富勒烯锚定还原氧化石墨烯杂化物的制备及其对环氧树脂阻燃性的协同增强
摘要
通过在 PEI 改性的还原氧化石墨烯 (rGO) 表面加入富勒烯 (C60) 制备 C60-PEI-rGO 杂化物,然后用于改性环氧树脂 (EP) 树脂。随后,GO 和 C60-PEI-rGO 杂化物的结构得到了很好的表征,表明 C60 均匀地固定在 PEI-rGO 的表面上。系统地研究了所制备的 C60-PEI-rGO/EP 纳米复合材料的阻燃性、机械性能和热稳定性。结果表明,C60-PEI-rGO 杂化物对 EP 具有高阻燃效率。具体而言,随着添加 1.0 wt% C60-PEI-rGO,环氧树脂的点燃时间从 68 秒增加到 89 秒,这在聚合物纳米复合材料中是不常见的。同时,改性环氧树脂的放热率峰值和总放热率峰值分别降低了40.0%和15.6%。 C60-PEI-rGO 与 EP 的协同阻燃机制归因于其独特的结构,结合了 C60 的高效捕获自由基、层状 rGO 的阻隔作用和环氧树脂交联密度的增加。结果表明,添加 C60-PEI-rGO 可同时提高环氧树脂的热稳定性和机械性能。该工作可能为制备同时具有其他改进性能的阻燃热固性树脂开辟一条新的、有效的方法。
背景
聚合物材料由于其重量轻、加工能力强和多功能等特点,已广泛应用于建筑、电气和电子、涂料等许多领域[1,2,3]。然而,大多数聚合物都是易燃的,这往往会引起安全问题[4]。因此,迫切需要提高聚合物材料的阻燃性。
与传统的阻燃剂相比,纳米填料不仅对高分子材料表现出更高的阻燃效率,而且还保留了其他原有的性能,甚至在低添加量的情况下赋予高分子材料一些特殊的功能,备受关注[5, 6] .其中,纳米填料的阻燃效果主要体现在峰值放热率(PHRR)、总放热量(THR)、总发烟量(TSR)等典型参数显着降低,或极限氧指数(意向书)。
然而,发现纳米填料对热塑性塑料和热固性树脂的阻燃效率是不同的。对于热塑性树脂,纳米填料可以显着提高阻燃性。例如,吉尔曼等人。通过熔融共混将表面活性剂改性蒙脱石掺入聚丙烯接枝马来酸酐 (PPgMA) 中,所得 PPgMA-MMT (4 wt%) 纳米复合材料的 PHRR 和 THR 分别比纯 PPgMA 低 75% 和 49%,仅在添加时4 wt%,表明阻燃性显着提高 [7]。此外,在聚甲基丙烯酸甲酯 (PMMA)/碳纳米管和聚丙烯 (PP)/石墨烯纳米复合材料中也观察到了类似的显着改进 [8,9,10,11]。这些纳米填料还被用于改性热固性树脂以提高其阻燃性[12, 13]。
然而,注意到这些纳米填料在热固性树脂中的阻燃效率不如它们在热塑性树脂中的显着。在我们之前的工作中,氧化石墨烯 (GO) 被掺入环氧树脂 (EP) 中,与 EP [14] 相比,在 1 wt% GO 含量下,所得纳米复合材料的 PHRR 仅降低了 16%。郭等人。在石墨烯/EP 中显示出类似的现象,与 EP 相比,石墨烯含量为 1 wt% 时,环氧树脂的 PHRR 降低了 23%。这种现象背后的原因可以通过纳米填料对交联结构的影响以及纳米填料对聚合物燃烧的作用来解释。一方面,由于热固性树脂具有较高的交联密度,纳米填料的加入难以显着改变交联密度,这对提高阻燃性起决定性作用[15, 16]。另一方面,纳米填料对聚合物阻燃的作用机制较为单一,主要依赖于其阻隔作用[17, 18],进而难以发挥高改性效率。显然,与这些在热塑性塑料领域取得的引人注目的进展相比,纳米填料在热固性树脂中的改性效率需要进一步提高。许多努力致力于用其他阻燃剂改性纳米填料 [12, 19]。例如,胡和他的同事用八氨基苯基多面体低聚倍半硅氧烷 (OapPOSS) 改性石墨烯,获得 OapPOSS-rGO,它对环氧树脂表现出显着的阻燃效果 [20]。然而,一些重要的指标如点火时间(tign)和放热率达到峰值的时间鲜有报道,其协同机制有待进一步研究。
最近,富勒烯 (C60) 已被掺入聚合物中以提高聚合物的阻燃性,因为它对自由基具有高反应性,自由基可以作为自由基捕获剂来延迟聚合物的热氧化降解 [21,22,23, 24]。然而,C60纳米粒子由于其比表面积大、范德华力强,容易在聚合物中团聚,导致阻燃效率低。由于化学成分相同,C60与其他碳纳米填料具有协同作用,它不仅提高了纳米填料的分散性,而且结合了C60的自由基吸收和其他碳纳米填料的阻燃作用如石墨烯的阻隔作用,进一步提高了 C60 的阻燃效率 [25,26,27]。与碳纳米管等一维碳纳米材料相比,石墨烯由于其层状纳米结构显示出更高的阻隔效率,并提供了更活跃的平台以与 C60 协同[28]。因此,如果 C60 和石墨烯的这些阻燃机制可以协同应用于聚合物,那将是有趣的。方和他的同事将GO和C60结合获得纳米杂化物,大大提高了HDPE的阻燃性和热稳定性[29]。然而,迄今为止,所有这些与C60相关的纳米材料都被掺入到热塑性树脂中,而没有研究人员研究它们在热固性树脂中的阻燃效果和机理。
在此,我们通过三步反应设计了一种由石墨烯和富勒烯(C60)组成的石墨烯相关杂化物(C60-PEI-rGO),并掺入环氧树脂中。 C60-PEI-rGO松散的层状和富含氨基的结构不仅可以实现石墨烯和C60在环氧树脂(EP)中的理想分散,充分发挥C60的自由基吸收、石墨烯的阻隔作用,并增加交联密度所得纳米复合材料的性能,但也可以改善所得纳米复合材料的其他典型性能。相信这项工作可能开创一种新的、有效的方法来制备同时具有其他改进性能的阻燃热固性树脂。
方法
材料
石墨(3000 目)由阿拉丁工业有限公司(中国)提供。硫酸 (H2SO4, 98%)、硝酸钠 (NaNO3)、高锰酸钾 (KMnO4)、过氧化氢 (H2O2, 30% aq.)、乙醇、二甲亚砜 (DMSO)、甲苯和丙酮是分析级的商业产品无需进一步纯化即可使用。蒸馏水是我们实验室生产的。 C60(纯度> 99%)购自河南濮阳有限公司。支化聚乙胺(PEI,50% aq.)购自Sigma-Adrich,Mn为70,000。双酚 A 的二缩水甘油醚 (DGEBA) 购自上海树脂厂有限公司(中国)。固化剂二乙基甲苯二胺(DETDA)购自中国崇顺化工有限公司。
C60-PEI-rGO 的制备
氧化石墨 (GO) 是使用改进的 Hummer 方法从石墨粉末制备的,如附加文件 1 [30、31] 中所示。 PEI 改性的还原氧化石墨烯 (PEI-rGO) 是通过 PEI 和氧化石墨烯之间的反应制备的,如附加文件 1 所示。之后,PEI-rGO(150 毫克)通过超声波分散在 DMSO(300 毫升)中30分钟。将 PEI-rGO/DMSO 溶液和 300 毫克 C60 添加到 DMSO-甲苯(350 毫升,4:3,v /v ) 超声波溶液;然后,在室温下超声处理 30 分钟后,将所得混合物在 90°C 下搅拌 24 小时。最后,将产物依次用甲苯和乙醇洗涤至少 3 次,然后在 60°C 下真空干燥 12 小时,命名为 C60-PEI-rGO。 C60-PEI-rGO的制备过程如Scheme 1所示。
<图片>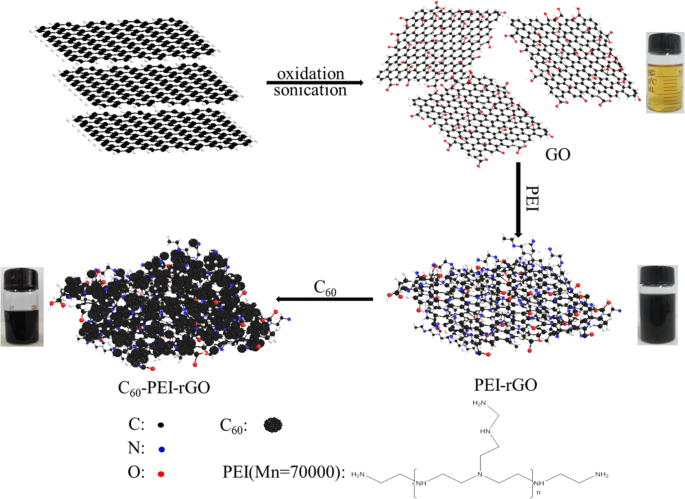
C60-PEI-rGO制备示意图
图>EP 树脂和纳米复合材料的制备
将适量的 DGEBA 和 DETDA 以 1:0.234 的重量比在 100°C 下在剧烈搅拌下混合 15 分钟,得到浅黄色液体,即 EP 预聚物。然后,将混合物在真空烘箱中在 110°C 下彻底脱气 30 分钟,然后将其倒入预热 (100°C) 的“U”型模具中。随后,将模具放入烘箱中,分别按照 120°C/1h + 180°C/2.5h 和 190°C/2h 的方案进行固化和后固化;所得树脂是固化的EP树脂。将适量的 PEI-rGO、C60 和 C60-PEI-rGO 分别加入 EP 预聚物和乙醇的混合物中,超声处理 30 分钟,形成黑色悬浮液,然后将混合物在 60 ℃下脱气除去多余的溶剂。 °C 在真空烘箱中。之后,分别通过 120°C/1h + 180°C/2.5h 和 190°C/2h 的程序将混合物浇铸到模具中进行固化和后固化。最后,将所得纳米复合材料脱模并编码为 PEI-rGO1.0/EP、C601.0/EP C60-PEI-rGOn /EP,分别为 1.0 和 n 表示所得纳米复合材料中所用纳米材料的重量百分比 (n =0.4, 0.6, 0.8, 和 1.0).
仪器及实验方法
通过原子力显微镜 (AFM) (Veeco Instruments, Nanoscope Multimode IIIa, USA)、透射电子显微镜 (TEM) (JEOL JEM-2010, Japan)、电子显微镜(SEM)(HITACHI,SU8010/EDX,日本),傅立叶变换红外(FTIR)光谱仪(AVATAR360N,美国)。纳米填料的热重分析 (TGA) 在 TA Instruments STA449C(美国)上在 25 至 800°C 范围内在氮气气氛下以 10°C/min 的加热速率进行,环氧树脂及其复合材料在在空气气氛下以 10、20、30 和 40°C/min 的加热速率在 25 到 800°C 的范围内升温。动态力学分析 (DMA) 使用来自 TA Instruments (USA) 的 TA DMA Q800 装置在 25 至 250°C 下进行,加热速率为 3°C/min,频率为 1Hz。拉伸性能根据 ASTM D638 以 5 毫米/分钟的恒定速度使用 1 kN 的称重传感器进行。根据 ASTM D2863/77,在 Stanton Redcraft 火焰计(中国)上测量 LOI 值。根据 ISO 5660,在 FTT 设备(英国)中使用锥形量热计表征树脂的可燃性,入射通量为 35 kW/m 2 使用锥形加热器。
结果与讨论
GO、PEI-GO 和 C60-PEI-rGO 的特征
一般来说,GO在常用的有机溶剂中很难很好地分散[32]。然而,在 PEI-rGO 和 C60-PEI-rGO 的制备中,值得注意的是所制备的 PEI-rGO 和 C60-PEI-rGO 可以很容易地分散在乙醇中并形成稳定的胶体悬浮液,这可归因于PEI 与乙醇的相容性高。该结果为石墨烯基纳米复合材料制备中GO的剥离和分散提供了有利条件。此外,水中的黄色GO颜色转变为黑色的PEI-rGO和乙醇中的C60-PEI-rGO,表明GO发生了还原反应。
图 1 显示了 GO、PEI-rGO、C60-PEI-rGO 和 C60 的 FTIR 光谱。与 PEI 复合后,3431 cm −1 处的氢键峰强度 由于 PEI 分子对 GO 的部分还原,以及 1719 cm -1 处的特征带,显着降低 完全消失,同时在 1385 (O–H) 和 1058 (C–O) cm -1 处的两个峰明显减弱 . 1623 cm −1 处的强波段 在 GO 中与未氧化石墨畴的骨架振动有关,它被 1640 cm -1 处的强带取代 这与酰胺键的形成有关 [33, 34]。此外,在 1463 cm −1 处的新波段 由于 PEI 到 GO 的覆盖,(C-N 伸缩振动)出现在 PEI-GO 中。对于 C60-PEI-rGO,C60 在 1426、1180、574 和 525 cm -1 处的四个特征吸收峰 以及 2973 cm −1 处的新峰 (C60-H) 反映了 C60 和 PEI-rGO 之间的反应,因为未反应的 C60 通过用甲苯洗涤混合体数次被完全去除,直到洗涤液的颜色在超声处理下从茄子色变为透明 [26]。
<图片>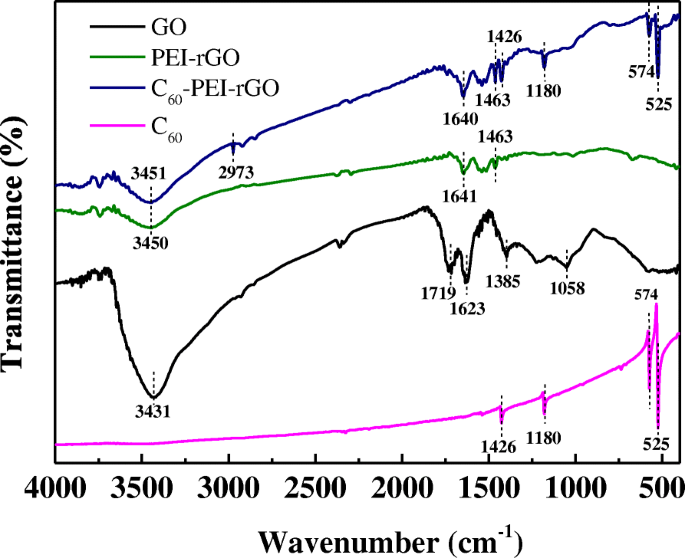
GO、PEI-rGO、C60-PEI-rGO和C60的FTIR光谱
图>图 2 显示了 GO、PEI-rGO 和 C60-PEI-rGO 的 XPS 光谱。可以看出,286.7 和 532.6 eV 处的两个尖峰分别对应于 GO 及其杂化物中的 C 和 O 元素。对应于 N1 的 399.7 eV (PEI-rGO) 和 400.1 eV (C60-PEI-rGO) 处的新尖峰表明功能化后形成酰胺键。 GO 及其杂化物的粗略化学成分也显示在图 2a 中。对于 PEI-rGO,GO 中 O 的含量从 28.72 at.% 减少到 14.41 at.%,这归因于 PEI 的引入。在 C60-PEI-rGO 的情况下,O 和 N 的含量分别下降到 7.74 原子%和 5.71 原子%,而 C 的含量达到 86.55 原子%,这是 C60 被引入 PEI 的明显证据-rGO。可以粗略计算出C60在C60-PEI-rGO中的重量比约为。 45.4 wt% 根据“混合规则”(at.% 在计算中已更改为 wt%)。在 PEI-rGO(图 2b)和 C60-PEI-rGO 图 2c)的 N1s 光谱中,PEI-rGO 的 N1s 光谱拟合到约 3 个峰。 399.1 eV(占 41.4%)、400 eV(占 35%)和 400.7 eV(占 23.6%),分别归属于伯胺、仲胺和叔胺。对于 C60-PEI-rGO,叔胺和仲胺中的 N 含量分别增加至 26.6% 和 43.8%,而伯胺中的 N 含量显着降低至 29.6%。基于仲胺中N含量的增加和伯胺中N含量的降低,表明C60主要与伯胺反应生成仲胺,少量C60与仲胺反应生成叔胺,这可归因于C60的空间效应和胺的化学活性。
<图片>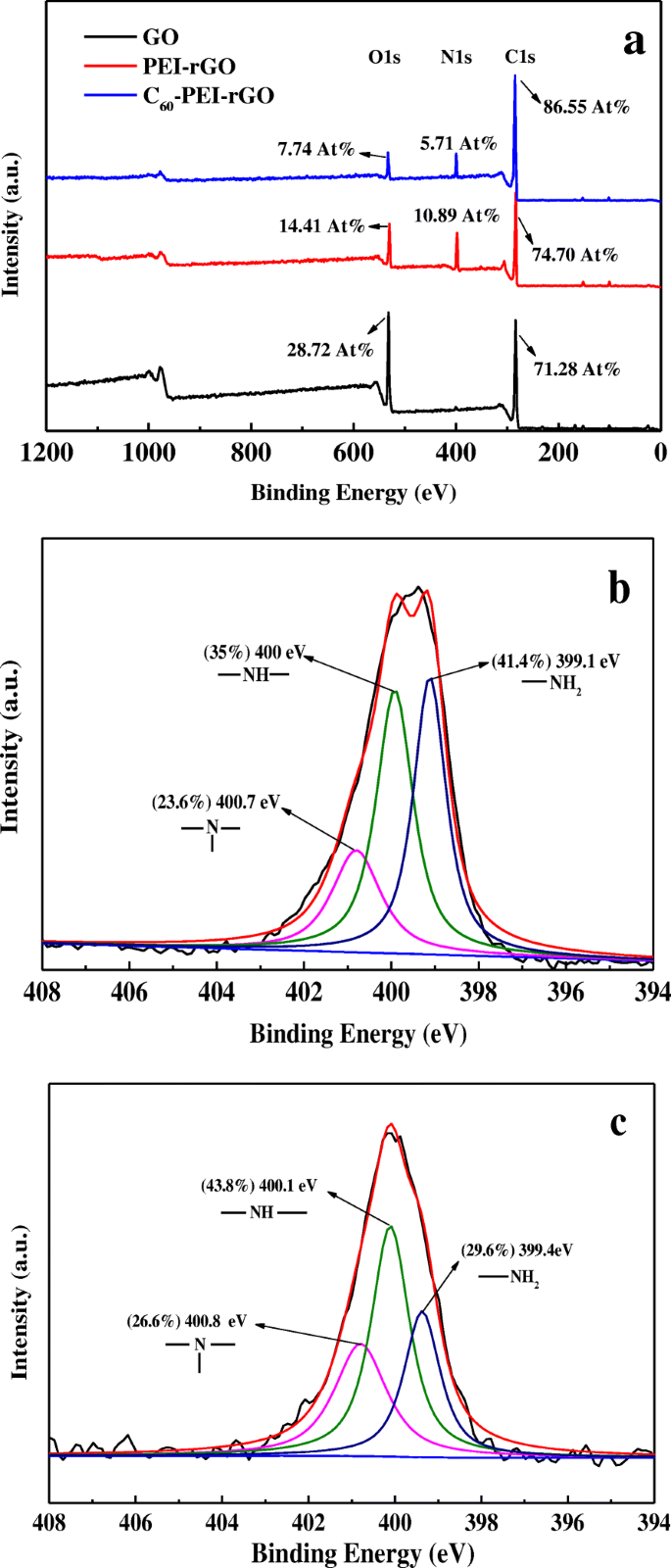
GO、PEI-rGO 和 C60-PEI-rGO (a ),以及 PEI-rGO 的 N1s XPS 光谱 (b ) 和 C60-PEI-rGO (c )
图>通过 AFM 和 TEM 研究了 GO、PEI-rGO 和 C60-PEI-rGO 的形态。如图 3 所示,GO 的尺寸覆盖 0.2-1 μm,厚度约为。 0.9 nm,这表明 GO 已经剥离并且可能是单层纳米片的形式。在 PEI 覆盖后,PEI-rGO 纳米片的厚度增加到大约1.5 纳米,表面高度分布均匀。 PEI 分子被吸附在 GO 片的两侧,即平均厚度约为0.3 纳米。如图 4 所示,PEI-rGO 纳米片表现出典型的光滑层状结构,而有趣的是发现 C60-PEI-rGO 具有松散的层状结构和约。 20 nm C60 聚集体均匀分布在 PEI-rGO 表面。可以预期,这种松散的层状结构不仅可以防止干燥过程中杂化纳米片的重新堆积,导致杂化纳米片在聚合物中的均匀分散,而且还改善了 C60-PEI-rGO 与 EP 基质之间的物理相互作用.
<图片>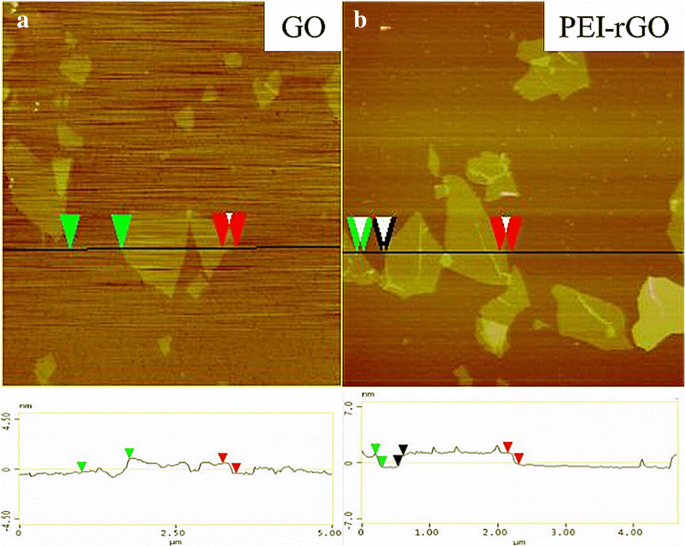
GO (a ) 和 PEI-rGO (b )
图> <图片>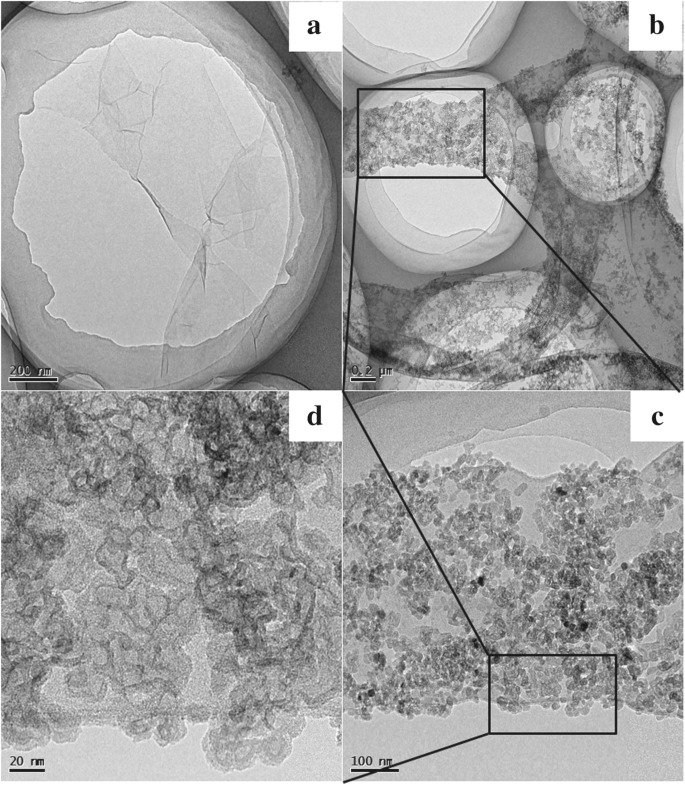
PEI-rGO (a ) 和 C60-PEI-rGO (b , c , d )
图>GO 及其杂交体的 TGA 曲线如图 5 所示。GO 的曲线显示约。由于吸收的水的解吸,在 150°C 以下重量损失 4%,大约从 200 到 600°C,42.9 wt% 的重量损失可归因于含氧基团的分解。在 PEI-rGO 的情况下,可以看出,重量损失约。 15.6 重量%发生在 100 至 190°C,这是由于吸收的水分解,以及 270 至 470°C 的失重阶段(约 26.7 重量%),主要源于 PEI 的分解和更稳定的氧-包含组。对于 C60,它在氮气中表现出很高的热稳定性,在 600°C 下的质量残留率为 99.3%。对于 C60-PEI-rGO,降解率明显降低,600°C 时的质量残留增加到 79.4%,显示出在 GO 和杂化物中最高的热稳定性。通过比较 PEI-rGO、C60 和 C60-PEI-rGO 的质量残留物,可以计算 C60 在 C60-PEI-rGO 中的重量比,大约为55.2 重量%;该结果与 XPS 结果 (45.4 wt%) 有 10 wt% 的差异,但仍然可以考虑 C60 在 C60-PEI-rGO 中的重量比约为。 50 重量%。
<图片>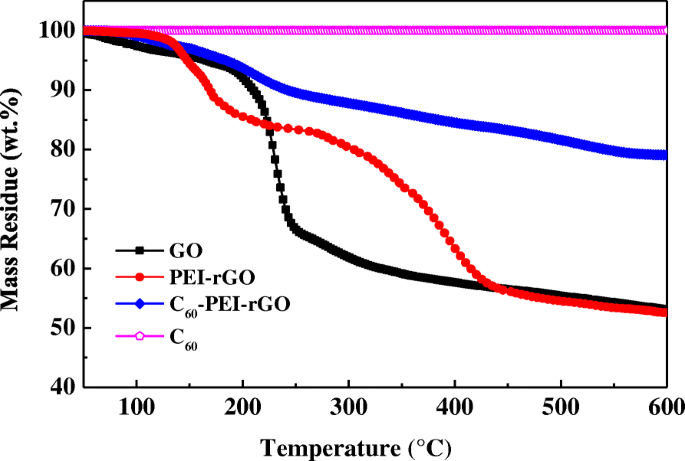
GO、PEI-rGO、EP、C60-PEI-rGO和C60在氮气氛下的TG曲线
图>基于上述结果,C60-PEI-rGO与乙醇表现出高相容性,导致在EP基质中的分散性优于C60,或者通过PEI和C60的化学键合成功制备了EP中单独的GO,最终会影响其性能得到的纳米复合材料。
C60-PEI-rGO/EP 纳米复合材料的结构
一般来说,结构决定了材料的性能。因此,有必要阐明纳米复合材料的结构,包括纳米填料的分散等宏观结构和基体的界面相互作用和交联密度等微观结构。
用该基团将 GO 功能化是一种广泛使用的方法,用于增加 GO 或石墨烯与聚合物之间的界面相互作用 [35]。在此,PEI分子中的胺基被设计为引入到GO表面,为优异的阻燃性和综合性能提供了保证。 Neat EP、PEI-rGO1.0/EP 和 C60-PEI-rGO0.6/EP 断面的 SEM 图像可以观察到,如图 6 所示。可以看出 PEI-rGO 和 C60 -PEI-rGO在EP基质中分散良好,无明显聚集,并表现出较高的粗糙度,表明PEI-rGO和C60-PEI-rGO分散良好,与EP的界面相互作用强。
<图片>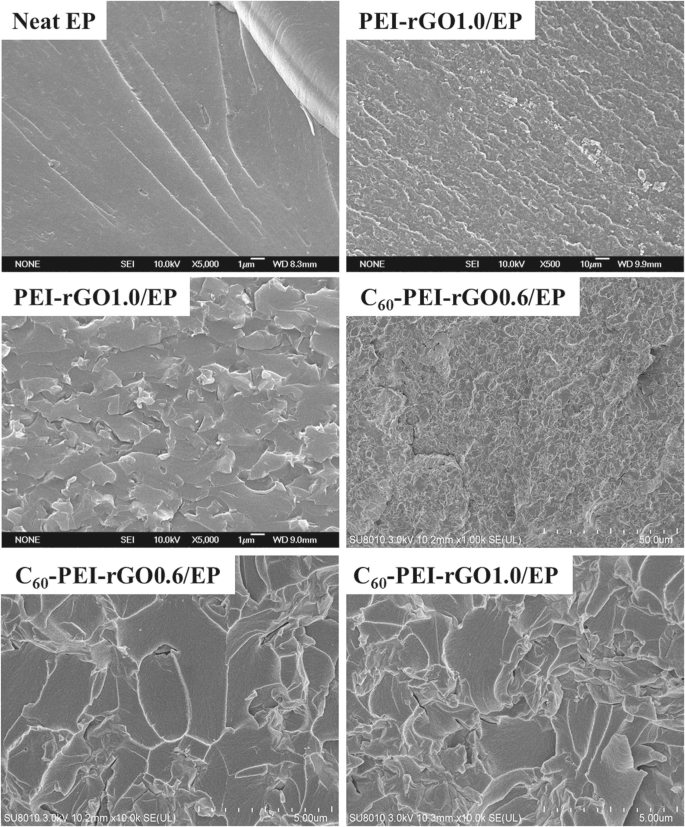
固化EP树脂及其纳米复合材料的SEM图像
图>橡胶平台的模量是聚合物网络的一个参数,是聚合物体系和填料之间相互作用程度的一般指标[36]。如图 7 所示,与纯 EP 相比,C60-PEI-rGO/EP 和 PEI-rGO1.0/EP 纳米复合材料都表现出更高的橡胶平台模量,表明 EP 和杂化物之间的界面相互作用很强。注意到C60-PEI-rGO1.0/EP纳米复合材料的橡胶平台模量高于PEI-rGO1.0/EP。可以解释,PEI 的胺基在固化过程中与 EP 键合并作为偶联点,增加了纳米复合材料的交联密度,此外,对于 C60-PEI-rGO,它不仅具有胺GO 表面上的基团,但也有粗糙的表面,与上面讨论的 EP 有更强的物理相互作用。通常,填料的加入通常会导致聚合物链的松散堆积,最终导致填料与聚合物之间的界面相互作用较弱 [37]。然而,在这项工作中,PEI-rGO和C60-PEI-rGO中的胺基倾向于缩短交联点之间的距离,导致PEI-rGO1.0/EP和C60-的交联密度增加。 PEI-rGO/EP,此外,C60-PEI-rGO的粗糙表面可以通过物理相互作用增强C60-PEI-rGO与EP之间的界面相互作用。
<图片>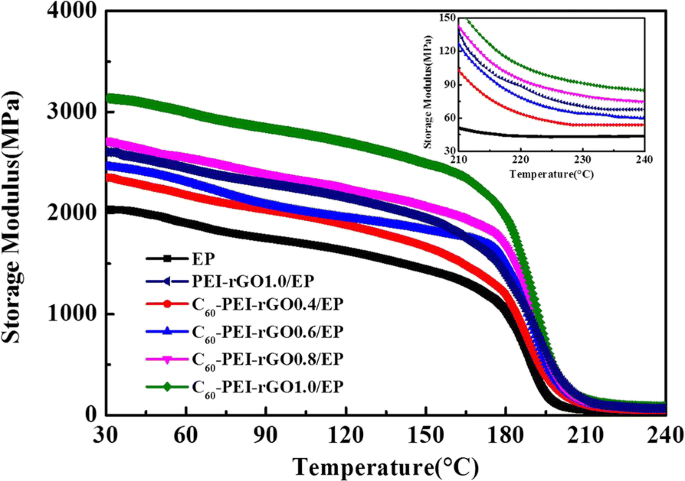
固化EP树脂及其纳米复合材料的储能模量(E')DMA曲线
图>固化 C60-PEI-rGO/EP 纳米复合材料的阻燃性及机理
锥形量热法和 LOI 是评估聚合物材料阻燃性的两种有效方法。图 8 显示了固化 EP 及其纳米复合材料的锥形量热仪曲线,典型参数和 LOI 值汇总在表 1 中。可以看出,将 C60-PEI-rGO 掺入到 EP 树脂中可以显着减缓燃烧过程。具体而言,C60-PEI-rGO/EP 的 PHRR 时间和时间显着增加。特别是,与纯 EP 相比,C60-PEI-rGO1.0/EP 纳米复合材料的 tign 增加了 21 秒,PHRR 的时间增加了 28 秒。同时,与纯 EP 相比,C60-PEI-rGO/EP 纳米复合材料表现出显着较低的 PHRR 和略低的 THR。其中,与纯 EP 相比,C60-PEI-rGO1.0/EP 的 PHRR 和 THR 分别降低了 40.0% 和 15.6%。此外,环氧树脂的 LOI 值随着 C60-PEI-rGO 的加入而增加,具体而言,固化后的 C60-PEI-rGO0.8/EP 纳米复合材料的 LOI 值最大,为 30.1%,约为 1.18 倍。纯EP树脂。此外,PEI-rGO 1.0/EP和C601.0/EP的LOI值分别为27.5和26.3,低于C60-PEI-rGO1.0/EP。显然,C60-PEI-rGO 对 EP 表现出很高的阻燃效率。此外,值得注意的是,C60-PEI-rGO1.0/EP 比 PEI-rGO1.0/EP 和 C601.0/EP 具有更好的阻燃性,进一步证明了功能化的 GO 和C60 对增强阻燃性的作用可以通过 PEI 对 GO 表面的 C60 进行共价官能化来发挥。如上所述,将 C60-PEI-rGO 掺入 EP 树脂中会增加交联密度,这是导致 C60-PEI-rGO/EP 纳米复合材料阻燃性提高的重要因素。样品的 UL-94 垂直燃烧结果在附加文件 1:表 S1 中给出。随着 C60-PEI-rGO 的加入,火焰传播速度略有降低。然而,没有任何样本可以达到 V-1 或 V-0。
<图片>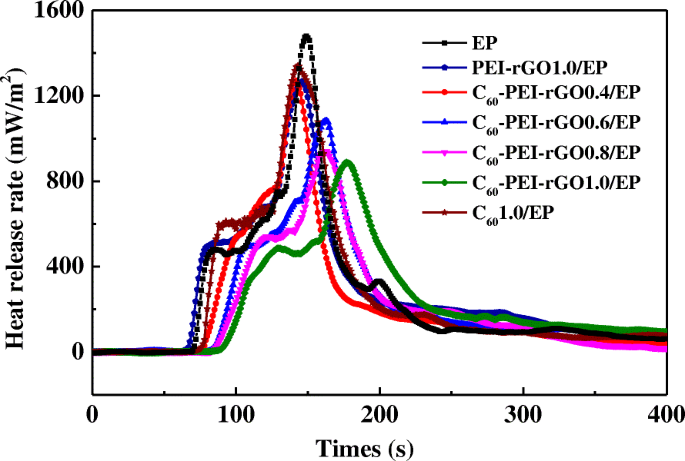
固化EP树脂及其纳米复合材料的放热速率与时间的关系
图> 图>为了进一步证实 C60-PEI-rGO 对阻燃性的影响,对固化的 C60-PEI-rGO/EP 和 EP 树脂的热氧化稳定性进行了评估,因为聚合物的阻燃性直接关系到热氧化降解步骤是否容易进行。具体而言,原始和改性 EP 树脂的热降解动力学通过 Kissinger 方法进行计算和比较 [38]。材料的热重动力学可以通过方程计算。 1:
$$ \ln \left(\beta /{T}^2\right)=\left(-{E}_{\mathrm{a}}/\mathrm{R}\right)\left(1/\mathrm {T}\right)-\ln \left[ ARn\left(1-\alpha \right)n-1/{E}_{\mathrm{a}}\right] $$ (1)其中 β 是最大降解速率下的加热速率 (K /min), T 是最大降解率 (K ), E a 是活化能(J/mol),R 是摩尔气体常数 (=8.314 J/mol K),A 是指前因子 (1/s),n 是分解顺序,α 是分解的分数。
引入四种加热速率(10、20、30 和 40 K/min)来研究热降解动力学。图 9 显示了固化的 EP 及其纳米复合材料的 TG 和 DTG 曲线。这里,第 1 阶段和第 2 阶段分别与大分子链的分解和焦残留物的氧化有关。表 2 显示了固化 EP 和固化 EP 纳米复合材料在空气气氛中不同加热速率下的 TG 分析的相关数据。活化能 (Ea) 可以从 ln(β /T 2 ) 与 1/T 图(图10),计算数据汇总在表3中。C60-PEI-rGO加入EP树脂后,第一降解阶段的Ea值有不同程度的变化,继续增加时增量增加C60-PEI-rGO 的加载。但是,E 的值 a是第二阶段略有变化。
<图片>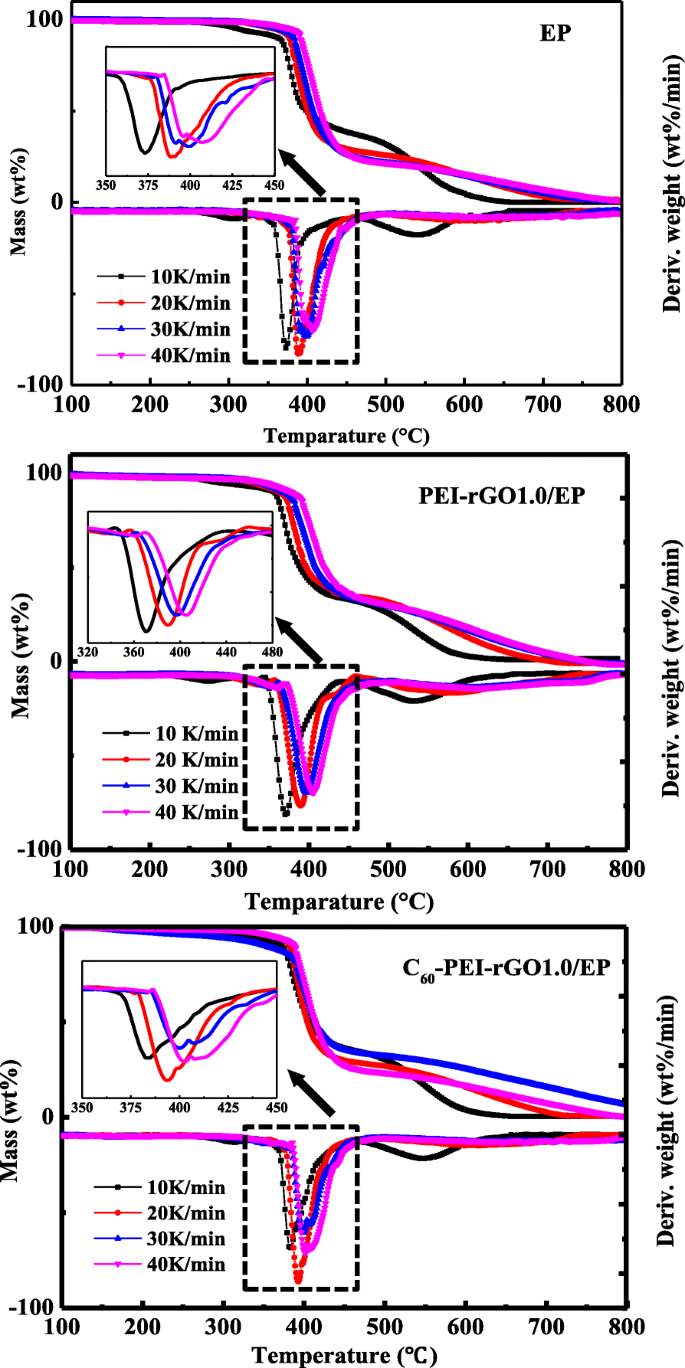
不同升温速率下固化EP树脂、PEI-rGO1.0/EP纳米复合材料和C60-PEI-rGO1.0/EP纳米复合材料的TG和DTG曲线
图> 图>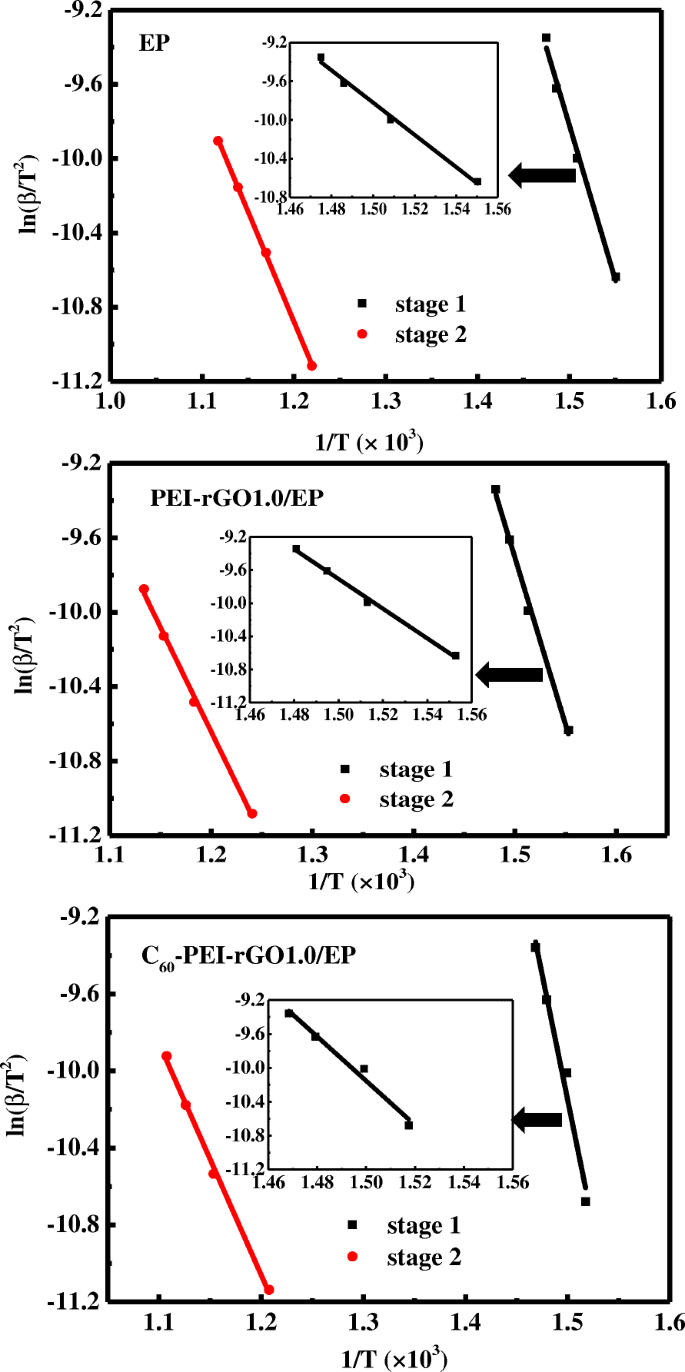
ln(β /T 2 ) 对 1/T 固化EP树脂、PEI-rGO1.0/EP纳米复合材料和C60-PEI-rGO1.0/EP纳米复合材料的不同分解阶段
图> 图>结果表明,随着 C60-PEI-rGO 的加入,与大分子链分解相关的初始热降解变得困难。可以解释为,C60对大分子链分解产生的自由基具有较高的捕获效率,需要较高的能量来保持分解,导致分解延迟。同时,未观察到单独添加 C60 对第一降解阶段 Ea 值的显着改善,这是由于分散性差导致比界面面积低。显然,增加的活化能表明环氧树脂的燃烧随着 C60-PEI-rGO 的加入而延迟和抑制。然而,如附加文件 1:图 S2 和 S3 中分别显示的炭的数字图像和 SEM 图像,加入 C60-PEI-rGO 后炭的重量和微观结构没有明显变化,这与结果一致锥形量热法,表明加入C60-PEI-rGO不影响炭的形成。
基于上述讨论,提出了如图 11 所示的阻燃机制。一方面,正如对 EP 及其纳米复合材料结构的讨论,C60-PEI-rGO 中的胺基倾向于缩短它们之间的距离。交联点并增加所得纳米复合材料的交联密度,这对提高EP的阻燃性起着积极的作用。另一方面,C60与石墨烯的协同作用对提高EP的阻燃性也起到了积极的作用。 Firstly, C60 aggregations with the size of 20 nm anchored evenly on the surface of PEI-rGO and the resultant large specific surface area can take them full use on trapping radicals and increases the activation energy of thermo-oxidative decomposition of EP chains. This effect delays the thermo-oxidative decomposition of the resultant nanocomposites, which reflects in some key index such as the time to ignition. Secondly, C60-PEI-rGO which possesses a layered structure acts as a physical barrier that hinders the transfer of volatile gases and heat. Finally, the combustion of nanocomposite is eased up and then the flame retardancy of C60-PEI-rGO/EP nanocomposites can be significantly improved.
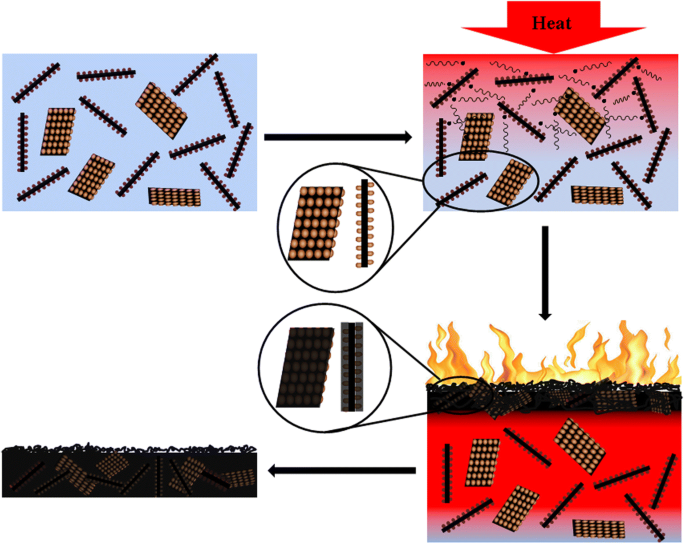
Schematic combustion processes of C60-PEI-rGO/EP nanocomposites
图>The Other Typical Properties of C60-PEI-rGO/EP Nanocomposites
Nowadays, there is a trend towards developing novel flame retardancy materials with simultaneously improved comprehensive properties rather than only attractive flame retardancy [4]. Therefore, it is necessary to evaluate other typical properties of C60-PEI-rGO/EP nanocomposites.
Figure 12 shows the tensile strength (Ts), and Young’s modulus (Yc) of cured EP resin and resultant nanocomposites. It is attractive to find out that all C60-PEI-rGO/EP nanocomposites have higher values of tensile strength, and Young’s modulus than those of EP resin, demonstrating that C60-PEI-rGO/EP nanocomposites have the significantly improved tensile properties. Young’s modulus of C60-PEI-rGO1.0/EP reaches 2810 MPa, which is 1.35 times of EP (2081 MPa). Generally, the rough fractured surface is considered as a reflection on strong interfacial interaction between the polymer and graphene, which will lead to a high Young’s modulus [37, 39]. As shown in Fig. 6, it can be seen that PEI-rGO1.0/EP, C60-PEI-rGO0.6/EP and C60-PEI-rGO0.8/EP nanocomposites exhibit rougher fractured surfaces comparing with the neat EP. These results indicate that the stress can be effectively transferred between graphene layers and EP matrix through the interface phase, and the graphene layers fully exert their high stiff in nature and divert the course of crack propagation when exerting the load on the nanocomposites. The stronger force is needed to offset the absorbed energy by graphene layers and thus leads to the higher Young’s modulus of nanocomposites. However, the biggest incremental improvement of tensile strength occurs in C60-PEI-rGO0.8/EP, and the value up to 77.4 MPa, which is 1.22 times of cured EP. This can be explained that the high viscosity of C60-PEI-rGO1.0/EP leads to the difficulty on completely eliminating the organic solution which plays a negative role on the tensile strength of the resultant nanocomposite.
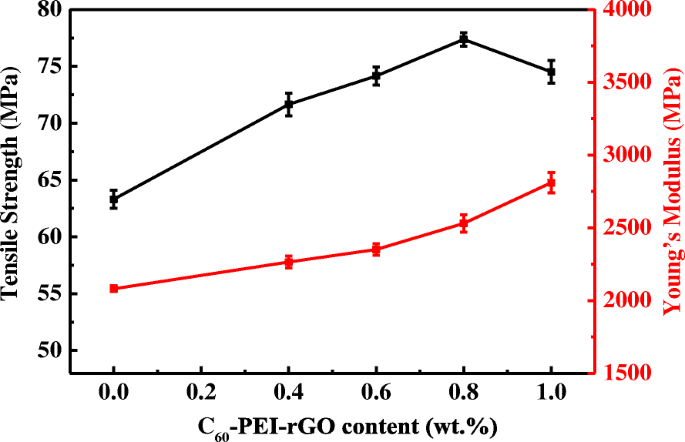
Tensile strength and Young’s modulus of EP resin and its nanocoposites
图>Figures 7 and 13 show DMA curves of cured EP and its nanocomposites, the storage modulus (E s) of cured EP significantly increase with the loading of C60-PEI-rGO, especially at lower temperature. The highest value of E s (3125 MPa) occurs in C60-PEI-rGO1.0/EP, which is increased by 53.7% compared to that of neat EP (2039 MPa) at 30 °C. As the same trend as the E s, the T g value of the C60-PEI-rGO/EP nanocomposite shifts towards higher temperature and the T g value of C60-PEI-rGO1.0/EP is up to 191.7 °C which is an increment of 11.3 °C compared to that of neat EP. Meanwhile, PEI-rGO1.0/EP has slightly increased T g and significantly increased E s compared to neat EP, which accords with the results in other reports about functional graphene polymeric nanocomposites [39]. These result indicate that the functionalization of GO exhibits a positive effect on the properties of resultant nanocomposites. It is noted that the improvement of C60-PEI-rGO1.0/EP is more effective than that of PEI-rGO1.0/EP, which is attributed to physical interaction between C60 aggregations and EP matrix.
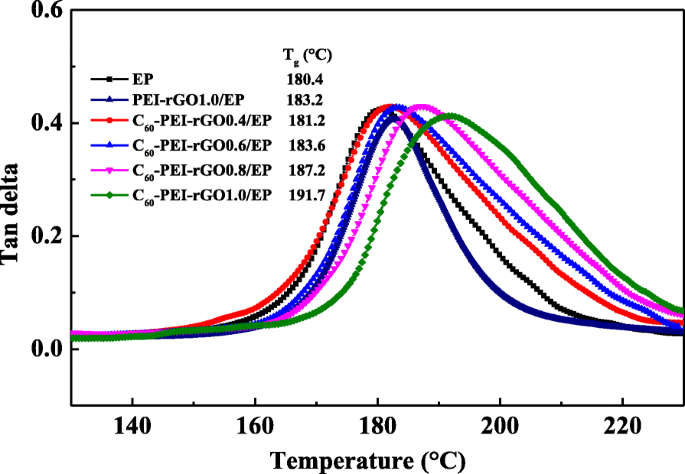
DMA curves of loss factor (tan delta) of cured EP resin and its nanocomposite
图>TG/DTG profiles for cured EP and its nanocomposites are shown in Fig. 14. The temperature (T onset) at 5 wt% of the weight loss of the sample and the temperature (T max) at maximum weight loss rate of samples are given. It can be seen that the thermal degradation process of neat EP has three stages, which mainly correspond to the vaporization of small molecules, the decomposition of the macromolecular chains, and the oxidation of char residue, respectively [20]. In case of PEI-rGO1.0/EP, the T onset (287 °C) is lower than that of neat EP (299 °C), while T m is not significantly changed, which could be due to the thermally unstable of PEI-rGO. For C601.0/EP and C60-PEI-rGO/EP nanocomposites, T onset and T max are shifting to high temperature. Specially, C60-PEI-rGO1.0/EP exhibits the best thermal stability, the 28 °C increment of T onset and 16 °C increment of T max compared to that neat EP are observed. While for C601.0/EP, the T onset increases by 16 °C and has no significant change on T m, which could be due to the highly effective free radical-trapping effect of C60. However, the T onset and T m of C601.0/EP are lower than those of C60-PEI-rGO/EP at equal content of nanofillers, which shows that C60-PEI-rGO is more effective than C60 or PEI-rGO alone in enhancing the thermal oxidation stability of EP. As described above, on the one hand, the layered structure of modified GO nanosheet increases the crosslinking densities of the resultant nanocomposites. Besides that, it creates a “Tortuous path” to form a gas barrier in degradation and provides a platform on which C60 could anchor evenly by chemical bond; the distribution of C60 in EP has improved. On the other hand, C60 acts as a radical trapping reagent during the process of degradation that delays the thermo-oxidative degradation of EP.
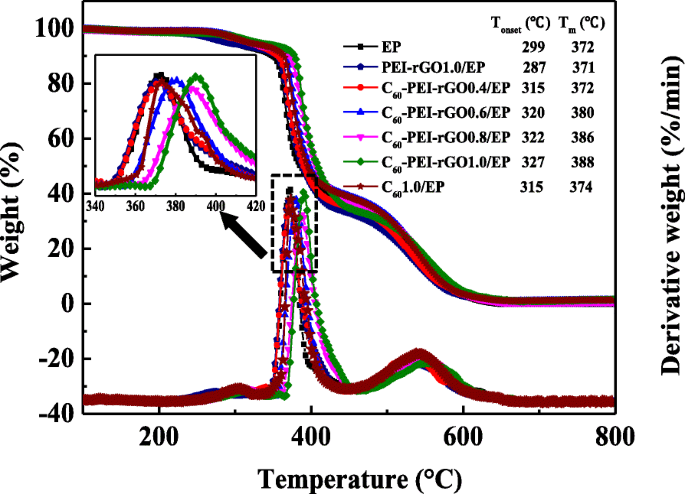
TG and DTG curves of cured EP resin and its nanocomposites in an air atmosphere
图>Layered nano-materials, such as graphene, clay, and layered double hydroxides, have been considered as potential multi-functional flame retardants. Comparing these nanomaterials, (i) the C60-PEI-rGO developed herein exhibits highly modified efficiency on flame retardancy of EP by combining multi-effects such as increase of crosslinking density, barrier effect of layered structure, and radical absorption of C60, and (ii) it endows modified resin with outstanding thermal stability and mechanical properties. Therefore, this work provides a new template to fabricate high flame retardant thermosetting resin with improved comprehensive properties.
结论
C60 was chemically anchored on the surface of PEI modified GO, and the resultant hybrid (C60-PEI-rGO) was successfully prepared. C60 aggregations with the size of ca. 20 nm are uniformly distributed on the surface of PEI-rGO, and C60-PEI-rGO exhibits a loose lamellar and amino-rich structure. The C60-PEI-rGO shows high flame retarding efficiency for EP. Specially, C60-PEI-rGO1.0/EP shows 40.0 and 15.6% reduction in the PHRR and THR compare to neat EP, respectively. More importantly, t ign and times to PHRR of C60-PEI-rGO1.0/EP nanocomposite procrastinate for 21 s and 28 s compare to that of neat EP, respectively. This C60-PEI-rGO hybrid increases the crosslinking densities of the resultant nanocomposites and acts as a physical barrier that hinder the transfer of volatile gases and heat due to the layered structure; meanwhile, C60 aggregations are uniformly dispersed in EP resin by anchoring on the surface of PEI-rGO, acting as a radical trapping reagent which delays the thermo-oxidative degradation of the resultant nanocomposites. Moreover, it is noted that the C60-PEI-rGO not only is a high effective flame retardant but also is a potential nanofiller for fabricating high-performance thermosetting resins.
缩写
- 原子力显微镜:
-
原子力显微镜
- C60 :
-
Fullerene
- DETDA:
-
Diethyltoluenediamine
- DGEBA:
-
Diglycidyl ether of bisphenol A
- DMA:
-
Dynamic mechanical analysis
- DMSO:
-
二甲亚砜
- EP:
-
Epoxy
- FTIR:
-
Fourier transform infrared spectrometer
- 开始:
-
氧化石墨烯
- LOI:
-
Limiting oxygen index
- PEI:
-
Branched polyethlyamine
- PHRR:
-
Peak heat release rate
- rGO:
-
还原氧化石墨烯
- SEM:
-
扫描电子显微镜
- TEM:
-
透射电子显微镜
- TGA:
-
热重分析
- THR:
-
Total heat release
- TSR:
-
Total smoke release
纳米材料
- 环氧树脂的历史
- 木工和环氧树脂
- 用环氧树脂项目打动亲朋好友
- 钛酸盐纳米管装饰氧化石墨烯纳米复合材料:制备、阻燃和光降解
- 石墨烯和氧化石墨烯的体外和体内生物安全和抗菌能力
- 氧化石墨烯杂交 nHAC/PLGA 支架促进 MC3T3-E1 细胞的增殖
- 石墨烯/WO3 和石墨烯/CeO x 结构作为超级电容器应用电极的评估
- 通过多元醇介导工艺制备和表征 ZnO 纳米夹
- 纳米级 CL-20/氧化石墨烯的一步球磨制备显着降低粒径和灵敏度
- 金属和金属氧化物纳米粒子的绿色合成及其对单细胞藻类莱茵衣藻的影响
- 氧化锌纳米粒子的特性及其对微生物的活性
- 用于高性能超级电容器的阴离子表面活性剂/离子液体插层还原氧化石墨烯