通过金属氧化物纳米粒子调整细胞形态力学扰动
摘要
如今在商业产品中越来越多地使用纳米颗粒 (NPs) 与对其潜在危害的全面了解不符。需要更多的体外研究来解决 NPs 的理化特性如何引导它们在细胞内的吞噬以及它们的细胞内运输、命运和毒性。这些纳米生物相互作用尚未得到广泛解决,尤其是从机械角度来看。细胞力学是细胞健康的关键指标,因为它通过细胞骨架重排调节细胞迁移、组织完整性和分化等过程。在这里,我们在体外研究了 Caco-2 和 A549 细胞系的弹性扰动,根据 SiO2NPS 和 TiO2NPS 诱导的杨氏模量修饰。与 SiO2NPs 相比,TiO2NPs 对细胞弹性的影响更大,因为它们在肌动蛋白网络中诱导了显着的形态学和形态学变化。 TiO2NPS 增加了 Caco-2 细胞的弹性,而在 A549 细胞上观察到了相反的效果。这些结果表明,细胞弹性的改变与 NPs 毒性之间存在相关性,而这又取决于 NPs 的理化性质和所测试的特定细胞。
背景
工程纳米粒子 (ENP) 在商业产品中的大量使用正在提高人们对其对人类和环境的潜在毒性的认识 [1]。迄今为止,已经进行了许多体外和体内研究,目的是阐明毒性的分子机制 [2, 3]。然而,由于缺乏标准化的操作程序,了解纳米颗粒 (NPs) 与生物体之间的相互作用相当困难,这导致当前可用的文献数据存在争议 [4, 5]。已确定 NP 的不利影响严格取决于它们的理化特性和所测试的特定细胞或生物体 [6]。因此,NP 的表征是获得可靠数据的基础 [7]。金属氧化物纳米粒子在商业产品中广泛使用 [8]。其中,无定形 SiO2NPs 和结晶 TiO2NPs 在广泛的工业领域中用作药物和化妆品的添加剂,以及保健品、打印机墨粉、油漆、食品包装和食品添加剂 [9, 10]。因此,这些 NP 很可能可以通过不同的途径(摄入、吸入和皮肤渗透)进入生物体 [11]。例子包括但不限于基于 TiO2NPs(商业标签中标记为 E171)和 SiO2NPs(商业标签中为 E551、E554、E556)的食品,它们已经有了巨大的增长 [12,13,14]。目前对 SiO2NPs 和 TiO2NPs 的研究表明它们积极干扰关键的细胞机制。例如,它们已被证明可刺激细胞因子释放(从而促进炎症)[15,16,17] 来破坏肠道微绒毛 [18, 19],诱导 ROS 产生 [20],抑制 ATP 合成 [21],并诱导遗传毒性 [22,23,24,25,26]。然而,很少有研究探讨这些 NP 是否与细胞力学相互作用 [27],这是一个需要进一步研究的主题。细胞粘附和细胞骨架重排对于维持细胞稳态确实至关重要 [28]。细胞骨架结构的任何变化都会扰乱细胞力学并影响细胞弹性和迁移动力学 [29]。在这项研究中,我们仔细评估了 20 nm SiO2NPs 和 TiO2NPs 对 Caco-2 和 A549 细胞的生物力学影响,Caco-2 和 A549 细胞是类似于暴露于 NPs 的组织的最佳模型。我们初步探索了它们的进入机制,并评估了细胞活力、膜损伤和 ROS 的产生以及超氧化物歧化酶 (SOD) 和丙二醛 (MDA) 的激活。然后,我们专注于通过原子力显微镜 (AFM) 表征 NPs 孵育后细胞弹性(杨氏模量)的变化。我们的结果表明,正如杨氏模量的变化所证实的那样,NPs 可能会诱导皮质肌动蛋白的显着重组。特别是,已观察到 SiO2NPs 对 TiO2NPs 慢性毒性的主要生物相容性。我们将细胞毒性研究与生物力学表征相结合的方法代表了一种新的潜在方法,可用于标准化 NP 毒性评估方案。
方法
非晶SiO2NPs的合成
按照 Malvindi 等人的方法,在室温下通过混合水、有机溶剂(环己烷,J.T. Baker)、表面活性剂(Triton X-100,Sigma-Aldrich)制备三元 W/O 微乳液。 [25]。简而言之,将 880 μL Triton X-100、3.75 mL 环己烷、170 mL 水和 50 μL TEOS(98%,Sigma-Aldrich)混合并搅拌 30 分钟。随后,将 30 μL NH4OH(28.0–30.0%,Sigma-Aldrich)加入到微乳液中。 24 小时后,通过离心(4500 rpm)分离悬浮液,然后在乙醇(98%,Sigma-Aldrich)和milliQ水中洗涤五次。然后将纳米颗粒分散在水中。
TiO2NPs的合成
TiO2NPs是按照Leena等人描述的溶胶-凝胶法制备的。 [30] 进行了一些修改。简而言之,在酸性条件下(pH 3)搅拌下,将异丙醇钛(IV)(TTIP;99.9% Sigma-Aldrich)滴入乙醇和milliQ水(5:1:1)的溶液中。 NPs先在30 °C孵育5 h,然后在430 °C孵育3 h,得到白色纳米粉末。
TEM 表征
透射电子显微镜 (TEM) 表征使用 JEOL Jem 1011 显微镜在 100 Kv (JEOL USA, Inc.) 的加速电压下运行。 TEM 样品的制备方法是将 NPs 的稀水溶液滴在碳包覆铜网格(Formvar/Carbon 300 Mesh Cu)上。
DLS 和 ζ-电位测量
SiO2NPs 和 TiO2NPs 的平均流体动力学尺寸和 zeta 电位是通过动态光散射 (DLS) 和 ζ 电位测量确定的,该测量在配备 4.0-mW HeNe 激光器的 Zetasizer Nano-ZS 上运行,工作波长为 633 nm 和雪崩光电二极管检测器(型号 ZEN3600,Malvern Instruments Ltd.,Malvern,英国)。在 25 °C 的水溶液和细胞培养基(DMEM,高葡萄糖,Sigma-Aldrich)中进行测量,该培养基补充有 FBS(Sigma-Aldrich),pH 值 7 为 10% 和 20%。每个样品运行 3 次,使用两个独立的技术重复,以获得 DLS 测量值和 ζ 电位的平均值。
XRD 表征
用于 TiO2NPs 晶相分析的粉末 X 射线衍射 (XRD) 在 Rigaku 衍射仪上使用过滤的 Cu-Ka 辐射在 Bragg-Brentano 反射几何结构中进行。通过步进扫描在2Q ¼ 20-80范围内记录XRD图谱,使用0.02的2Q增量和2 s/步的固定计数时间。
细胞培养
Caco-2 (ATCC® HTB-37™) 和 A549 (ATCC® CCL-185™) 保存在含有 50 μM 谷氨酰胺、100 U/mL 青霉素和 100 mg/mL 链霉素的 DMEM 中。 FBS 的百分比对于 A549 为 10%,对于 Caco-2 细胞为 20%。细胞在湿度控制的气氛中培养,空气/CO2 比例为 95% 到 5%,温度为 37 °C。
测定 SiO2NPS 和 TiO2 NPs 的细胞内摄取
10 5 Caco-2 和 A549 细胞接种在 1 mL 培养基中的六孔板中。在 37 °C 下孵育 24 小时后,将培养基更换为含有 SiO2NPs 和 TiO2NPs 的新鲜培养基,浓度分别为 15 μg/ml 和 45 μg/ml。 37 °C孵育48 h、72 h和96 h后,去除DMEM,用PBS(pH 7.4)洗涤细胞4次,去除可能与细胞膜结合的NPs。细胞被胰蛋白酶消化并使用自动细胞计数室进行计数。 36万个细胞悬浮在200 μL的milliQ中,并用HCl/HNO3 3:1 (v /v ) 并稀释至 5 mL:分析所得溶液以评估 Si 和 Ti 含量。元素分析采用电感耦合等离子体原子发射光谱(ICP-AES)和Varian Vista AX光谱仪。
WST-8 检测
Caco-2和A549细胞以5 × 10 3 的浓度接种于96孔板中 24 h 稳定后细胞/孔。将 NP 储备溶液(SiO2NPs 和 TiO2NPs)以 15 μg/ml 和 45 μg/ml 添加到细胞培养基中。将细胞孵育 24 小时、48 小时、72 小时和 96 小时。在终点,使用标准 WST-8 测定法 (Sigma-Aldrich) 确定细胞活力。按照先前在 De Matteis 等人中描述的程序进行检测。 [31]。数据表示为平均值 ± SD。
LDH 检测
Caco-2 和 A549 细胞按照 WST-8 测定报告的程序用 SiO2NPs 和 TiO2NPs 处理。按照制造商的说明,通过应用 CytoTox-ONE 均质膜完整性测定试剂 (Promega) 在微孔板上进行乳酸脱氢酶 (LDH) 测定。收集培养基,并使用 Bio-Rad 微孔板分光光度计通过读取 490 nm 处的吸光度来测量 LDH 的水平。数据表示为平均值 ± SD。
DCF-DA 分析
Caco-2 和 A549 细胞接种在 96 孔微孔板中,并用终浓度为 15 μg/ml 和 45 μg/ml 的 SiO2NPs 和 TiO2NPs 处理。在细胞-NP 相互作用 24 小时、48 小时、72 小时和 96 小时后,按照 De Matteis 等人报道的程序在微孔板上进行 DCF-DA (Sigma) 测定。 [32] 数据表示为平均值 ± SD。
SOD 检测
Caco-2 和 A549(用 15 μg/ml、45 μg/ml 孵育 24 h、48 h、72 h 和 96 h)细胞提取物根据 [33] 中描述的方案制备。通过应用测量所有三种类型的 SOD(Cu/ZnSOD、MnSOD 和 FeSOD)的 SOD 测定(Cayman Chemical Company, Michigan, OH, USA)在微孔板上进行该测定。该测定使用四唑盐来检测由黄嘌呤氧化酶和次黄嘌呤产生的超氧自由基。一个单位的 SOD 定义为显示超氧自由基 50% 歧化所需的酶量。使用Bio-Rad微孔板分光光度计通过读取440-460 nm处的吸光度来测量SOD活性。
MDA 检测
Caco-2 和 A549(用 15 μg/ml、45 μg/ml 孵育 24 h、48 h、72 h 和 96 h)细胞提取物根据之前描述的程序制备 [33]。该测定通过应用脂质过氧化 (MDA) 测定试剂盒 (Abcam) 在微孔板上进行:样品中的 MDA 与硫代巴比妥酸 (TBA) 反应生成 MDA-TBA 加合物。该路线涉及对 MDA-TBA 加合物形成过程中产生的红色进行分光光度测量,可通过使用 Bio-Rad 微孔板分光光度计读取 532 nm 处的吸光度进行定量(以 nmol/mg 蛋白质计)。
CLSM 分析
细胞接种于24孔板中,浓度为10 5 细胞/孔,并与浓度为 15 μg/ml 和 45 μg/ml 的 SiO2NPs 和 TiO2NPs 连续孵育 24 h、48 h、72 h 和 96 h。处理后,对于每个时间点,去除含有纳米颗粒的培养基,用PBS洗涤细胞3次,用0.25%戊二醛(v /v PBS, Sigma-Aldrich) 20 分钟,最后用 0.1% Triton (v /v 在 PBS 中,Sigma-Aldrich) 5 分钟 对于肌动蛋白染色,鬼笔环肽-ATTO 488 (Sigma-Aldrich) 以 1 μg/ml 的浓度使用 30 分钟。通过 DAPI (Sigma-Aldrich) 以 1 μg/ml 的浓度标记细胞核 7 分钟。激光扫描共聚焦显微镜在配备 Axio Observer Z1 (Zeiss) 倒置显微镜的 Zeiss LSM700 (Zeiss) 共聚焦显微镜上进行,使用 × 100, 1.46 数值孔径油浸透镜进行成像。使用 ZEN2010 软件 (Zeiss) 处理共聚焦数据文件,并使用 ImageJ 1.47 分析软件对 15 个细胞进行形态计量学量化(F-肌动蛋白的一致性和积分密度)。 OrientationJ 插件用于基于局部邻域中结构张量的测量,通过在共聚焦采集中选择特定的 ROI 序列来量化相干性参数。同时,软件计算了代表肌动蛋白纤维取向程度的取向和相干性值:更无序的纤维具有接近 0 的值,而完全对齐的纤维的相干性值约为 1 [34]。为了量化细胞中肌动蛋白纤维的数量,还通过共聚焦采集的 ROI 中像素值的总和计算了积分密度。
原子力显微镜分析
Caco-2 和 A549 细胞以 10 5 的浓度接种在塑料培养皿(Corning)中 细胞/孔并生长至 70-80% 的汇合。然后在 DMEM 中用 45 μg/ml 的 TiO2NPS 和 SiO2NPs 处理细胞 72 h。随后,去除 NPs,并用 PBS 洗涤细胞。细胞用 0.25% 戊二醛固定 20 分钟,然后用 PBS 洗涤。通过安装在倒置光学显微镜(Zeiss Observer Z1,Zeiss GERMANY)上的先进扫描探针显微镜(Bioscope Catalyst,Bruker Inc.,USA)进行测量。整个系统放置在一个基座上,该基座充当环境机械振动的绝缘体。 AFM 实验是通过使用 V 形布鲁克的夏普微杠杆(MSNL,尖端 C)在力-体积模式下进行的:高灵敏度氮化硅悬臂,标称弹簧常数为 0.01 N/m。该值是在执行 AFM 采集之前通过热调谐方法 [35] 准确估计的。使用的参数如下:扫描面积 50 μm,斜率 3 Hz,FV 扫描速率 0.03 Hz,触发阈值 100 nm,样品数量 128,每行样品 64 和 64。 20 个细胞,从中提取 25 条力-距离曲线对应于核面积和 25 条细胞质区域曲线。从提取的曲线导出的接近数据(从接触点到最大力值)集与修改后的 Sneddon 模型拟合:
$$ -{k}_{\mathrm{c}}{\delta}_{\mathrm{c}}=\frac{2 Etg\alpha}{\pi \left(1-{\nu}^2\ right)}{\left(z-{\delta}_{\mathrm{c}}\right)}^2 $$其中 z 和δc 是实验加载数据(分别为高度和悬臂挠度),α 是尖端的半角,k c为悬臂的弹性常数值,ν 是泊松比(假设生物样品为 0.5)。在拟合算法中,将接触点视为拟合变量,并考虑了对20个细胞的粘附力。
统计分析
数据表示为平均值和相关标准偏差。执行 Student t 时,不同平均值之间的差异被认为具有统计学意义 用 p 测试 值˂ 0.05(<0.05*、<0.01**和<0.005***)。
结果
SiO2NPs 和 TiO2NPs 的表征
SiO2NPs 和 TiO2NPs 已经用不同的和可重复的合成路线合成,以获得具有窄且可控的尺寸分布的 NPs(参见“方法”部分)。然后,通过 TEM、DLS、ζ 电位和 XRD,在水中和具有不同浓度蛋白质源 (FBS) 的细胞培养基 (DMEM) 中对 NPs 进行深入表征。这是至关重要的,因为培养基蛋白可以覆盖 NPs 表面,从而改变它们的理化特性,从而改变生物学效应 [36]。 TEM 分析表明 SiO2NP 呈球形,平均直径为 20 ± 2 nm(图 1a)。 TiO2NPs 具有相似的尺寸 (25 ± 5 nm),但形态不同(图 1)。在水中 96 h 进行的 DLS 测量证实,SiO2NP 和 TiO2NP 的流体动力学半径分别为 21 ± 7 nm 和 27 ± 12 nm(图 1b 和图 1e)。正如预期的那样,这些数据与 TEM 观察结果非常吻合。 ζ-电位分析还证实了水中 SiO2NPs 的表面电荷值为 − 45 ± 3mV,TiO2NPs 为 − 50 ± 3mV(图 1c、f)。正如预期的那样,NPs 的理化特性在细胞培养基中接种后发生了变化。 DLS 证实了 NP 大小的显着增加,尤其是在存在补充有 20% FBS 的 DMEM 的情况下(表 1)。特别是,SiO2NPs 的尺寸为 29 ± 9 nm,而 TiO2NPs 在 96 h 后增加到 41 ± 14 nm。在 DMEM 测量中观察到的 DLS 峰的扩大(有或没有 FBS)是 NPs 团聚的标志,这可以通过介质的离子强度来促进(数据未显示)。此外,ζ 电位测量表明两个 NPs 的表面电荷转移到更多的负值。 这种大的时间依赖性现象是由于细胞培养基中吸附在 NPs 表面的血清蛋白的存在诱导了相当稳定的蛋白质电晕形成 [37, 38]:NPs 的大小和电荷随函数变化FBS 浓度。
<图片>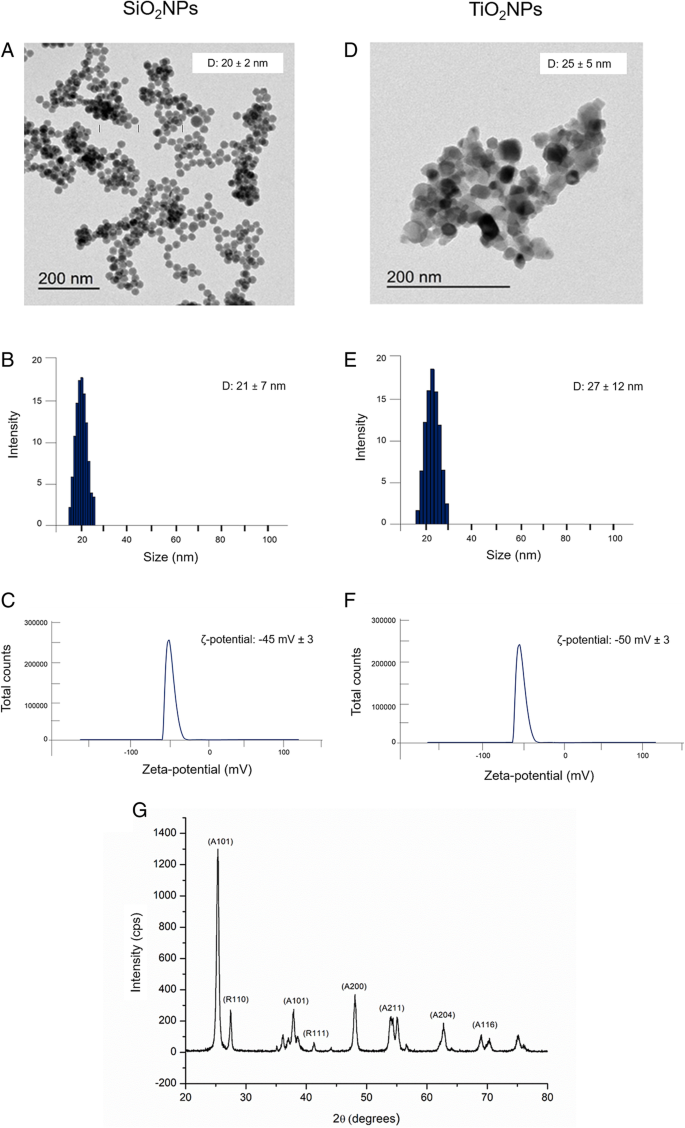
SiO2NPs 和 TiO2NPs 在水中的表征。 一 –d 代表性 TEM 图像。 b –e 动态光散射 (DLS) 和 c –f ζ-电位测量。 g TiO2NPs的X射线衍射分析(XRD)图
图> 图>在 430 °C 下煅烧的 TiO2NPS 的 XRD 图显示锐钛矿和金红石晶相的混合物(图 1g) .在 2θ =25.4° (101)、48.1° (200)、54.1° (211)、62.4° (204) 和 68.8° (116) 处的主要峰是锐钛矿相位与标准 JCPDS 数据匹配良好的特征(卡号:21–1272)。金红石相在27.5°(110)、36.2°(101)和41.2°(111)处出现衍射峰。
Caco-2 和 A549 细胞中纳米颗粒的摄取
为了量化细胞吸收的 SiO2NPs 和 TiO2NPS 的数量,我们对裂解的细胞进行了 ICP-AES 元素分析作为初步调查。细胞用 15 μg/ml 和 45 μg/ml 的 NPs 处理。实验数据证实了两种细胞系中都存在 SiO2NPs 和 TiO2NPs,具有时间依赖性内化效率(图 2a)。与 SiO2NPs 相比,TiO2NPs 表现出更大的吸收。这在 Caco-2 中尤为明显,其中 Ti 含量在 72 小时和 96 小时后分别达到 8.2 ± 0.4 微克和 9.7 ± 0.031 微克。 在 A549 中检测到的 Ti 量较低,因为我们发现在 72 小时后为 5 ± 0.599 微克,在 96 小时的孵育时间后为 7.12 ± 0.11 微克。与 TiO2NPs 相比,SiO2NPs 被细胞吸收的更少,即使 Caco-2 的内化更明显。同样在这种情况下,事实上,Caco-2 细胞中内化 SiO2NP 的量在 72 小时后为 4.69 ± 0.031 μg,在孵育 96 小时后为 5.78 ± 0.045 μg。 A549 中的值下降,我们在 72 h 后量化为 2.58 ± 0.045 μg,在 96 h 后量化为 4.7 ± 0.04 μg。
<图片>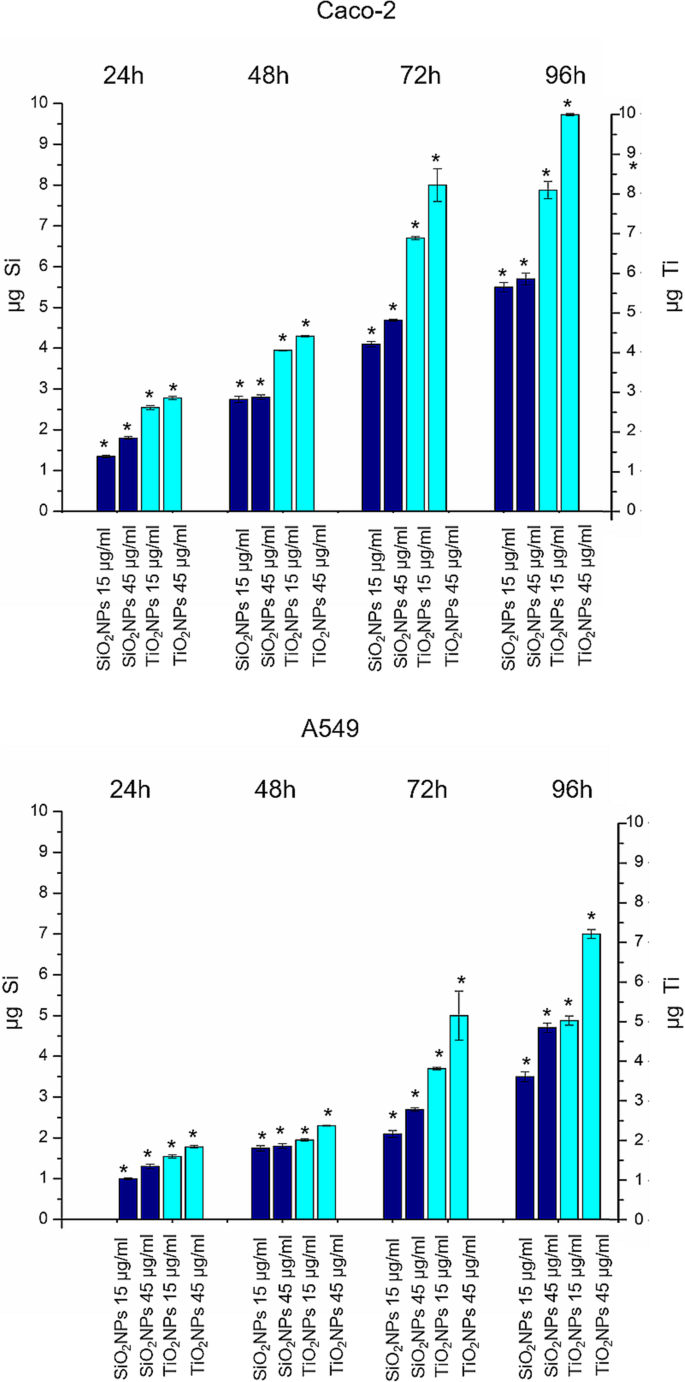
TiO2NPs 和 SiO2NPs 在 Caco-2 和 A549 细胞系中的积累,暴露于 15 μg/ml 和 45 μg/ml 的 TiO2NPs 和 SiO2NPs 24 h、48 h、72 h 和 96 h。然后收获细胞,对活细胞进行计数,并测量 360,000 个细胞(μg Ti 和 μg Si)中的 Ti 和 Si 含量。数据报告为来自三个独立实验的平均值 ± SD; p 暴露细胞与对照细胞的统计显着性 值 <0.05(<0.05*、<0.01** 和 <0.005***)
图>纳米颗粒对 CaCo-2 和 A549 的影响:细胞活力、膜损伤和 ROS 产生
Caco-2 和 A549 细胞活力用 WST-8 测定法评估。用 SiO2NPs 和 TiO2NPs 处理在测试的两种细胞系中都引起了轻微的剂量依赖性降低(图 3)。 TiO2NPs 对 SiO2NPs 的细胞毒性增强,在用 TiO2NPs 处理后,CaCo-2 细胞的细胞活力比 A549 受到的影响更大。特别是,我们观察到在用 45 μg/ml TiO2NPs 处理 72 h 的 Caco-2 中存活率降低了约 40%。这种降低在 96 小时后进一步下降至 50%,而在 A549 细胞系中,TiO2NPs 仅在处理 96 小时后才导致存活率降低 30%。在暴露于 TiO2NPs 和 SiO2NPs 后,评估了 Caco-2 和 A549 细胞中 LDH 的释放和 ROS 的产生。如图 4a、b 所示,NPs 诱导细胞膜穿孔(和 LDH 确实释放)与活力结果非常一致。对于 A549,Caco-2 中的效果更明显,尤其是在 TiO2NP 处理时,在最高时间点(72 和 96 h)。在暴露 96 h 后,LDH 释放百分比相对于未处理(对照)细胞增加了约 160%。 ROS 的产生已被广泛研究,因为它是 NPs 诱导的主要影响之一 [39]。这种现象会干扰生物抗氧化防御反应 [40],尽管重要的是要提到真正的作用机制仍在研究中。通过 DCFH-DA 测定估计潜在的 NP 诱导的氧化应激。正如预期的那样,NPs 和细胞之间的相互作用以剂量依赖性方式刺激了 ROS 的产生,对 Caco-2 对 TiO2NP 处理产生了强烈影响(图 4c、d)。在测试的最高浓度下,相对于对照细胞的释放百分比达到了165%。
<图片>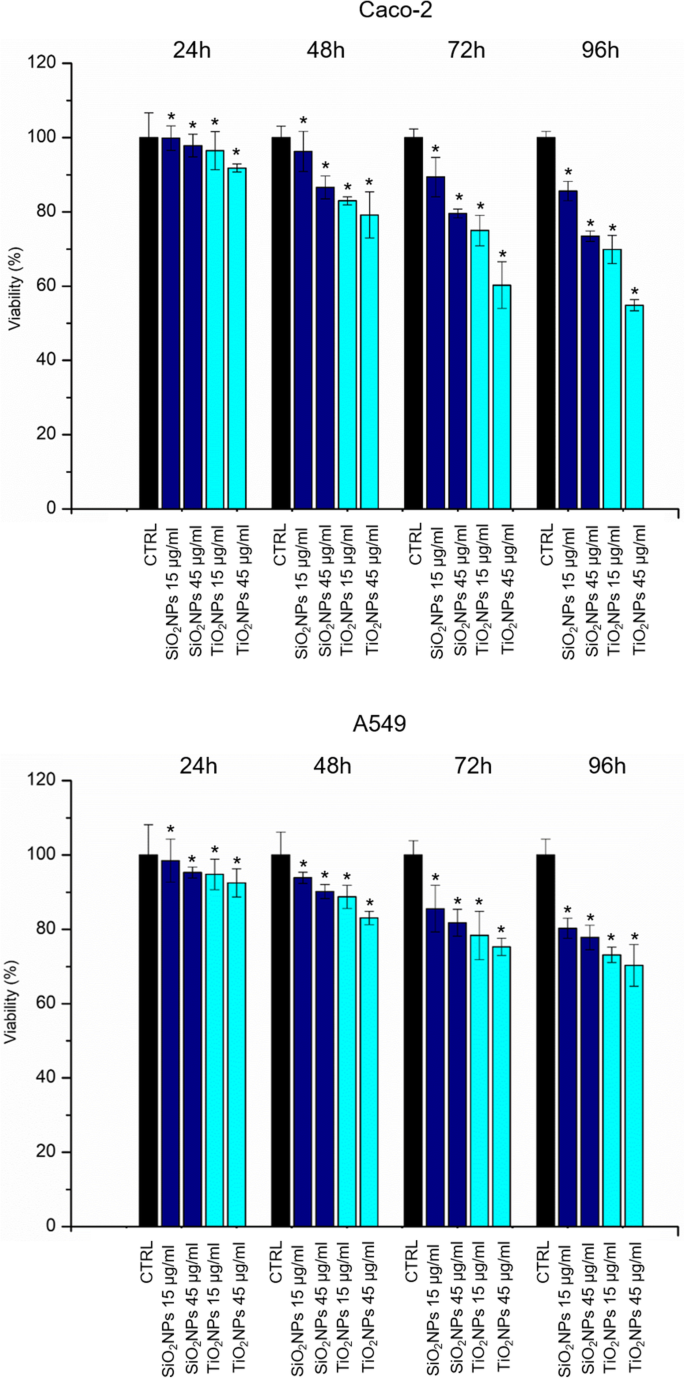
Caco-2 (a) 的活力测定 (WST-8) ) 和 A549 (b ) 细胞暴露于两种剂量(15 μg/ml 和 45 μg/ml)的 TiO2NPs 和 SiO2NPs 后 24 h、48 h、72 h 和 96 h。 NP 处理的细胞的活力被归一化为未处理的对照细胞。作为阳性对照 (P),细胞与 5% DMSO 一起孵育(数据未显示)。与对照相比,三个独立实验中报告为平均值 ± SD的数据被认为具有统计学意义(n =8) 对于 p 值 <0.05(<0.05*、<0.01** 和 <0.005***)
图> <图片>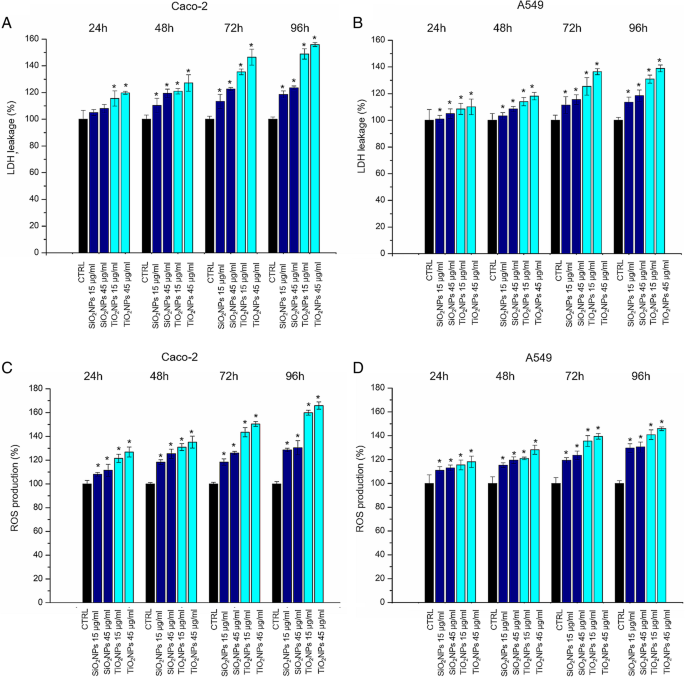
LDH (a –b ) 和 ROS (c –d ) 对 Caco-2 和 A549 细胞的检测。将细胞与 15 μg/ml 和 45 μg/ml 的 TiO2NPs 和 SiO2NPs 孵育 24 h、48 h、72 h 和 96 h。纳米颗粒处理的细胞的 LDH 泄漏百分比表示相对于未处理的对照细胞。阳性对照 (P) 包括用 0.9% Triton X-100 处理细胞,显示约。 500% LDH 增加(数据未显示)。记录 ROS 水平,将 Caco-2 和 A549 细胞暴露于 15 μg/ml 和 45 μg/ml 的 TiO2NP 和 SiO2NP,与 100 DAμM DCFH-孵育 24 小时、48 小时、72 小时和 96 小时。测量细胞荧光。作为阳性对照 (P),将细胞与 500 μM H2O2 一起孵育,显示大约300% DCFH-DA 增加(未显示)。与对照相比,三个独立实验中报告为平均值 ± SD的数据被认为具有统计学意义(n =8) 对于 p 值 <0.05(<0.05*、<0.01** 和 <.005***)
图>纳米颗粒对 Caco-2 和 A549 细胞抗氧化活性和脂质过氧化的影响
SOD酶参与氧化应激诱导后的抗氧化防御系统。这种酶催化高反应性超氧化物 (O2 •− ) 阴离子转化为过氧化物 H2O2 [41]。我们观察到在不同时间点(从 24 到 96 h)与 SiO2NPs 和 TiO2NPs(15 μg/ml,45 μg/ml)孵育后 Caco-2 和 A549 中 SOD 酶活性的剂量依赖性降低(图 5a) , b)。与细胞毒性评估非常一致,Caco-2 对 TiO2NP 暴露的影响更为明显。例如,在暴露于 45 μg/ml TiO2NPs 的 Caco-2 细胞中,在 96 h 后,SOD 活性水平从对照中的 4.1 ± 0.2 U/ml 降低到 1.03 ± 0.325 U/ml。暴露于相同浓度的 SiO2NPs 将 SOD 活性降低至 1.45 ± 0.12 U/ml。基于 MDA 的测定用于检查潜在的 ROS 介导的脂质过氧化,这反过来又是检查细胞氧化应激的另一种方法。 [42] 在暴露于 Caco-2 和 A549 的两种类型的 NP 后,MDA 的细胞水平增加(图 5c,d)。正如预期的那样,增加的 MDA 水平与浓度和暴露时间成正比。
<图片>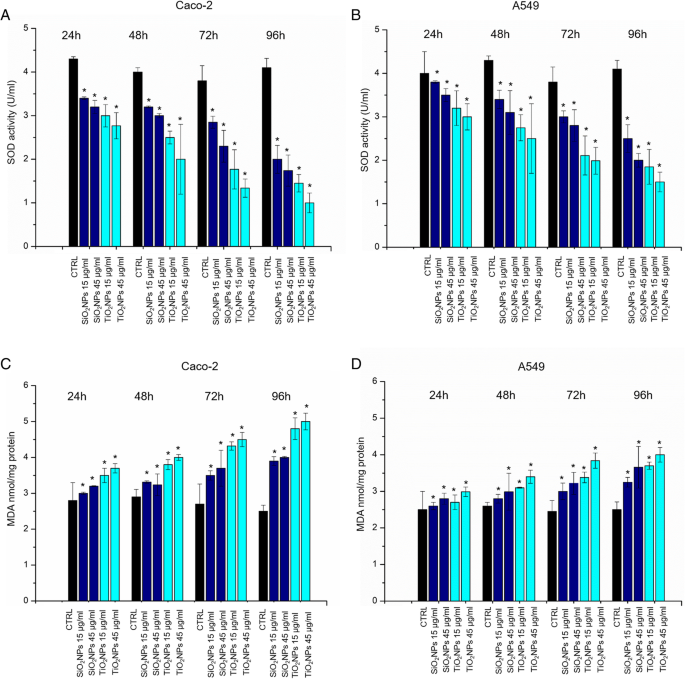
一 –d Caco-2 和 A549 细胞上的 SOD 和 MDA 测定。将细胞与 15 μg/ml 和 45 μg/ml 的 TiO2NPs 和 SiO2NPs 孵育 24 h、48 h、72 h、96 h。 SOD 测定使用四唑盐来检测由黄嘌呤氧化酶和次黄嘌呤产生的超氧自由基。标准曲线用作阳性对照(数据未显示)。 MDA 水平通过定量 MDA-TBA 加合物 (OD =532 nm) 来检测。与对照相比,三个独立实验中报告为平均值 ± SD的数据被认为具有统计学意义(n =8) 对于 p 值 <0.05(<0.05*、<0.01** 和 <0.005***)
图>NPs 引起的形态力学效应
Caco-2 和 A549 与 15 μg/ml 和 45 μg/ml 的 SiO2NPs 和 TiO2NPs 孵育 24 h、48 h、72 h 和 96 h 的共聚焦显微镜分析报告在图 1-2 中。 6 和 7. 对照 Caco-2 细胞表现出类似于肠肠细胞的形态,在顶端具有紧密连接和刷状缘 [43]。用 NPs 处理后,细胞的紧密连接坍塌,细胞的图案被隔离,形状拉长。当细胞用 45 μg/ml 的 TiO2NPs 处理 72 小时的孵育时间时,这些影响更加明显,显示出肌动蛋白模式的相关改变,以及细胞形态的变化。未处理的 A549 细胞显示出卵石状形状和功能性细胞 - 细胞粘附 [44],而用 NPs 处理减少了细胞 - 细胞接触并将细胞形态改变为更细长的形状。图 8 显示了一个放大的共焦图,它可以检测肌动蛋白分布的变化。使用 ImageJ 软件通过荧光密度和相干性对 NP 暴露后肌动蛋白网络组织的改变(72 h 45 μg/ml SiO2NPs 和 TiO2NPs)进行定量分析(图 9)。我们特别选择了这两个参数,因为积分密度允许我们量化肌动蛋白的数量,而相干性为我们提供了与周围环境相比纤维取向程度的信息 [45]。未处理的 Caco-2 细胞的密度值为 129.4 ± 16,这不受 NP 处理的影响;在暴露于 SiO2NPs 和 TiO2NPs 后,这些值分别为 127.7 ± 20 和 128.5 ± 18(图 9a)。类似地,处理前后 A549 中肌动蛋白染色网络的密度也保持不变(分别为 68.4 ± 14、67.9 ± 15 和 67.7 ± 18,分别用于阴性对照、SiO2NP 和 TiO2NP,图 9b)。虽然 NP 处理不会引起肌动蛋白量的改变,但一致性分析表明不同的肌动蛋白空间重组。在 Caco-2 中,SiO2NP(0.16 ± 0.04)和 TiO2NP(0.09 ± 0.02)处理的细胞的相关性值相对于对照(0.26 ± 0.03)降低(图 9c)。与对照细胞 (0.4 ± 0.03) 相比,即使是 A549 细胞在与 SiO2NPs 和 TiO2NPs 相互作用后也经历了相干性的降低(0.2 ± 0.07 和 0.158 ± 0.04)(图 9d)。因此,NPs 诱导了肌动蛋白网络的显着重组,显示出肌动蛋白各向同性的方向,但它们并没有改变表达的肌动蛋白的总量。除了细胞骨架重排,我们还分析了由细胞核/细胞质比描述的区域。 N/C 比值计算为核面积与整个细胞面积之间的比率(对 15 个细胞进行测量)。我们在用 45 μg/ml NPs 处理 72 h 后观察到相反的值,具有显着的统计有效性。特别是,在未处理的 Caco-2 细胞中,该比率为 (0.40 ± 1.7),在 SiO2NP 和 TiO2NP 暴露后,该比率增加到 0.554 ± 0.09 和 0.62 ± 0.12(图 9e)。 A549 的趋势不同。 The nuclear/cytoplasm ratio dropped down upon exposure to NPs from values of 0.550 ± 0.04 for control cells to 0.334 ± 0.06 for SiO2NPs and 0.225 ± 0.09 for TiO2NPs. After the morphological investigations, we analyzed the elastic properties of cells after exposing them to 45 μg/ml of SiO2NPs and TiO2NPs for 72 h by AFM, in force–volume mode. We measured the different elasticity expressed by Young’s modulus values in the nuclear and cytoplasmic region. Caco-2 cells displayed Young’s modulus value of 105 ± 25 kPa for nuclear region and 47 ± 21 kPa for the cytoplasm. After SiO2NP treatment, we observed a reduction of value to 42 ± 8 kPa for the nucleus and 19.59 ± 2 kPa for the cytoplasm. The effects were more evident after treatment with TiO2NPs:the Young modulus for the nucleus was 27 ± 4 kPa and 18 ± 4 kPa for the cytoplasm (Fig. 10a). We found an opposite outcome concerning the elastic properties of A549 cells. In this case, Young’s modulus was 129 ± 24 kPa for the nuclear region and 147 ± 26 kPa for the cytoplasmic area. After SiO2NP treatment, the values of elasticity increased to 152 ± 23 kPa for nucleus and 152 ± 25 kPa for cytoplasm. When cells were doped with TiO2NPs, Young’s modulus values drastically increased to 372 ± 60 kPa for nucleus region and 549 ± 40 kPa for cytoplasmic region (Fig. 10b).
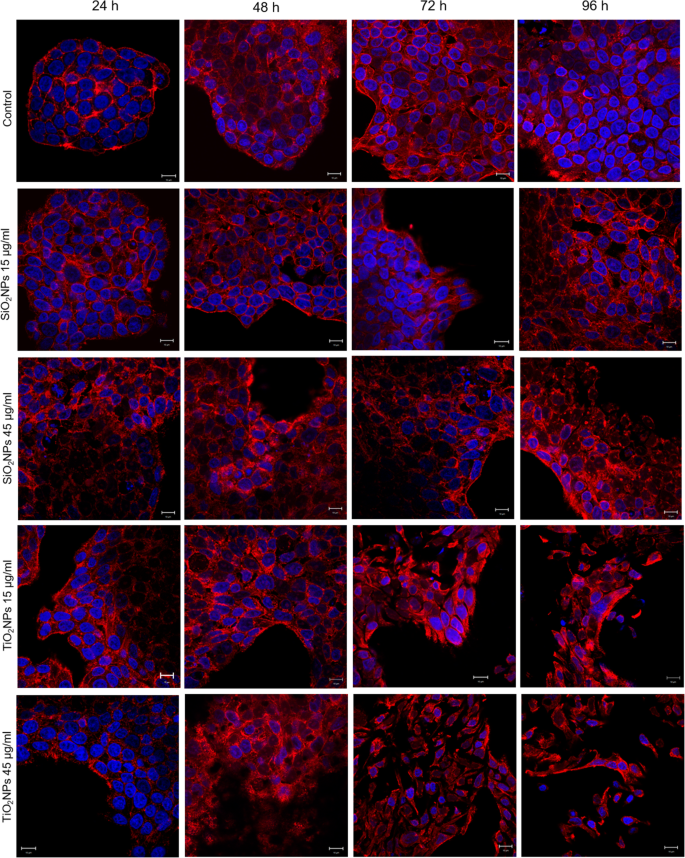
Effects of SiO2NPS and TiO2NPs on actin network of Caco-2 cells. Caco2 were treated with 15 μg/ml and 45 μg/ml of NPs for 24 h, 48 h, 72 h, and 96 h; cells were fixed and then stained with Phalloidin–ATTO 488 and DAPI. The 2D images of cortical actin were acquired by a Zeiss LSM700 (Zeiss) confocal microscope equipped with an Axio Observer Z1 (Zeiss) inverted microscope using a × 100, 1.46 numerical aperture oil immersion lens. All data were processed by ZEN software (Zeiss)
图>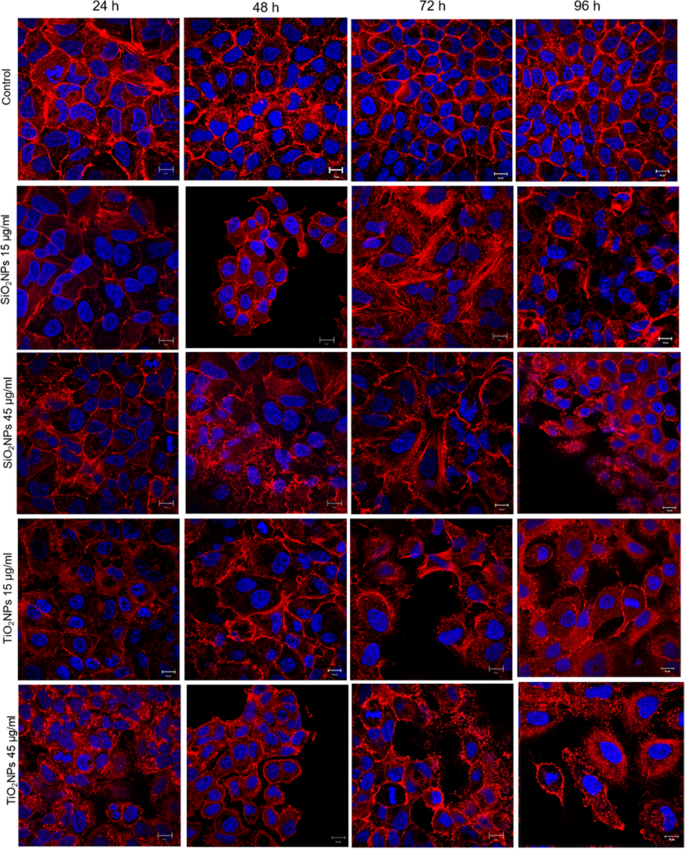
Effect of SiO2NPS and TiO2NPs on actin network on A549 cells. A549 were treated with 15 μg/ml and 45 μg/ml of NPs for 24 h, 48 h, 72 h, and 96 h; successively they were fixed and stained with Phalloidin–ATTO 488 and DAPI. The 2D images of cortical actin were acquired by a Zeiss LSM700 (Zeiss) confocal microscope equipped with an Axio Observer Z1 (Zeiss) inverted microscope using a × 100, 1.46 numerical aperture oil immersion lens. All data were processed by ZEN software (Zeiss)
图>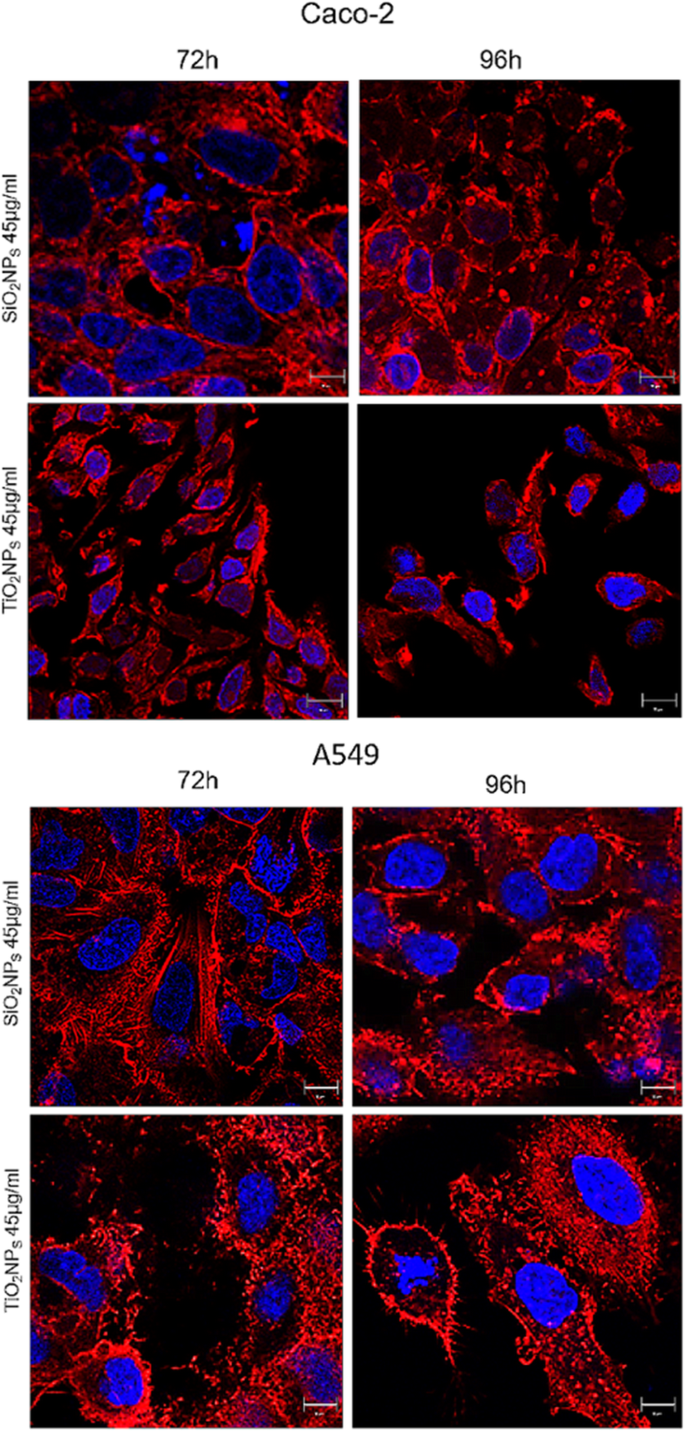
Local enlargement of confocal acquisitions acquired in Figs. 6 and 7 showed (more in details) the effect of SiO2NPS and TiO2NPs on actin network of Caco-2 and A549 cells after the exposure of 45 μg/ml of NPs for 72 h and 96 h
图>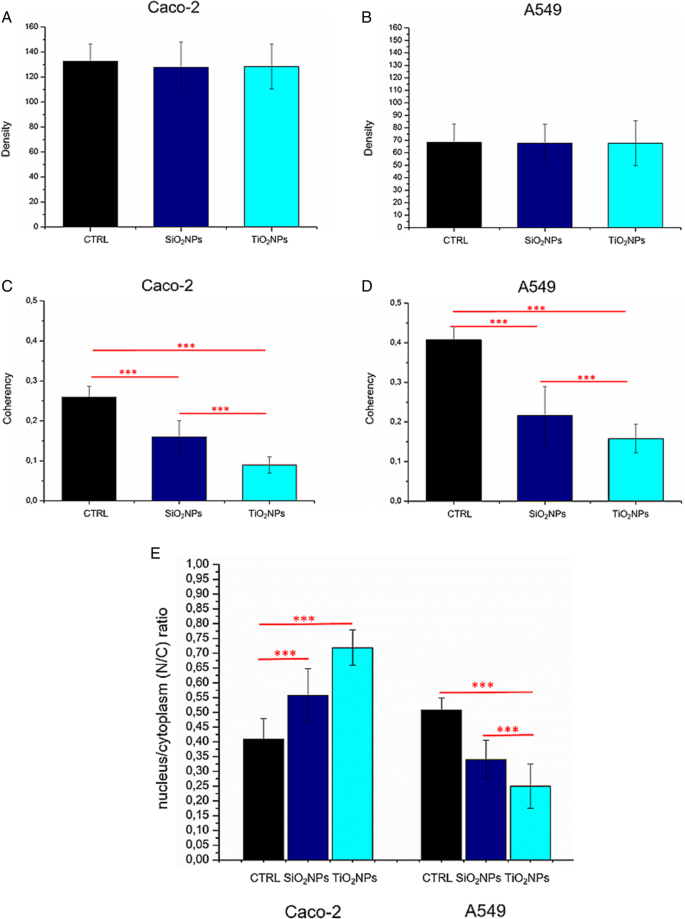
Integrated density (a , b ) and coherency (c , d ) for Caco-2 and A549 cells treated with 45 μg/ml of SiO2NPs and TiO2NPs after 72 h. The integrated density and coherency values were expressed as a mean value and relative SD, calculated from confocal acquisitions by ImageJ (calculation on 15 cells). The mean values and their standard deviations were reported in the histograms. Results were statistically significant for p < 0.05 (< 0.05*, < 0.01**, and < 0.005***). e Analyses of nucleus/cytoplasm ratio on Caco-2 and A549 after exposure to 45 μg/ml of SiO2NPs and TiO2NPs for 72 h. The ratio was calculated on 15 cells by ImageJ. The mean values and the SD were reported in the histogram. Results were statistically significant for p < 0.05 (< 0.05*, < 0.01**, and < 0.005***)
图>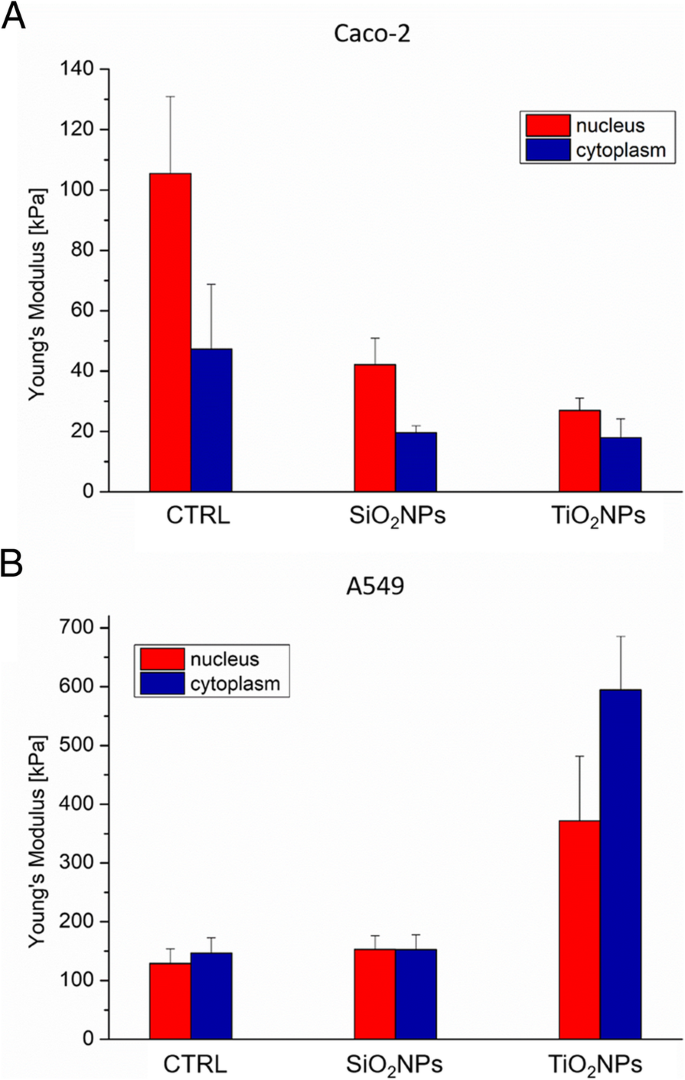
Young’s modulus values expressed in kPa, calculated from the nuclear region and the cytoskeletal area of Caco-2 (a ) and A549 (b ) cell lines after a treatment to 45 μg/ml of SiO2NPs and TiO2NPs for 72 h
图>讨论
The spread of different kind of ENPs in several fields raises awareness about the importance to assess their potential toxicity in living organisms and the environments as well, taking into account their potential application in biomedical field [46,47,48]. In vitro and in vivo investigations are crucial to enrich the scientific knowledge and to release reliable clinical and epidemiological data [49]. The toxicity tests performed on different cells are considered the golden standard to assess the safety of NPs. However, few studies have investigated the interactions between NPs and cells from a biomechanical point of view. Cell mechanic is an important factor that influences many cellular pathways, including apoptosis, differentiation, migration, cancer metastasis, and wound healing [50]. In our work, we have addressed this point and related cell viability with the changes in mechanical properties of cells treated with different NPs. Firstly, we synthetized amorphous SiO2NPs and crystalline TiO2NPs with a size of c.a. 20 nm. NPs were stable in water and DMEM up to 96 h, even upon incubation with 10% and 20% of FBS. This was found to induce an increase in NPs size due to the formation of protein corona, in perfect agreement with the literature data. [51]. Since the entry route of NPs often occurs through inhalation and ingestion, we opted to investigate the potential effects on Caco-2 and A549 cells, which are representative models for the intestinal tract and airways mimicking oral and inhalation uptake [52]. As primary investigation, we quantified the cellular internalization of SiO2NPs and TiO2NPs by elemental analysis. The most effective uptake was observed in Caco-2 cells, especially upon treatment with TiO2NPs in a time-dependent manner. It has been reported that amorphous SiO2NPs, with a small size range of 15–20 nm, can bind the plasma membrane and then passively pass across the lipid bilayer to get access into the cells [53]. As demonstrated in A549 [54] and Caco-2 [55], in fact, small SiO2NPs can translocate in the cytoplasm with no apparent membrane encapsulation. The anatase crystalline form of TiO2NPs is the more chemically reactive [56] showing a faster absorption with respect to rutile, as previously reported [32]. However, the uptake mechanisms of Caco2 are still unclear, despite that some hypothesis have been formulated, some of these include metal ion release upon NPs degradation in the intestinal barrier lumen or/and direct uptake by endocytosis. [57]. In A549 cells, TiO2NPs were localized in cytoplasm and close the nucleus region [58]. We used WST-8 assay to assess the influence of different concentrations of SiO2NPs and TiO2NPs on cell viability. We have observed a general decrease of viability, especially in Caco-2, with TiO2NPs displaying the strongest toxicity. After assessing the viability, we monitored the extracellular release of the cytoplasmic enzyme LDH. We confirmed that the NPs induced an extensive membrane damage, which relates also to the increase of intracellular ROS levels, resulting in oxidative stress. In this context SOD, which acts as strong antioxidant against ROS [59], was significantly reduced most probably because of the unbalance of the redox repair systems. In addition, the oxidative stress increased the lipid peroxidation [60], as demonstrated by MDA measurements after NPs incubation. This is particularly evident in Caco-2 cells after TiO2NP exposure. It is worth mentioning that this effect can decrease membrane fluidity, which can further explain the observed higher entry levels of the TiO2NPs [61]. This was in significant accordance with the intracellular oxidative stress levels measured by SOD inhibition, as well as with the reactive oxygen species generation. After these assessments, we investigated the modulation of the cell cytoskeleton, as an increase of intracellular ROS could affect the F-actin organization [62]. The cytoskeleton is characterized by a set of filaments (actin microfilaments, microtubules, and intermediate filaments) organized in a network that affects the mechanical properties of cells, as well as their behavior [29]. In particular, actin filaments are crucial for cell mechanics, and any alterations may induce aberrations in cell morphology under sub-toxic conditions [63]. It has been demonstrated that actin was one of the most commonly bound protein by SiO2NPs and TiO2NPs in cellular extracts. This definitely suggests that the actin functions, as well as cell motility and organelles trafficking, can be strongly affected by the presence of these NPs [64, 65]. As a further proof, several in vivo studies have revealed the potential of NPs to induce alterations in the expression of genes related to the cytoskeleton [63]. In order to understand how NPs modulate the cytoskeleton, we performed qualitative and quantitative confocal analyses on Caco-2 and A549 cells, after SiO2NP and TIO2NP treatment. We focused on actin stress fibers and cortical actin because they allowed to maintain the physiological mechanical architecture of cells. As reported in Figs. 4 and 5, the treatment with NPs induced a significant reorganization of actin. This was more evident after 72 h of treatment with 45 μg/ml of NPs, and especially with the use of TiO2NPs. The adverse effects were stronger in intestinal cells, where we have observed the formation of protrusions and philopodia at the plasma membrane level, together with the disruption of tight junctions. Fluorescence coherency and fluorescence density have been used as quantitative parameters to assess alterations of actin distribution in the cytoskeleton. While coherency gives information about the organization of actin, density quantifies the levels of fluorescent actin. Caco-2 and A549 exposed to NPs showed a reduction of coherency compared to untreated cells, especially upon incubation with TiO2NPs. This was in good agreement with the qualitative confocal imaging analyses. The fluorescence density of actin was not altered by NP treatment in both the cell lines, even if untreated Caco-2 cells showed higher values with respect to untreated A549. These data could suggest a potential difference in the amount of actin, which is dependent on>the specific cell type. We also evaluated the nucleus-to-cytoplasm ratio as the relative area of the nucleus over the whole cell. We confirmed a reduction of values in A549 and an increase of the ratio in Caco-2 with respect to the control cells. This indicates changes in cell morphology after NP treatment:Caco-2 underwent an increase of nucleus area, whereas A549 became larger through cytoplasm extension. As a final point, we explored any potential change in cell elasticity upon NP treatment. Cell elasticity is commonly expressed by Young’s modulus (E), which is a ratio between the stress and the applied strain (with unit in Pascal) [66, 67]. Changes in cell elasticity due to cytoskeleton reorganization is often associated to disease progression [68], hence (E) can be a refined indicator of potential pathological states [67]. The deformability of cells was measured through indentation experiments by AFM [69]. Many studies showed the detrimental effects of NPs on the F-actin that induced an enhancement of cell elasticity. However, a clear relation between change in cell stiffness, actin rearrangement and cell viability has not been clearly established yet. Here, we have covered such topic and found that Caco-2 and A549 cells significantly change their (E) upon NP treatment, even though in two different ways. Caco-2 cells are softer as confirmed by the decreased Young’s modulus, which has been found to be a function of both the NPs type and the cell regions analyzed. In particular, TiO2NPs induced a general enhancement of elasticity, and this effect is more evident in the nuclear regions rather than the cytoplasmic one. On the other side, A549 displayed a remarkable increase of Young’s modulus after TiO2NP exposure in cytoplasm region, compared to control cells (594 ± 40 kPa versus 146 ± 26 kPa, respectively). These data indicated that TiO2NPs induce dose-dependent changes in force–deformation profiles in both cell lines, whereas SiO2NPs showed little effects. The decrease of Young’s Modulus, and consequently an increase of elasticity after NPs exposure, can potentially impact cell homeostasis and physiological pathways. The reorganization F-actin, together with a reduction of coherency, showed a strong modulation of mechanical cell properties. NPs have been demonstrated to make the nuclear region of Caco-2 cells softer than untreated cells. The increase of elasticity (corresponding to a reduction of Young’s modulus) is a critical factor in tumor progression, because it is an indicator of disruption of cell junctions, which promotes in turn cell migration and metastatization [70]. Therefore, the treatment with NPs on Caco-2 (and TiO2NPS in particular) can potentially promote migration due to change of elastic properties and deformability of cells. Also, the larger and softer nucleus area can be associated to cancer progression [71].
结论
In this paper, we careful assessed the adverse effects of SiO2NPs and TiO2NPs on two different cell lines (Caco-2 and A549), mimicking the typical tissue that are exposed to NPs (ingestion and inhalation). SiO2NPs presented a low cytotoxicity in comparison with TiO2NPS. We demonstrated how the cellular exposure to high doses of NPs induced morphostructural changes in term of actin reorganization, coherency, density and nucleus/cytoplasm ratio, which were more evident upon TiO2NP treatment. Cell membrane deformability measurements showed different behavior in the two cells. In Caco-2, the cell elasticity increased after NP treatment, whereas A549 displayed an increase of stiffness. These results demonstrated that NPs induce modifications of cytoskeleton structures and, as consequence, a different Young’s Modulus values. Hence, the phenotype of cancer cells can turn into a more invasive profile, characterized by enhanced migration. On the other side, the increased stiffness observed in A549 might not promote the mobility of this specific cell indeed. We are sure that the analysis of cell mechanics upon NP exposure, combined with standard toxicological assays, will represent a golden standard to accurately assess the safety of NPs and to predict any potential possible diseases triggered by NPs.
缩写
- A549:
-
Human adenocarcinoma alveolar basal epithelial cells
- 原子力显微镜:
-
原子力显微镜
- ATP:
-
Adenosine triphosphate
- Caco-2:
-
Human epithelial colorectal adenocarcinoma
- CLSM:
-
Confocal Laser Scanning Microscopy
- DAPI:
-
4′,6′-Diamidino-2-phenylindole
- DCF-DA:
-
2′,7′-Dichlorofluorescein diacetate
- DLS:
-
动态光散射
- DMEM:
-
Dulbecco’s modified Eagle’s medium
- ENPs:
-
Engineered nanoparticles
- FBS:
-
胎牛血清
- HCl/HNO3:
-
Hydrochloric/nitric acid
- ICP-AES:
-
Inductively coupled plasma atomic emission spectroscopy
- LDH:
-
Lactate dehydrogenase
- MDA:
-
Malondialdehyde
- NH4OH:
-
Ammonium hydroxide
- NP:
-
纳米粒子
- PBS:
-
Phosphate Buffered Saline
- ROIs:
-
Regions of interest
- ROS:
-
Reactive Oxygen Species
- SiO2NPs:
-
Silicon dioxide nanoparticles
- SOD:
-
超氧化物歧化酶
- TBA:
-
Thiobarbituric acid
- TEM:
-
透射电子显微镜
- TEOS:
-
原硅酸四乙酯
- TiO2NPS:
-
Titanium dioxide nanoparticles
- TTIP:
-
Titanium (IV) isopropoxide
- XRD:
-
X射线衍射
纳米材料
- 用贵金属纳米粒子装饰的电纺聚合物纳米纤维用于化学传感
- Au 纳米颗粒对 HT29 和 SPEV 细胞系影响的体外研究
- 由金属纳米粒子组成的高效太阳能吸收器的数值研究
- 用银纳米粒子作为抗菌剂装饰的基于氧化石墨烯的纳米复合材料
- 通过腹膜内和静脉内给药途径对大鼠生物合成铜和氧化锌纳米颗粒的体内比较检查
- 金属和金属氧化物纳米粒子的绿色合成及其对单细胞藻类莱茵衣藻的影响
- 氧化锌纳米粒子的特性及其对微生物的活性
- 聚(γ-谷氨酸)促进 Fe-Pd 纳米颗粒对对氯苯酚的增强脱氯
- 微小的稀土氟化物纳米粒子通过电极性相互作用激活肿瘤细胞生长
- 通过调节溶剂组成选择性合成具有亚稳相的 CIGS 纳米粒子
- 深入了解纳米颗粒的细胞摄取和细胞内运输
- 用 MnFe2O4 纳米颗粒装饰的还原氧化石墨烯对四环素的吸附