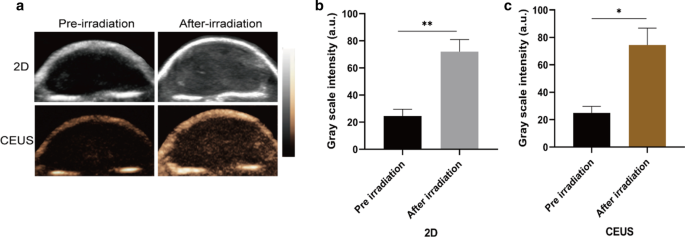
一 2D and CEUS images with and without LIFU irradiation. b , c The corresponding grayscale intensity analysis measured by ImageJ (**p < 0.01, *p <0.05, n =3)
图>In Vivo Synergistic Therapeutic Efficiency of CPDP NPs
Seeing from Fig. 8a, b, the tumor volume of CPDP NPs + LIFU group was significantly smaller after 18 days of treatment than that of other groups, which may attribute to the effectiveness of ROS originated from SDT treatment as well as chemotherapy to exert a valid synergistic therapy efficiency. Similarly, photographs of mice-bearing tumors (Fig. 8a) also showed the same trend, verifying the cooperative treating efficacy of CPDP NPs triggered by LIFU exposure. Furthermore, there was no obvious weight reduction of mice between different groups (Fig. 8c). The results above all indicated a much higher inhibition rate of CPDP NPs + LIFU group, revealing that the synergistic therapy could significantly prevent tumor growth.
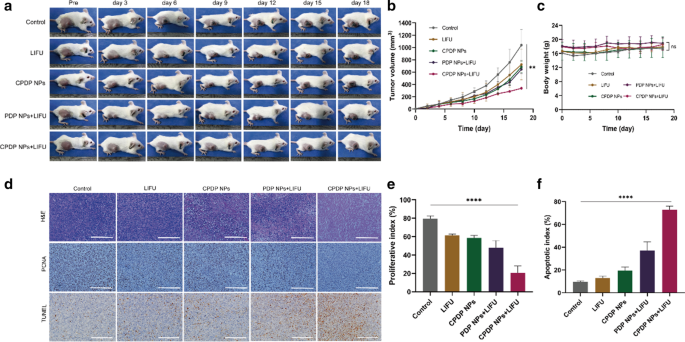
一 Images of tumor-bearing mice under various treatments within the certain 18 days (n =3)。 b Tumor volume analysis according to various treatments (**p <0.01)。 c Weights of tumor-bearing mice under various treatments (ns no significance, n =3)。 d H&E, PCNA and TUNEL results under various treatments (scale bar:200 μm). e Analysis of PCNA proliferation index of tumors under various treatments. f Analysis of TUNEL apoptotic index of tumors under various treatments (****p <0.0001, n =3)
图>To further testify the therapeutic results of all groups, both H&E, PCNA, and TUNEL staining were utilized (Fig. 8d). The proliferate rate of PCNA in CPDP NPs + LIFU group was only 20.50%, which was fourfold lower than control group, threefold lower than LIFU and CPDP NPs only group and twofold lower than PDP + LIFU group, respectively, demonstrating a significant anti-tumor proliferation rate (Fig. 8e). As it is shown in Fig. 8d, f, the TUNEL results indicated CPDP NPs + LIFU group exhibited an obvious apoptosis index of 72.86%, which was much higher than control (9.66%), LIFU (12.86%), CPDP NPs (19.59%), and PDP NPs + LIFU (37.06%) group. The results above all demonstrated the effectiveness of synergistic therapy exerted in vivo, which was also proved consistent with the previous in vitro results.
Biosafety of CPDP NPs In Vivo
Despite the effective therapeutic outcome, it is of great importance to explore the biosafety of the novel established nanoparticles as well. On behalf of the safe distribution of CPDP NPs in vivo, the metabolic safety was conducted. The results showed that instead of apparent body weight loss, the mice body weight elevated gradually in all the groups of mice (Fig. 9a), which indicated a negligible negative influence of CPDP NPs. In addition, as various organs and the blood samples exhibited in Fig. 9b, no significant changes were observed in blood cell, biochemistry analysis index, and H&E staining (Fig. 9c) among different treating groups, indicating the excellent biosafety of CPDP NPs in vivo.
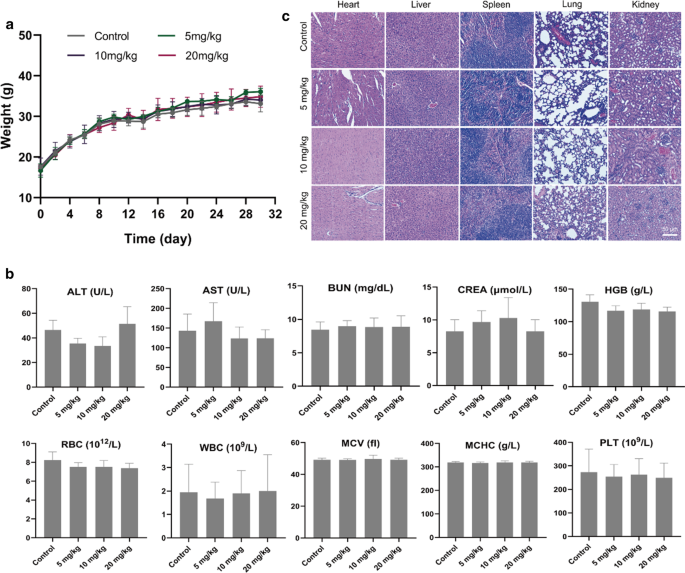
一 The weights of healthy Kunming mice under various concentrations of CPDP NPs (n =3)。 b The blood biochemistry and blood routine examination under various concentrations of CPDP NPs within a certain period of 30 days (n =3)。 c H&E results of different organs (heart, liver, spleen, lung, and kidney) of mice under the same treatment (scale bar:50 μm)
图>In vivo Inhibition of Lung Metastasis
It is well established that the lung is the main target organ for distant metastasis of breast cancer [48]. In order to evaluate the suppressing efficiency of metastasis, lung tissues of mice were utilized for anti-metastatic investigation. As seen from Fig. 10a, b, compared with control, LIFU, CPDP NPs, and PDP + LIFU group, the CPDP NPs + LIFU group exhibited the most remarkable decrease of lung nodules, which suggested its desirable lung metastatic inhibition efficiency. A similar trend of decreasing also further indicated by H&E staining (Fig. 10c), which in all demonstrated this synergistic therapy strategy could exert effective effort in eliminating lung metastasis in mice.
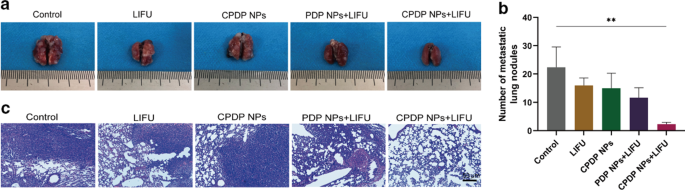
一 Images of the general appearances of lung tissues. b The analysis of the metastatic lung nodules between various treatments (**p <0.01, n =3)。 c Corresponding images of lung metastatic H&E staining results (scale bar:50 μm)
图>讨论
It has been greatly acknowledged that the metastasis of breast cancer will extensively influence its poor prognosis [3, 49]. As a desirable therapeutic approach, SDT may serve for its high efficiency and deep penetrating capability has been extensively convinced [11, 50]. Admittedly, since certain limitations exist in the single application of sonosensitizers, using SDT alone may still be less sufficient in further cancer exploration. The development of nanotechnology combined with clinical medicine has been promoted significantly in recent years, owning to the inspiring merits such as negligible toxicity, none invasiveness, and excellent biocompatibility. Using this novel approach, researchers have made many efforts in exploring multifunctional therapeutic strategies to enhance antitumor efficiency.
The biosafety is the priority of nano-agents. As a widely accepted material approved by the Food and Drug Administration (FDA) certification, it is highlighted that PLGA could be performed as a desirable carrier in application [51, 52]. Based on its advantages, we established a nano-system to realize multifunctional therapy efficiency, exploiting PLGA as the outer structure to encapsulate sonosensitizer Ce6, phase-shift material PFP and chemotherapeutic agent DTX. The CPDP nanoparticles (CPDP NPs) were primarily observed by the core–shell structure and appropriate size so that a desirable aggregation through the EPR effect could be achieved. The cell viability of CPDP NPs has been proved to be above 80% after 24-h coincubation, indicating the well safety of this nanoplatform. Besides, the phase transformation of encapsulated PFP has also guaranteed CPDP NPs as a contrast-enhanced agent when activated by LIFU. The enhanced ultrasound imaging capability will not only ensure the ideal therapeutic window but also promote the promising future for CPDP NPs to realize an integration of accurate diagnosis and precise treatment.
The key strategy in SDT is the generation of ROS. Compared with the single employment of Ce6, the encapsulated Ce6 in PLGA exerted a desirable protection, which could be verified by the ROS result. In our study, there was only a negligible amount of ROS generated by single use of Ce6, while nanoparticle-encapsulated Ce6 produced a considerable amount of ROS, which was further proved by SDT efficiency. The result indicated that ROS generation was preserved by the encapsulated Ce6 in CPDP NPs, which could lay a firm foundation for later tumor inhibition. Besides, the drug-releasing rate showed even at pH 5.5, little Ce6 was released from nanoparticles, demonstrating a well protection of sonosensitizer by PLGA, which was further proved by the ROS production and SDT outcome with high efficiency. Interestingly, the drug-releasing efficiency of DTX and Ce6 at pH 5.5 was quite different according to the results (more than 40% and around 20%, respectively), which may possibly cause by the diverse encapsulation efficiency of the two drugs. The minor releasing rate of Ce6 demonstrated that it could be effectively protected in PLGA so that substantial ROS generation through LIFU stimulation could be reached to get a more convinced SDT efficiency. Another reason for this difference may be due to the different solvents of the two drugs during the preparation process. Specifically, DTX and PLGA were directly dissolved in dichloromethane, while Ce6 needed to be first dissolved in methanol and then added dropwise to dichloromethane, due to its poor solubility in the latter [53]. Since drug release in nanoparticles is directly related to the effectiveness of subsequent combination therapy, the assessment of drug-releasing rate as well as ROS generation result has mutually proved this point. Current researches have suggested that using chemotherapy alone may not significantly reverse tumor progression [41]. In this study, the nanoplatform we designed demonstrated strong evidence that compared with the single employment of chemotherapy, the synergistic treatment has remarkably elevated therapeutic efficiency both in vitro and in vivo. Since LIFU stimulation optimized the therapeutic strategy, an increased cell apoptosis rate was remarkably elevated. It is worth noting that due to this synergistic strategy, lung metastasis could be significantly inhibited both at tumor cell level and inoculated mice model, which is consistent with previous reports [16, 52].
Conclusion
In conclusion, the safe and stable CPDP NPs we designed and prepared plus LIFU irradiation could remarkably eliminate breast tumor progression and its lung metastasis. With an enhanced imaging capability, this nanoplatform was also considered to be a promising contrast-enhanced agent in clinical. Hence, the novel synergistic strategy combined with LIFU might be considered as an effective treating application to reverse the poor outcome of metastatic breast cancer.
缩写
- LIFU:
-
低强度聚焦超声
- SDT:
-
Sonodynamic therapy
- PFP:
-
Perfluoropentane
- EPR:
-
Enhanced permeability and retention effect
- DCFH-DA:
-
Dichlorodihydrofluorescein diacetate
- PI:
-
Propidium iodide
- TEM:
-
透射电子显微镜
- ROS:
-
活性氧
- Ce6:
-
Chlorin e6
- DTX:
-
Docetaxel
- FBS:
-
胎牛血清
- CCK-8:
-
Cell Counting Kit-8
- CPDP NPs:
-
Ce6/PFP/DTX/PLGA nanoparticles
- PDP NPs:
-
PFP/DTX/PLGA nanoparticles
纳米材料
- 用于改进诊断和治疗应用的多功能金纳米粒子:综述
- 用于癌症治疗的纳米粒子:当前的进展和挑战
- 加载白藜芦醇的白蛋白纳米颗粒具有延长血液循环和改善生物相容性的高效靶向胰腺肿瘤治疗
- 用于体内 CT 成像和肾脏清除特性的新型生物相容性 Au Nanostars@PEG 纳米颗粒
- 用于光热疗法和光声成像的聚吡咯涂层铁铂纳米粒子的合成和体外性能
- 纳米粒子和超声波控制的水过冷
- 肿瘤靶向和生物相容性 MoSe2 纳米点@白蛋白纳米球作为协同光热放射治疗的双模态治疗剂
- 用于生物医学、药物输送和成像应用的磁性功能化纳米粒子
- 具有氧化还原不稳定聚合物壳的聚多巴胺复合纳米颗粒,用于控制药物释放和增强化学光热疗法
- 环境兼容的生物共轭金纳米粒子作为炎症诱导的癌症成像的有效造影剂
- Her2 功能化金纳米壳磁性杂化纳米粒子:一种用于乳腺癌双模态成像和光热治疗的治疗诊断剂
- 负载原卟啉 IX 的海带多糖纳米颗粒用于抗癌治疗、它们的细胞行为、ROS 检测和动物研究