发光二氧化硅纳米粒子的分类、合成和应用:综述
摘要
发光材料因其独特的光学特性而受到全世界的关注。二氧化硅对光是透明的,是发光材料的理想基质。发光二氧化硅纳米粒子 (LSN) 由于其增强的化学和热稳定性而具有广泛的应用。可以通过不同的方法合成各种尺寸的二氧化硅球以满足特定的要求。不同的发光染料具有用于不同应用的潜力。受淬灭剂等诸多因素影响,其性能表现不尽如人意。因此,这篇综述讨论了 LSN 的发展,包括它们的分类、合成和应用。重点是二氧化硅如何改善发光染料的性能以及二氧化硅在系统中的作用。此外,还描述了它们在生物学、显示和传感器中的应用。
介绍
发光材料因其特殊的光学性能而被广泛应用[1]。然而,它们的应用受到许多限制,如疏水性和生物相容性低,或由于毒性大、生物相容性差、吸光度低等缺点[2,3,4,5]。因此,有必要对发光材料进行改性以满足实际应用的需要。
具有改进特性的 LSN 在生物学 [6, 7]、照明 [8] 和传感器 [9] 中越来越受到关注。它们特有的光学特性使它们在光学材料中独一无二 [10]。二氧化硅对光是透明的,这使二氧化硅成为荧光材料的理想基质。热力学和化学稳定性也是重要因素,因为基质和二氧化硅与这些基本因素相吻合 [11]。此外,二氧化硅的表面可以很容易地进行改性,从而可以用各种官能团进一步官能化以适应不同的要求 [12]。具有上述许多优点的二氧化硅自然是改善发光材料性能的理想基材[13]。多功能纳米系统可以通过使用不同的工艺在二氧化硅纳米粒子内部和表面组装、封装或集成一种或多种不同的纳米材料来创建 [11]。作为改性发光材料,LSNs以其优异的性能在前沿研究中越来越受到关注[14]。蒙塔尔蒂等人。用有机染料掺杂的二氧化硅总结了许多在医学成像方面的优秀研究[6]。二氧化硅为荧光粉提供了一个稳定的多功能平台,但长期毒性需要研究。 Michael Schäfrling 展示了发光传感器的艺术 [9]。选择性和灵敏度是传感器材料的核心。邹华等。详细阐述了有机二氧化硅改性的方法。纳米复合材料具有分离组分的优越性能 [15]。有很多关于生物学等特定领域的精彩评论 [6, 7, 16],但缺乏对 LSN 及其在其他领域的出色表现的系统介绍。
这篇综述从 LSN 的分类开始,然后是它们的合成方法。 LSN的类别是根据发光材料的分类系统建立的。在化学性质和发光机制方面,有机分子染料、发光金属和量子点(QDs)掺杂的荧光粉是三种典型的荧光粉,作为LSNs的代表,它们都有自己独特的发光机制和优势[17,18,19 ]。重点是二氧化硅如何增强磷光体的特性。由于发光材料应用的不足,讨论了可能的策略来提高 LSN 的性能。它不仅涉及生物应用,还涉及显示器和传感器。
LSN 的分类
发出各种亮度的发光在材料领域具有重要价值[20]。围绕如何提高信噪比、稳定性和环境适应性以实现潜在应用,已经对发光材料的改性进行了大量研究。在镧系元素配合物中引入天线配体以增强发光性能是改性的典型例子。二氧化硅是混合具有不同功能和不同化学性质的材料的良好基质。已将磷光体掺入二氧化硅基质中以修饰其天然缺陷并改善其性能,这有利于具有容易改性且无毒的二氧化硅表面以及对发光染料的保护的广泛应用。由于具有多功能性和可调适应性,LSNs 越来越受到关注。在所有发光荧光粉中,有机发光分子、发光金属掺杂荧光粉和量子点是最值得强调的三大类。因此,以上三种染料与二氧化硅结合显示为典型的 LSN。表 1 中列出了代表性示例。
图>有机发光分子掺杂 LSN
有机发光分子是重要的发光材料,具有π共轭环结构和小尺寸[16]。然而,非特异性标记和漂白阻碍了它们的应用。有机染料掺杂的二氧化硅纳米粒子被广泛研究,具有优异的稳定性、选择性和生物相容性 [52, 53]。范布拉德伦等。 [21] 初步尝试合成发光二氧化硅球体。在APS((3-氨基丙基)三乙氧基硅烷)的帮助下,将异硫氰酸荧光素(FITC)涂覆在二氧化硅表面,这提供了一种通过共价键将染料与二氧化硅结合的可行方法。受这个过程的启发,安德鲁等人。 [22] 合成了两层双发射荧光二氧化硅纳米粒子。两种染料,异硫氰酸四甲基罗丹明 (TRITC) 和 FITC,在无水氮环境中通过 APS 与二氧化硅共轭。示意图和 SEM 图像(图 1a)显示了纳米颗粒的纳米结构。首先合成具有 TRITC 的二氧化硅作为双发射纳米粒子的核心,然后将 FITC 与进一步的四乙氧基硅烷 (TEOS) 共轭在核心表面。合成的双发射荧光二氧化硅纳米粒子成功地研究了大鼠嗜碱性白血病肥大细胞(RBL-2H3)的细胞内pH值,如图1b-d所示。
<图片>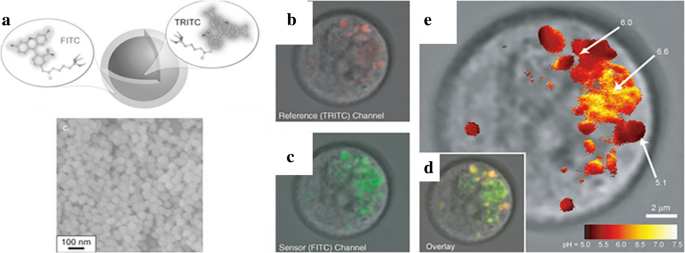
一 双发射荧光二氧化硅纳米粒子与参比染料(TRITC)和传感染料(FITC)的形成图和扫描电子显微镜(SEM)图像; RBL 肥大细胞的共聚焦荧光显微镜图像(红色为 TRITC 二氧化硅颗粒,绿色为 AlexaFluor 488-霍乱毒素 B); RBL 肥大细胞作为 pH 传感器的共聚焦荧光显微镜图像。 b 对于参考频道,c 对于传感器通道,d 对于叠加图像,和 e 根据实验计算出的pH值的假色比率成像[22]
图>为了提高有机荧光团的光稳定性,硅胶封装是一种常用的改性方法。龙焦等。 [23] 选择四种氨基花青染料作为近红外 (NIR) 荧光探针,并将它们与 3-氨基丙基三乙氧基硅烷 (APTES)(一种常见的硅烷偶联剂)相应地偶联。 TEOS 在微乳液系统中被处理过的 NIR 染料水解。离心和洗涤后获得载有花青的荧光二氧化硅纳米粒子(FSNPs)。整个过程如图 2a 所示。从图 2b 中可以看出,封装在二氧化硅中的四种 FSNP 显示出比游离染料更好的荧光强度 pH 稳定性。四个 FSNP 同时提高了它们的亮度(图 2c)。他们通过共聚焦激光扫描显微镜 (CLSM) 测试了它们在活细胞中的光稳定性。与游离染料相比,FSNP-3 和 FSNP-4(更多锚定位点)的光稳定性得到改善,而 FSNP-1 和 FSNP-2 没有得到任何改善。更多的锚定位点加强了染料分子的结构。具有刚性结构的染料具有较少的非辐射衰减和强的分子内旋转,这使得染料更亮。二氧化硅层可以保护具有增强分子结构的包封材料,并在不光漂白的情况下提高其亮度。根据图 2d 中的甲基四唑 (MTT) 方法,FSNP-3 和 4 也具有低生物毒性。生物相容性是二氧化硅的另一个优势。
<图片>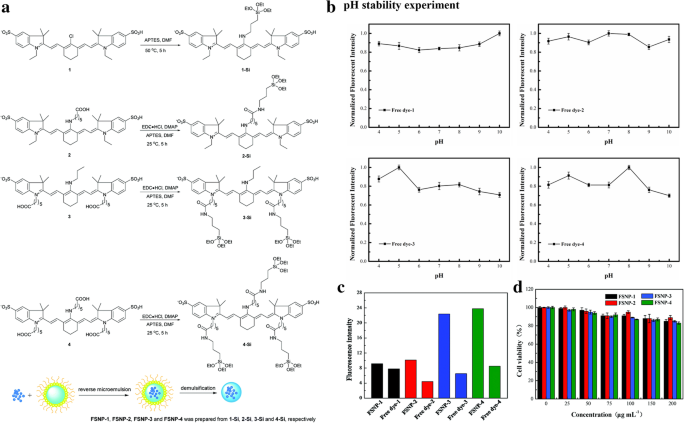
一 FSNP-1、FSNP-2、FSNP-3 和 FSNP-4 的机制。 b 所有样品在不同 pH 值下的归一化强度。 c FSNPs 和游离染料的发射强度。 d 显示与 FSNPs 孵育 24 小时后,raw264.7 巨噬细胞的活力 [23]
图>团聚是大多数发光染料猝灭的主要原因之一。荧光粉与二氧化硅可以稳定地保持在适当的浓度。与传统的发光体不同,聚集诱导发光发光体 (AIEgens) 不会遇到这个问题。相反,聚集会导致强烈的发射 [54]。为了提高 AIEgens 在生物领域的性能,许多聚合物基质被用于封装 AIEgens。此外,还有一些其他问题会导致 AIEgens 淬火,例如水和氧气,这对应用产生负面影响。二氧化硅可以防止它们与猝灭剂接触 [55]。基于上述这些分析,TPETPAFN(一种典型的由两个四苯基乙烯悬垂体和一个分子内电荷转移核心组成的荧光基团),一种 AIEgen,被 F127(聚(乙二醇)-嵌段-聚(丙二醇)-嵌段-聚(乙二醇)) 形成核心胶束 [24]。 TEOS通过改进的溶胶-凝胶法水解得到包覆在核胶束上的二氧化硅壳。如图 3 所示,合成的 TPETPAFN-F127-SiO2 纳米颗粒得益于二氧化硅壳的保护,表现出更好的光致发光性能。
<图片>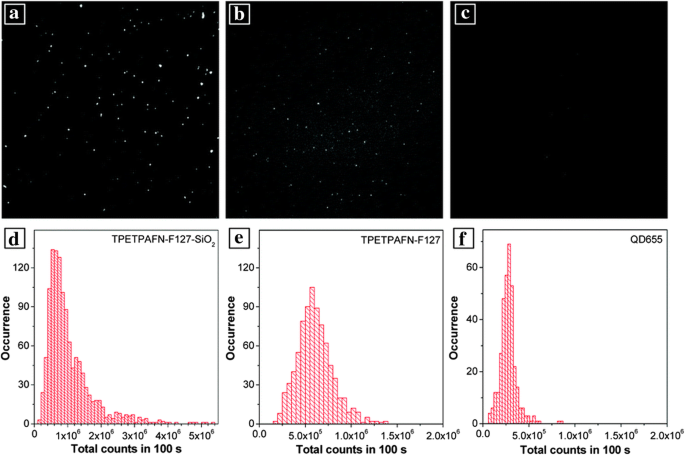
一 , d 显示 TPETPAFN-F127-SiO2 NPs 的荧光图像和光子直方图,对应 b , e 对于 TPETOAFN NP 和 c , f 用于商用 QD655 [24]
图>发光金属掺杂 LSN
稀土金属[56]和过渡金属[57]是基于电荷转移跃迁的常见发光金属材料。与配体络合后的发光是这种材料最明显的特征。金属发光有两种主要机制,LMCT(配体到金属电荷转移)和MLCT(金属到配体电荷转移)。镧系金属和过渡金属分别是它们的典型例子。由于电子能级丰富,存在多种发光金属,在不同发射发光领域具有巨大的应用潜力[58]。具有 LSPR 的贵金属已广泛用于增强发光材料,并在本节中涉及。然而,低敏化效率和猝灭限制了发光金属的应用[59]。为了提高它们的光稳定性和生物相容性,Francis 等人。将取代的甲硅烷基添加到配体中以进行进一步修饰 [30]。 Eu@Si-OH纳米粒子是通过反相微乳液法用二氧化硅包覆甲硅烷基修饰的Eu络合物后得到的。产物最终用 APTES 将胺功能化为 Eu@Si-NH2 纳米粒子。二氧化硅层使配合物远离猝灭剂(OH 和 NH2 基团)。因此,它们在图 4 中都表现出更好的光稳定性。Eu@Si-NH2 纳米粒子在生物成像方面表现出良好的性能。
<图片>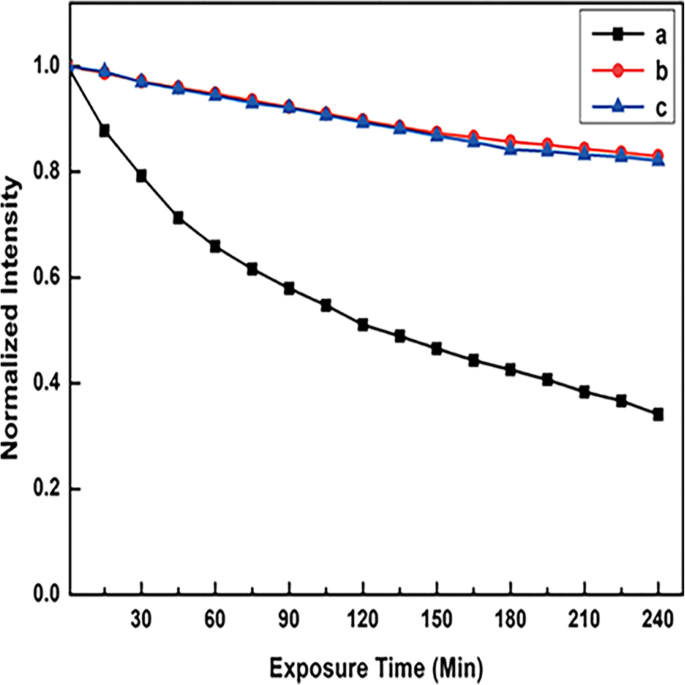
365 nm照射下荧光强度随曝光时间变化曲线,a CHCl3 溶液中的母 Eu 络合物,b Eu@Si-NH2 和 c Eu@Si-OH 纳米粒子在磷酸盐缓冲盐水 (PBS) 缓冲溶液中 [30]
图>埃斯奎罗等人。通过溶胶-凝胶工艺将 Ir 配合物、MLCT 发光配合物纳入二氧化硅骨架,以提高稳定性和光物理性能 [60]。在二氧化硅的保护下,这些荧光粉不仅在环境条件下表现出优异的稳定性,而且在恶劣的环境下也表现出优异的稳定性,进一步应用于白光发光二极管(WLED)。
Y. Li 等人[31] 合成了油酸稳定的上转换纳米粒子(UCNPs)。然后他们通过微乳液法在 UCNPs 上包覆二氧化硅层,得到水溶性 UCNPs。将 Eu (TTA)3phen 配合物引入系统,他们合成了 NaGdF4:Yb,Er@SiO2@Eu (TTA)3Phen (UCNPs@SiO2@EuTP) 纳米球。二氧化硅包覆后表面猝灭得到抑制,发射强度增强,如图5所示。在二氧化硅的帮助下,获得了两种不同发射的水溶性纳米粒子。
<图片>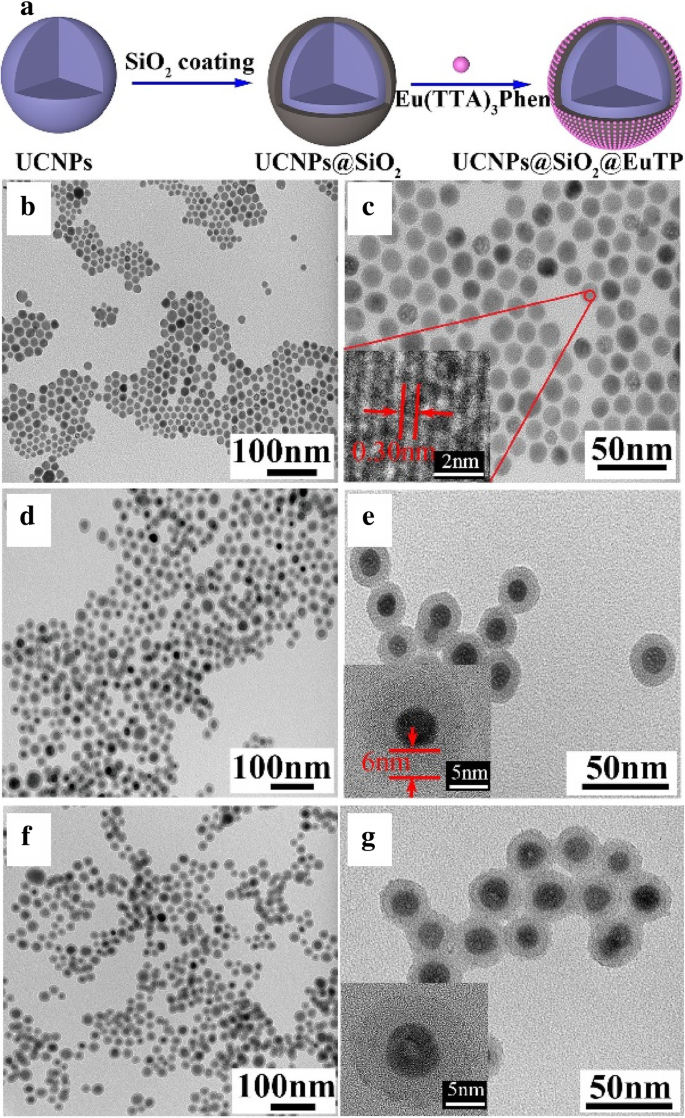
一 UCNPs@SiO2@EuTP的形成和样品的TEM(透射电子显微镜)图像; b , c 对于 UCNP,d , e 对于 UCNPs@SiO2 和 f , g UCNPs@SiO2@EuTP [31]
图>陈等人。 [42] 成功地将碳点 (CD) 和稀土离子用于 WLED。他们通过一锅有机热解法合成了 CD,其在 470 nm 处具有最大的蓝色发射,并分别在 251 和 364 nm 处具有两个激发峰。为了获得白色发光复合材料,CDs用作蓝色发射核心和Sr2Si5N8:Eu 2+ 磷光体用作橙色发射组分。 CD 被放入 Stöber 系统。当 TEOS 水解时,CD 会被一层带有红色磷光体的二氧化硅层覆盖。在离心、干燥和研磨后合成碳点-二氧化硅-磷光体复合材料(CDSP)。 CDSP 具有从 UV(紫外线)到黄色区域(200-600 nm)的广泛吸收,在 UV 区域特别强。在测试了不同波长的激发后,他们发现 CDSP 在图 4 中的 400 nm 激发下获得了最接近的国际照明委员会 (CIE) 坐标 (0.32, 0.32) 与纯白光 (0.33, 0.33) 的坐标。 6. 通过调整CD与荧光粉的质量比,获得CDSP的发射率是一个很好的尝试。在 400 nm 激发下,它们获得了与白色发射最接近的质量比(3.9% (0.32, 0.32) 和 5.1% (0.34, 0.32))。 CDSP 在发光二极管 (LED) 封装中显示出比 CD&P(直接与 CD 和磷光体混合)(0.28、0.29)更好的白光发射(0.30、0.31)。两种组分与二氧化硅均匀分散,降低了聚集和相分离的可能性。最后,他们在 UV 二极管芯片(375 nm)上得到了一个带有 CDSP 粉末的 WLED,并获得了白光(0.30、0.33)。显色指数(CRI)约为94,高于基于YAG:Ce的商用WLED(CRI <75)。
<图片>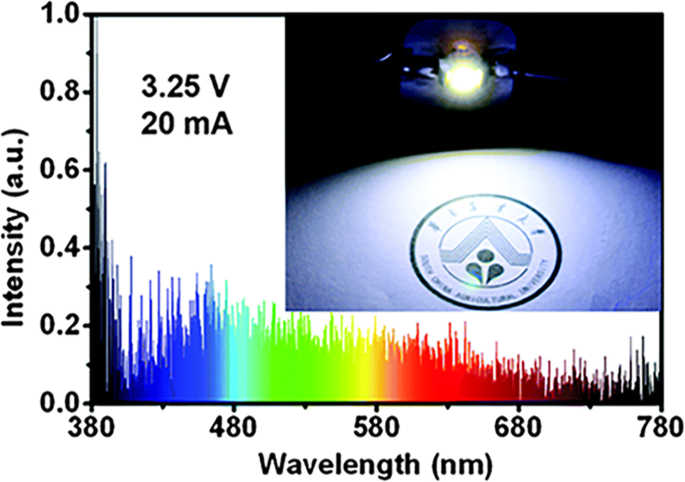
基于CDSP的WLED性能:发射光谱和照片[42]
图>二氧化硅通常用作发光材料的保护层,以与贵金属保持适当的距离,以增强荧光。这是由于光引起的自由电子的静止振荡。为了增强发光,染料与贵金属颗粒之间需要保持适当的距离。至于贵重增强材料,金属纳米粒子本身会导致发色团猝灭(在 5 nm 内),但它们的荧光可以增强 100 倍(在 10 nm 左右)。在早期的研究中,Tuo Li 等人。 [61] 在微乳液基质中合成了具有二氧化硅壳的银纳米颗粒(Ag/SiO2 纳米颗粒)。在银还原后,将生产二氧化硅所需的试剂(TEOS 和环己烷)注入微乳液中。他们仔细研究了不同条件(R 为水/表面活性剂,H 为水/TEOS)对 Ag/SiO2 纳米粒子的影响,结果如图 7 所示。这是在表面涂覆均匀厚实的二氧化硅层的好方法核心不仅是银,还有其他具有微乳液系统的纳米粒子。什么振华白等。 [25] did 就是一个很好的例子。 8-Hydroxypyrene-1, 3, 6-tresulfonic acid (HPTS) 是一种荧光 pH 敏感染料,由于其独特的优势,适合用作细胞内 pH 传感器。但极端的 pH 值条件使其不敏感。当溶液呈酸性时,其荧光效率会显着降低。基于贵金属增强的荧光效应制备了 HPTS 吸附的 Ag@SiO2 纳米粒子(图 8a)。从图8b可以看出,Ag@SiO2-8 nm@HPTS表现出更好的荧光强度,尤其是在极端pH条件下。
<图片>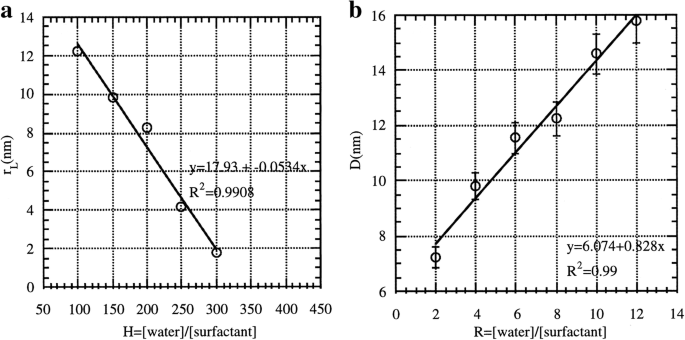
一 作为 H (R =4 和 X =1); b 当 R 是可变的,Ag 簇的大小变化 [61]
图> <图片>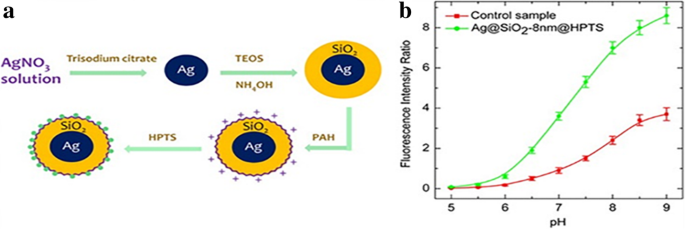
一 HPTS 吸附 Ag@SiO2 纳米粒子的合成进展[J]. b Ag@SiO2-8 nm@HPTS(绿色)与对照样品(红色)的荧光强度比[25]
图>QDs-Doped LSNs
由于量子限制效应,无论是半导体量子点、碳量子点还是其他类型的量子点,量子点都表现出优异的发光特性。最近,许多研究都集中在量子点在光学器件中的应用上。有时,它们的性能不足以适应复杂的应用。必要的改性势在必行,二氧化硅是合适的基质[1]。
为了实现生物标记和磁共振成像的结合,CdSe QDs 被带有 NH2 基团的二氧化硅层包覆在磁性 Fe2O3 核上。相关图片和表征如图 9 所示。结合 NH2 基团与生物锚定膜 (BAM),4 T1 小鼠乳腺癌细胞膜显示出 BAM-SiO2-CdSe MQD 的特异性标记 [44]。具有生物相容性和磁性的多功能发光纳米粒子将在医学上获得广泛的应用。
<图片>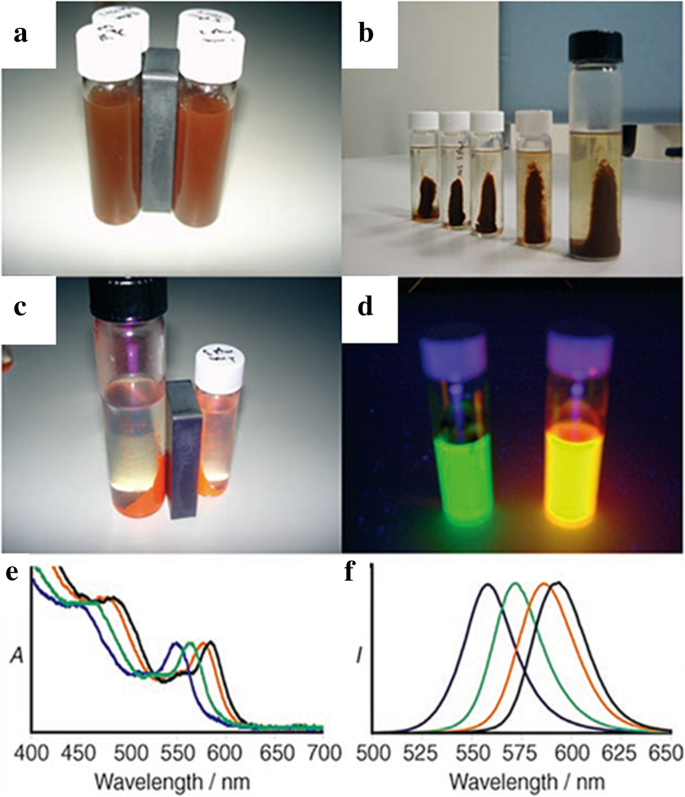
在常光下证明磁性改性的照片(a , b )。 c 紫外光下的照片证明了磁性和发光特性。 d 绿色和橙色磁性量子点 (MQD) 的 UV 照片。 e , f SiO2-MQDs 在 PBS 溶液中的发光光谱 (e 用于吸收和f 发射) [44]
图>为了扩大量子点的应用,有必要改变它们的水溶性和无毒性。二氧化硅在量子点的修饰中显示出巨大的潜力。马云飞等。 [43] 将自制的相转移试剂(腺苷 5'-单磷酸腺苷,AMP)和硅烷偶联剂(3-巯基丙基三甲氧基硅烷,MPS)引入 Stöber 系统。油溶性(初始 CdSe/CdS/ZnS QDs)、醇溶性(AMP-QDs)和水溶性(QDs 周围 TEOS 的水解)是溶解度变化的整个过程。 QD@SiO2 具有与最初相同的光致发光效率(50-65%)。更宽的pH范围(pH 4-8至2-13)、提高电解质稳定性、更好的热稳定性以及提高Hela细胞的生物相容性是QD@SiO2的优势。
为了保证光器件中量子点的稳定性,需要减少闪烁的影响。闪烁是一种随机间歇发光的现象,会影响 QD 光学器件的稳定性 [62]。为了减少眨眼的影响,Botao Ji 等人。 [45] 以 CdSe/CdS 量子点为核心材料,并通过反微乳液法置换初始疏水配体,将这些量子点封装到二氧化硅壳中。量子点进一步被二氧化硅表面的金层改性,聚(1-乙烯基咪唑-共-乙烯基三甲氧基硅烷)(PVIS)作为硅烷偶联剂。纳米尺寸的金壳充当等离子体共振器,使 QD 增强了光学状态密度。由于混合层,无论局部环境发生剧烈变化,都可以保留 QD 的属性。结果,量子点的光稳定性增加。镀金后,QD 荧光寿命从 123 ns 减少到 20 ns。金色量子点表现出高效的多激子发射,其中性光致发光强度高于量子点。稳定性测试结果如图 10 所示。此外,光致发光强度可以保持稳定数小时(甚至 24 小时)。发光稳定性测试表明,仅 1 小时后,裸量子点的发光就会急剧下降。二氧化硅层略微改善了量子点的性能,但为下一层金层提供了合适的间隔,以显示等离子体增强效果。
<图片>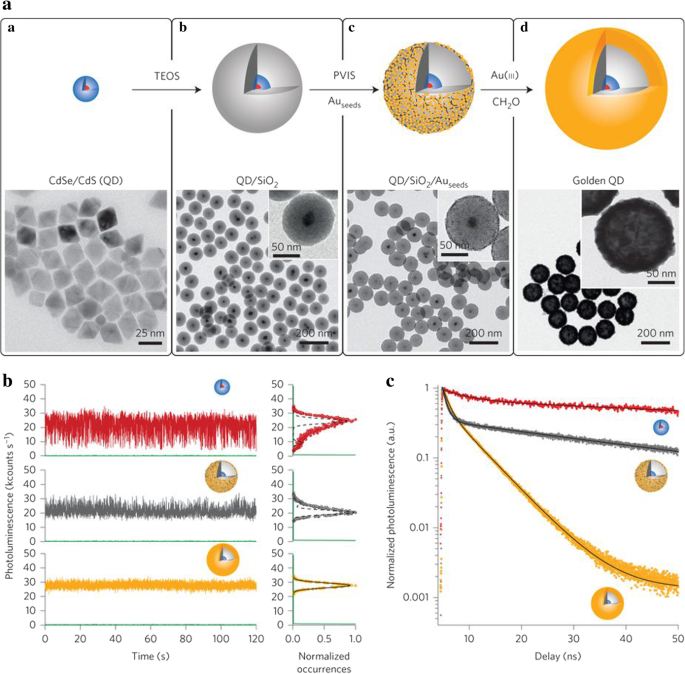
一 量子点/SiO2/Au 杂化(金 QD)示意图和每个阶段的 TEM 图像(CdSe/CdS QD、QD/SiO2 QD/SiO2/Auseed 和金 QD)。 b 光致发光强度随时间的变化。 CdSe/CdS 为红色,QD/SiO2/Auseed 为灰色,金色 QD 为橙色。 c (b中三种纳米粒子的光致发光衰减曲线 ) [45]
图>LSN的合成方法
对于LSNs的制备,荧光粉的选择和合成路线的设计是核心内容。磷光体决定了 LSN 的发射范围,合成路线确定了它们的结构和功能。 LSNs的所有合成路线都基于二氧化硅。溶胶-凝胶法、反微乳液法和直接胶束辅助法是获得均质规则二氧化硅球体的三种主要方法,已用于 LSNs。图11是上述方法的示意图。
<图片>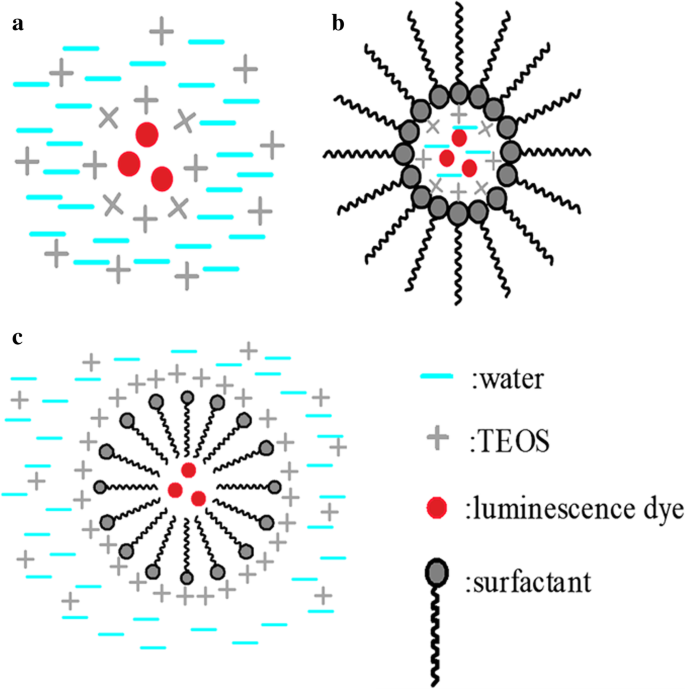
使用不同方法的不同 LSN 的示意图。 一 对于 Stöber 方法。 b 对于反相微乳法,c 直接胶束辅助法
图>溶胶-凝胶法
溶胶-凝胶法,也称为 Stöber 法,是一种方便可行的获得单分散二氧化硅纳米球的方法。合成二氧化硅纳米球是理想的方法,因为 Stöber [63] 仔细研究了在氨催化下用烷氧基硅烷水解合成 50 nm-2 μm 范围内特定尺寸的二氧化硅球。通过溶胶-凝胶法控制乙醇与水的比例、氨的量和温度等合成条件,可以很容易地获得不同尺寸(10至数百纳米)的均质二氧化硅球体。使用 Stöber 方法,Van Blaaderen 和 A. Vrij Langmuir 通过在反应体系中添加 (APS) 成功合成了染料 (FITC) 掺杂的二氧化硅 [21]。使用来自 APS 的胺基团,二氧化硅球很容易捕获 FITC,如图 11a 所示。到目前为止,除染料外,许多其他材料已通过 Stöber 方法与二氧化硅相连。 Luis M. Liz-Marzan 等。改进了 Stöber 方法并使用(3-氨基丙基)-三甲氧基硅烷(APTS)作为表面活性剂合成了金-二氧化硅核-壳颗粒[64]。结合金核,APTES 为二氧化硅封装提供化学键桥接。碱性条件导致均匀的二氧化硅球体作为流行的 Stöber 系统,烷氧基硅烷的酸催化水解也是将发光染料封装到二氧化硅中的可行方法[65]。
基于 Stöber 方法合成了一种新型 LSN。杨林刚等。 [50]基于乙烯基的π-π堆积,通过Stöber方法成功合成了结晶二氧化硅。以乙烯基三乙氧基硅烷 (VTES) 为前驱体的 Stöber 进展,用盐酸中和,真空蒸馏除去溶剂,然后用四氢呋喃萃取是有机二氧化硅纳米晶体 (OSNC) 的整个过程。已经合成了三个具有相同晶体结构但尺寸不同的 OSNC,如图 12d-f 所示。由于VTES的增加,有机二氧化硅纳米晶体(OSNCs)的尺寸逐渐增加。结果,它们显示出不同的发光特性,如图 12g、h(紫外光下的蓝色、绿色和红色)所示。 OSNCs 具有良好的光稳定性和 pH 稳定性。由于 π-π 堆积,金刚石立方晶体结构中的乙烯基外延生长。有序堆叠的乙烯基最终形成一个大的π共轭体系,在量子限制后具有荧光。由于二氧化硅的特性,这些OSNCs在光学领域具有巨大的潜力,为获得自发光二氧化硅材料提供了新的途径。
<图片>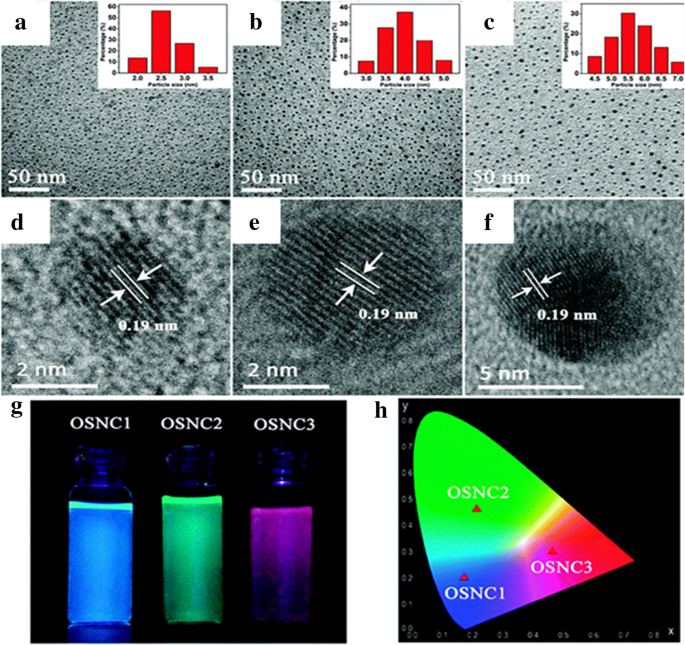
OSNC 的特征:a –c 作为 TEM 图像和 d –f 作为高分辨率透射电子显微镜(HRTEM)图像。 g 受紫外光照射的 OSNC 样品的照片。 h 国际照明委员会 (CIE) 色度图上的分布 [50]
图>反相微乳法
Stöber法是一种简单方便的合成LSNs的方法,但不受控制的反应条件和初始粒子对发光染料的限制。为了克服这些限制,Bagwe 和 Khilar [66] 在合成涂有二氧化硅纳米复合材料的银的过程中引入了油包水微乳液系统 [67](图 11b)。使用表面活性剂将含 TEOS 的银纳米粒子的初始碱性水溶液封装在水滴中。 TEOS的水解过程与Stöber法相同。但整个进展仅限于由表面活性剂包裹的水滴,这导致了控制良好的系统和单分散的二氧化硅纳米粒子。通过选择不同的表面活性剂、溶剂和改变表面活性剂与水的比例,可以很好地控制二氧化硅的尺寸。当荧光团是水溶性的时,很容易在液滴中分子的表面形成均匀的二氧化硅层。王念芳等。 [46] 通过反相微乳液法合成发光二氧化硅包覆的 CdS/CdSe/CdS 纳米粒子。图 13 显示了合成 QD 和 QDs@SiO2 的 TEM 图像。受保护的量子点显示出优异的酸和热稳定性。为满足应用的特殊要求提供了进一步修改的可能性。
<图片>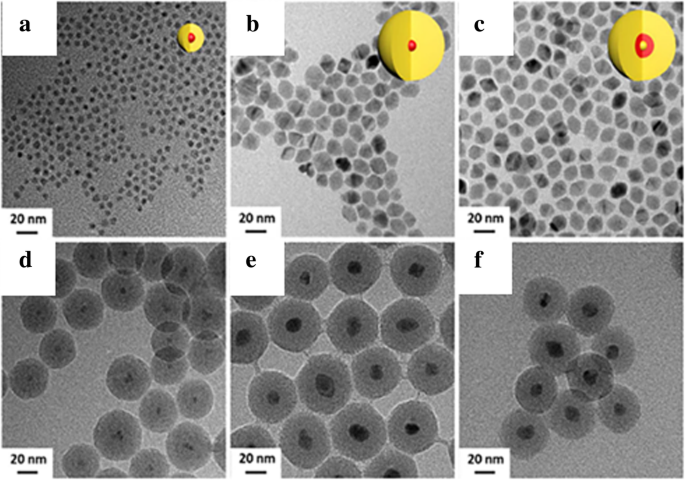
具有 CdS 薄壳 (a) 的 CdSe/CdS 核/壳量子点的 TEM 图像 ) 和用二氧化硅 CdSe/CdS@SiO2 (d ); CdSe/CdS 核/壳量子点与 CdS 壳 (b ) 和用二氧化硅 CdSe/CdS@SiO2 (e ); CdS/CdSe/CdS 核/壳量子点 (c ) 和用二氧化硅 CdS/CdSe/CdS@SiO2 (f ) [46]
图>直接胶束辅助方法
反相微乳液法需要水溶性发光染料。相反,脂溶性初始胶束是直接胶束法的主要特征,水解过程发生在胶束周围(图 11c)。 A precursor is indispensable for the agglomeration of silica. As a common progress, the luminescent dye is modified with the silane coupling agent, such as APS, to form the assistant micelles. The initial modified micelles ensure that the TEOS condensation occurs around them. Using Rhodamine B conjugated to APTES as the original micelle, Kumar et al. [26] successfully synthesized Rhodamine-conjugated organically modified silica nanoparticles in oil in water system and modified them with different function groups (such as sulfhydryl, amino, and carbonyl) which can be used as cell fluorescence probe.
The role of the surfactant is not only reflected in the silica synthesis but also in the synthesis of mesoporous silica. A common method of synthesizing mesoporous silica is calcination. Large specific surface area and modifiable surfaces make the mesoporous silica nanoparticles perfect carriers. In addition to the known application value in the field of medical drug loading, it also has important application prospects in the field of loading phosphors. Li Wang et al. [68] mixed up CDs with hollow mesoporous silica microspheres with good photochemical stability which can be used for oxygen detection in the whole range. Mesoporous structure makes them unique. Bin Xie et al. [69] incorporated the CdSe/ZnS core-shell QDs into mesoporous silica microspheres by a swelling and evaporation method. Coated with a mesoporous silica layer on the surface of Gd2O3:Eu phosphors via modified Stöber method is also feasible according to the Ali Aldalbahi et al. [70]. Because of the encapsulation of silica, the modified Gd2O3:Eu nanoparticles showed excellent solubility and biocompatibility.
Other Methods
There are also other methods to synthesize LSNs such as chemical vapor deposition (CVD) [71], hydrothermal method [51], and amino acid-catalyzed seed regrowth technique [72, 73].
Lianzhen Cao et al. [71] synthesized SiC/SiO2 by CVD and thermal annealing processes. Si was used to coat on the SiC core by thermal CVD and then SiO2 shell was obtained after oxidizing. The annealed SiC/SiO2 nanoparticles showed narrow luminescence in the blue-green region. The synthetic method provided a new way to synthesize core-shell nanomaterials.
Chandra et al. [51] synthesized smaller fluorescent silica nanoparticles (1 to 2 nm) with silicon tetrabromide (SiBr4) and APTS. Heating to 200 °C in an autoclave was the core step of the whole reaction. The final products were obtained after further purification including dialysis and centrifugation. The silica nanoparticles emitted bright blue luminescence with a photoluminescence quantum yield around 34%. It was non-photobleaching and biocompatible at the same time.
Surface modification makes the LSNs more tunable for complex application [74]. Silane coupling agents are the most common chemical methods as it mentions before. Abundant hydroxyl groups provide reaction sites for further modifications. Junqiang Wang et al. synthesized silica modified CeO2 ammonia sensor with high gas response due to hydroxyl groups [75]. After hydrolysis and condensation, silane coupling agents with different function groups bond on the surface of silica. Superhydrophobic silica was synthesized with the condensation of VTES (-CH=CH2) [76]. Ming Ma et al. grafted PEGMA and DMEAA on the surface by RAFT polymerization based on the -NH2 of APTS [77]. Surface modification can enhance their adaptability in complex environments and get improved luminescence properties with appropriate materials.
Among these methods, there are two main ideas to fabricate LSNs, namely the luminescent dyes are added directly into the reaction system when the silica resources start hydrolyzing, and that the luminescent dyes are established chemical bond with silica by other reagents such as silane coupling agents, either before or after silica network set up. It is necessary to select and design an appropriate synthetic route for LSNs with specific structures.
Applications of Luminescent Silica Nanoparticles
Light is the most intuitive tool for people to recognize the world. Luminescent materials with special emission can be directly used in many ways such as lighting, display, and so on. At the same time, changes in fluorescence intensity can reflect some important information. Compared with separate luminescent dyes, LSNs have improved performances in applications, since silica provides a stable matrix for the luminescent dye. It provides an effective way for multifunction at the same time [6]. LSNs with multifunction and tunable surface have great application prospects and development potential in biology, lighting, and sensors.
Biolabeling and Medicine
LSNs have great application value in biology. Non-toxicity is a fundamental requirement for medical field, especially in vivo [78]. The fact that the common luminescent dyes are often toxic limits their clinical application [79]. Silica, a favorite non-toxic modified material, is a good solution to elimination of toxicity. Toxicity of silica nanoparticles (20–200 nm) were also carefully studied by In-Yong Kim et al. [80]. Size, dose, and cell type-dependent cytotoxicity were the issues in their research. Although high dose can cause a disproportionate decrease in cell viability, the silica nanospheres with 60 nm showed their good biocompatibility up to 10 μg/ml. Different cells had different tolerance to silica nanoparticles which indicated that it was necessary to have substantial tests before clinical tests. Although inhalation of silica particles can cause acute and chronic diseases including silicosis [81], silica still has potential in biological application at the nanoscale. The toxicity of luminescent silica nanoparticles to living cells was studied in detail by Yuhui Jin et al. [38]。 From the DNA level to the cell level, the toxicity of RuBpy-doped LSNs were carefully tested. At a certain concentration, the results showed that the dye-doped luminescent silica nanoparticles were non-toxic to the targeted DNA and cells, which indicate that LSNs are a good solution to the non-toxic modification. Xiqi Zhang et al. [27] encapsulated AIE dye (An18, derivatized from 9, 10-distyrylanthracene with an alkoxyl endgroup) into the silica nanoparticles via a one-pot modified Stöber method. Coated with silica lead to an enhanced fluorescence intensity, good water solubility, and non-toxicity to living cells which made the An18-SiO2 NPs had a potential for biomedical application.
LSNs have great application value in diagnosis and biolabeling. For hybrid imaging contrast agents, Dong Kee Yi et al. [48] combined magnetic particles (MPs) Fe2O3 with QDs (CdSe) and encapsulated them in silica shell by reverse microemulsion method. The nanostructures of MPs with QDs are clearly showed in Fig. 14. Magnetic resonance imaging (MRI) is an effective method for disease detection, especially for cancer. The advantages of feasible usage, low cost, and accurate diagnosis make it more popular as a diagnostic tool [7]. The nanocomposites can be used as both optical and MRI contrast agents. It is worth mentioning that the presence of CdSe increased the effective magnetic anisotropy of the γ-Fe2O3-containg particles. This is a good attempt, but the low quantum yield (SiO2/MP-QD 1.1% to CdSe 11.4%) limits the actual effect. Willam J. Rieter et al. [39] also synthesized the same multifunctional nanocomposites. What is different is that [Ru (bpy)3] Cl2 was chosen as the luminescent core and the paramagnetic Gd complex was coated on the luminescent core by water-in-oil reverse microemulsion method. The nanocomposites were finally embedded in silica in the same way. The results of Fig. 15 proved that hybrid silica nanoparticles had good optical and MRI performances in biological imaging. Mesoporous silica nanospheres doped with europium (Eu-MSN) were obtained by Mengchao Shi et al. [32]。 Nanoscale size (280–300 nm) and fluorescent property were the basic for an ideal biolabeling material. They found that Eu-MSNs had a positive influence on osteogenesis and angiogenesis-induction. By promoting proper response of macrophages and the expression of relevant genes, the defect of bone replaced by new bone and the healing process of skin wound can accelerate with Eu-MSNs. Besides the function of biolabeling, the LSNs showed the potential in tissue repair. LSNs can achieve the target binding effect by modifying the special group. In Duarte’s work [33], organosilane Bpy-Si was chosen as a ligand of Eu complex for the further reaction with silica. SiO2-[Eu (TTA)3(Bpy-Si)] nanoparticles were obtained with a uniform size (28 ± 2 nm). With a further modification of an amino acid spacer and an anchor group (anti-Escherichia coli , IgG1), the functionalized silica had the specific bonding with E . coli bacteria. It was easy to get the distribution of E . coli bacteria with luminescence. The bio-multifunction of LSNs was also carefully studied by Laranjeira et al. [82]. Gadolinium (Gd) composites with unique magnetic properties have potential in MRI contrast agents but Gd3+ ions are toxic in humans especially in kidneys and pancreas. GdOHCO3 nanoparticles were chosen as the MRI contrast core and coated with silica layer via Stöber method. With the silica coating, the Gd composite (SiGdOHCO3) had the same brightness of MRI contrast images but no degradation at designed pH values (5.5, 6.0, and 7.4). And SiGdOHCO3 had little effect on human fibroblasts according to the cell proliferation assay which indicated an excellent biocompatibility. Silica provides a more stable environment and further possible modification for GdOHCO3 without affecting MRI performance. By diverse micelles method, Atabaev et al. [83] synthesized Gd2O3:Tb 3+ ,Eu 3+ @SiO2 nanoparticles which can be used as both MRI contrast and fluorescence agents in vivo. The above two examples perfectly reflected the role of LSNs in multifunction with the silica platform.
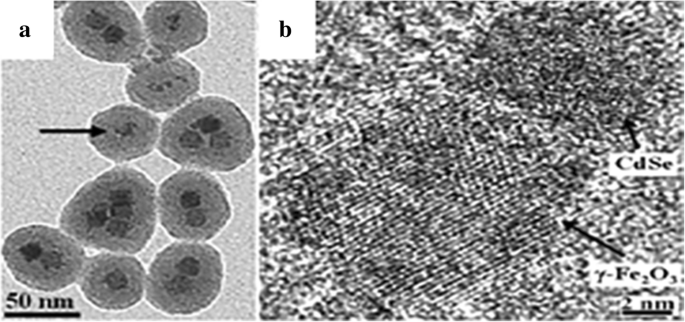
一 TEM 图像和 b HRTEM image of SiO2/MP-QD nanoparticles [48]
图>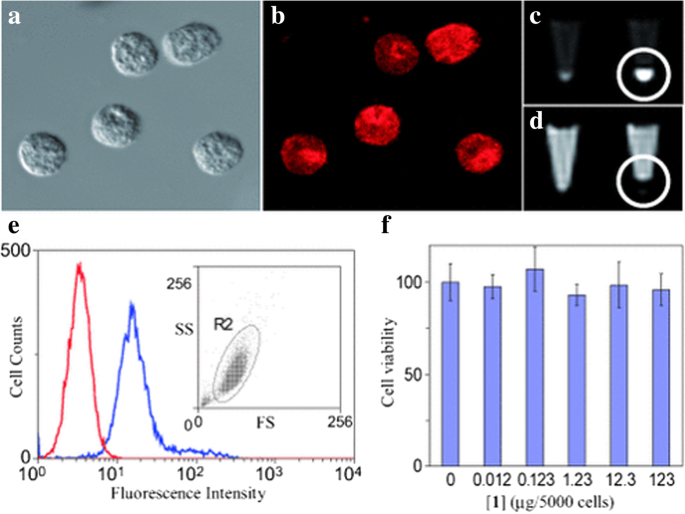
The imaging results of monocyte cells with a optical microscopic, b laser scanning confocal fluorescence, c , d the images of monocyte cells with MR:left for unlabeled monocyte cells and right for hybrid silica nanoparticles labeled monocyte cells, e flow cytometric results of blank and hybrid silica nanoparticles-labeled monocyte cells, and f the cell viability with different amount of hybrid silica nanoparticles [39]
图>LSNs have great application value in drug delivery. Hongmin Chen synthesized luminescent mesoporous silica nanoparticles biofunctionalized by targeting motifs, which makes them applicable in drug delivery [47]. They first prepared APS-containing mesoporous silica particles, and subjected the products to calcination at 400 for 2 h. They synthesized mesoporous silica by the help of cetyltrimethyl ammonium bromide (CTAB). There were luminescent carbon dots in the silica matrix after calcination. The fluorescence intensity was at the maximum when the particles were excited at 380 nm. The target selectivity of FL-SiO2 was achieved by surface modification of RGD peptide with the help of APS. They also studied the RGD-FL-SiO2 loading and release of doxorubicin (Dox). After calcination, fluorescent mesoporous silica (FL-SiO2) can still load Dox effectively. The porous structure was not affected by calcination. They found that RGD-FL-SiO2 had good luminescent effect especially around the blood vessels of tumor in vivo imaging studies. Integrin αvβ3 of the tumor was the key of the interactions. Although there are many excellent attempts to apply LSNs to medicine but less successful clinical tests in human beings means that there is still a long way to go for the real medicine applications.
Light-Emitting Devices
Due to their special emitting features, LSNs also play a vital role in light emission fields including the field emission- and liquid crystal-based display technologies [84]. WLEDs have received recent attention for their broad applications including general illumination and displays. Tunable color, high color purity, and luminescence efficiency are in line with the requirements of light-emitting diodes (LEDs) [85]. Quantum dot-based light-emitting diodes (QD-LEDs) have demonstrated recently, and may offer many advantages over conventional LED and organic light-emitting diodes (OLEDs) technologies in terms of color purity, stability, and production cost, while still achieving similar levels of efficiency. In order to improve the performances of polymer dots (Pdots) as WLED phosphors, Kaiwen Chang et al. [49] introduced some Pdots with different emission wavelength into the Stöber system to get encapsulated. The silica-encapsulated Pdots showed the same luminescence properties but markedly enhanced photostability.
To reduce the manufacturing complexity required for achieving full-color displays, it is more desirable to use a common device structure to achieve high efficiency for three primary colors (blue, green, and red). QDs have been widely used in display field because of its unique luminescent properties, such as high luminescent intensity, narrow emission spectra, and tunable emission. Chun Sun et al. [34] synthesized the perovskite QDs, CsPbBr3, as the light-emitting core of WLEDS. Only the perovskite QDs are not enough for a LED device since photostability and stability are necessary for an optical device under long-time work and elevated temperature. There are anion-exchange reactions between different halide QD nanoparticles which would widen the narrow emission spectrum. QD/silica composite were fabricated in APS to avoid oxidation and decomposition. So they used APTES as the QDs’ capping agent and improved the silica coating process to avoid the decomposition of the QDs. Green and red QD/silica composites were synthesized and a WLED was obtained by the combination of the composites with a blue LED chip. The WLED had good performances with great air stability as depicted in Fig. 16.
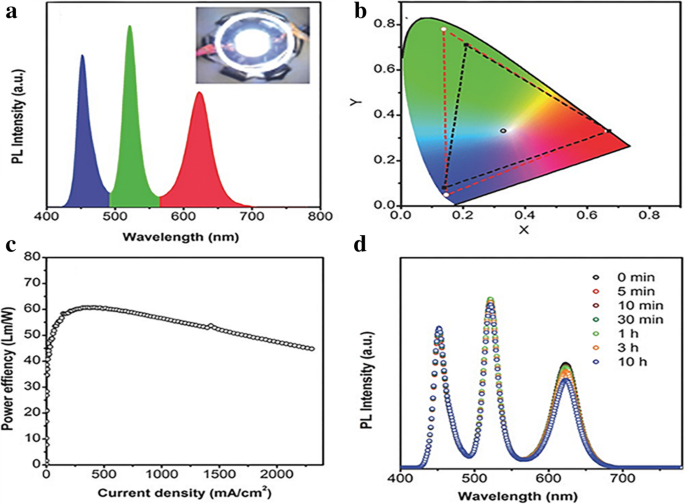
The optical performances of the WLED:a the emission spectra, b the CIE color coordinates and the color triangle of WLED (red dashed line) with the NTSC TV standard (black dashed line), c the power efficiency, and d emission spectra after working for a while [34]
图>LSNs can keep good dispersion, brightness, and photostability of QDs. Hung-Chia Wang et al. [35] provided a new composite method for QDs and silica (Fig. 17). By mixing the QDs with mesoporous silica powder of which pore size was bigger than that of QDs in non-polar solution, mesoporous silica green PQD nanocomposite was obtained after washing and drying. The quantum dot showed better thermal stability and photostability after composited with silica. On the other hand, QDs are a typical kind of aggregation-caused quenching (ACQ) nanoparticles, which means that it is necessary to keep a good dispersion to get a good brightness and photostability. Kai Jiang et al. [86] synthesized carbon dots with red, green, and blue luminescence with phenylenediamines as precursors to enhance luminescence properties as solution and poly (vinylalcohol) (PVA) film. But it would exist quenching effect as solid-state CDs which was fatal for LED devices owing to aggregation and the result Förster resonance energy transfer (FRET). To avoid the dispersion and the resulting FRET phenomenon, Junli Wang et al. [36] embedded carbon dots into silica matrix (Fig. 18) by dispersing carbon dots into the N -(3-(trimethoxysilyl)propyl) ethylenediamine (KH-792) and heating to form a homogenous CD/silica film. A white LED was fabricated by drying the CD/silica solution on the inner wall. By the assistant of silica, CDs were well dispersed with an appropriate distance without quenching which improve the performance as powders. Figure 18 showed the emission spectra and performance in WLED. And the CIE coordinates (0.44, 0.42) and correlated color temperature (CCT) (2951 K) suggested that it was suitable for indoor illumination.
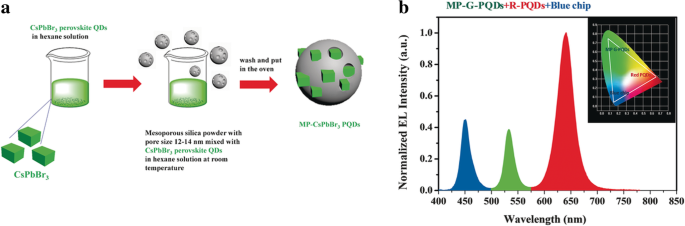
一 The formation progress of MP-CsPbBr3PQDs. b The luminescence intensity and the color triangle of WLED [35]
图>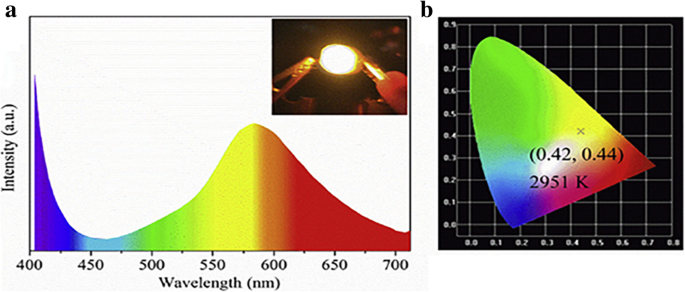
The performance of WLED showed as a the emission spectrum and b for CIE chromaticity and CCT [36]
图>Sensors
Luminescent silica showed the excellent performances on static luminescent materials, such as biolabeling and WLED phosphors. All these were based on their unique and stable optical properties. When it came to dynamic luminescent materials, LSNs also display the same wonder [9]. The luminescent sensors of pH [28], ions [87], and temperature [40] are following as representatives.
pH value have great influence on the luminescence intensity which inspires luminescent pH sensor. In the same principle as ref. [22], Atabaev et al. synthesized the same ratiometric pH sensor [28]. FITC was chosen as the pH-dependent luminescence dye and Y2O3:Eu 3+ as pH stable dye. With the Stöber coating of silica, Y2O3:Eu 3+ @SiO2 with FITC composite NPs were successfully synthesized. The change of pH was reflected by the ratio of fluorescence intensity (I FITC/I Y2O3:Eu3+). The standard dye led to a less influence of concentration and a more accurate result.
LSNs can also be used as ions sensors. Based on the changes of luminescence intensity with the measured physical quantity, LSNs have been applied to many sensor fields by the environment-dependent effect of the luminescence. Quenching effect is an effective detective tool to detect the changes of quenching factors such as ions and pH value with external quenching mechanisms such as FRET and photoinduced electron-transfer (PET) [9]. Sensors for metal ions are important fields whether in cells or open system. Won Cho et al. [37] synthesized europium (III) coordination polymer (EuCP) and found the specific quenching effect of Cu 2+ (Fig. 19). In view of this fact, they synthesized silica@EuCP microsphere which have the same sensitivity on Cu 2+ with less mass of europium. As an auxiliary material, silica can effectively reduce the amount of sensor materials. Both of them have their unique situations. Besides quenching effect, there are some different effects which can be used in the fields of sensors. 2,2-Dipicolylamine (DPA) and its derivatives have good affinity to heavy ions. And enhanced luminescence effect would happen after chelated with heavy ions. Yu Ding et al. [29] modified silica spheres with N ,N ′-bis (pyridine-2-ylmethyl)ethane-1,2-diamine (Fig. 20). The concentration of heavy ions (Cd 2+ Hg 2+ 和铅 2+ ) in samples can be determined by the change of fluorescence intensity. The test in real water samples and simulated biological samples confirmed the heavy metal ions-binding ability and the detection which has application prospects in the water monitoring and so on.
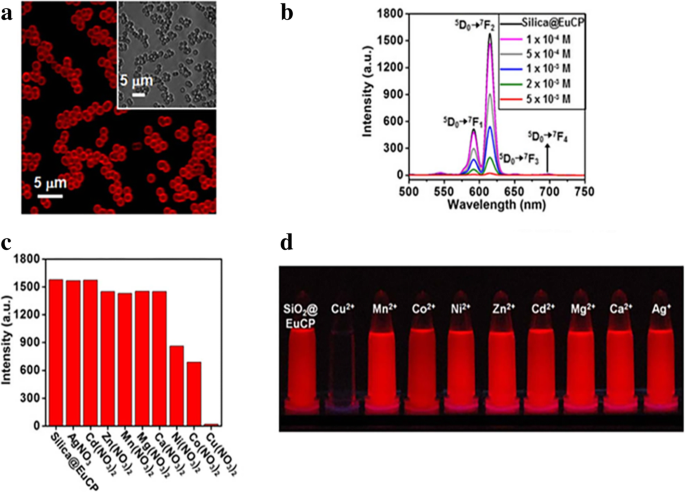
一 Confocal microscopy and OM (inset) images of silica@EuCP microspheres. b Luminescence spectra with different Cu (NO3)2 in MeCN; luminescence intensity changes (c ) and photograph (d ) with different metal ion solutions (5 mM) [37]
图>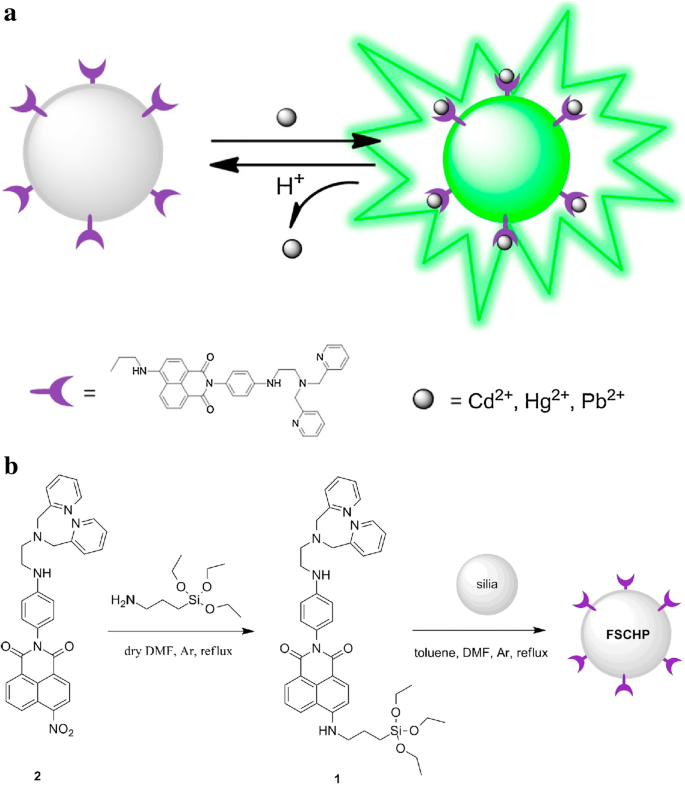
The formation and sensing progress scheme of sensitive fluorescent sensor (FSCHP) [29]
图>Temperature sensors are also important applications of LSNs. Temperature is a basic variable in most science fields. The temperature dependence of radiative and non-radiative transition rates is the core content of temperature sensing which makes it possible for luminescence temperature sensing, with the contactless and large-scale advantages [9]. However, in order to be applied in practice, their stability is crucial as the environment of application is more complex than of that of experiment condition. Silica is an ideal matrix to improve their performance for application. Mirenda et al. [40] synthesized silica as the core and then TEOS was hydrolyzed with Ru (bpy)3Cl2 to form the Ru (bpy)3@SiO2 NPs. The emission spectra of Ru (bpy)3@SiO2 NPs (Fig. 21) showed that the intensity of Ru (bpy)3@SiO2 NPs decreased linearly as the temperature rising as the result of the activated non-radiative 3 d-d state (20–60 °C, λexc = 463 nm). The polyethyleneimine (PEI)-modified glass with Ru (bpy)3@SiO2 NPs showed the same trend as the NPs which proved that the potential as the temperature sensing. With cycling the temperature between 20 and 60 °C, the relative slope decreased until the seventh cycle which meant that it is necessary to condition to obtain the stable sensing materials. The influence of temperature on probes is complicated. So it is necessary to research the temperature-dependent luminescence of the probes to know how to apply it into temperature sensors. Temperature is a fundamental variable that governs diverse intracellular chemical and physical interactions in the life cycle of biological cells. The change of temperature reflects the level of cell metabolism. GdVO4 co-doped with Er 3+ (1 mol%) and Yb 3+ (1 mol%) has the potential to apply as the temperature sensor. To improve their performance as temperature sensor, Savchuk et al. [41] coated silica shell on the nanoparticles surface by Stöber method. The fluorescence intensity ratio (FIR) of Er, Yb:GdVO4, I 520/I 550, had a certain linear relationship with temperature in the range from 297 to 343 K after excitation at 980 nm. And the probes got enhanced thermal sensitivity, high thermal resolution and good stability in different solvents. And the result of the ex vivo experiment to monitor temperature evolution with the special sensor showed in Fig. 22 proved that Er, Yb:GdVO4@SiO2 core-shell nanoparticles had a good thermal resolution as the temperature sensor in biomedical applications.
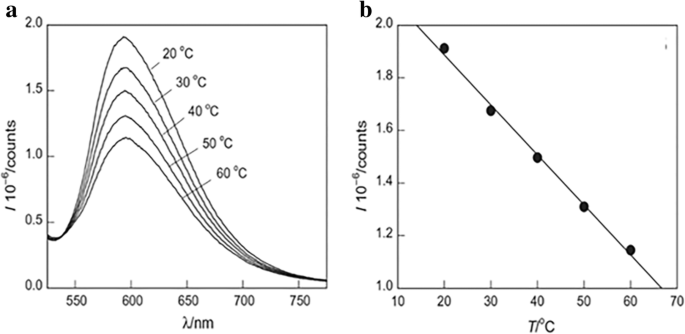
一 PL spectra of Ru (bpy)3@SiO2 under different temperature. b The peak intensity changes as a function of temperature [40]
图>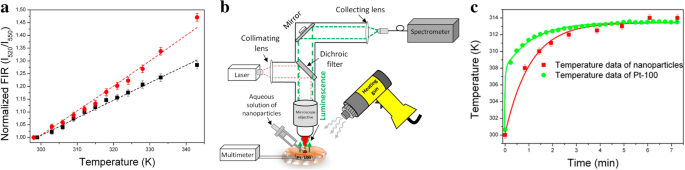
一 我 520/I 550 with different temperature for Er, Yb:GdVO4 and Er, Yb:GdVO4@SiO2. b The sketch map for the ex vivo temperature determination experiment. c The results of the temporal evolution of temperature for the Er, Yb:GdVO4@SiO2 and a thermoresistor Pt-100 [41]
图>Conclusion
In this article, LSNs with various functions demonstrate that silica is an ideal host material for luminescent dyes. The visualization of related parameters is the most special feature of luminescent dyes. Various luminescent materials have their own advantages but there are still some defects which limit their applications. Improved brightness, photostability, and thermal stability are the advantages of LSNs with the protection of silica. At the same time, it provides phosphors with a versatile platform which makes it possible to become multifunctional and specially modified. Excellent performance, adjustable adaptability, and potential versatility broaden the applications of fluorescent materials. LSNs have great potential in many unmentioned fields such as solar cells and photocatalysts. However, there is still a long way to apply LSNs to the actual species. Poor selectivity and low signal-to-noise ratio in complex conditions are factors that constrain LSNs for the practical applications which need to be further studied. Defined distances between phosphors and LSPR metal deserve more investigations to get the positive effect. Many new luminescent materials with excellent luminescence properties have been developed which means that it is necessary to improve the traditional synthetic methods to obtain LSNs. Silica is a traditional modified material but LSNs still have great potential for development.
缩写
- ACQ:
-
Aggregation-caused quenching
- AIEgen:
-
Aggregation-induced emission luminogens
- AMP:
-
Adenosine 5′-monophosphate
- An18:
-
An aggregation-induced emission-based organic fluorogen derivatized from 9,10-distyrylanthracene with alkoxyl endgroup
- APS:
-
(3-氨基丙基)三乙氧基硅烷
- APTES:
-
3-Aminopropyltriethoxysilane
- APTS:
-
(3-Aminopropyl)trimethoxysilane
- B:
-
Blue
- BAM:
-
Bio-anchored membrane
- CCT:
-
Corresponding correlated color temperature
- CD:
-
碳点
- CDSP:
-
Carbon dot-silica- phosphor composite
- CIE:
-
Commission Internationale de l’Eclairage
- CLSM:
-
Confocal laser scanning microscope
- CRI:
-
Color rendering index
- CTAB:
-
Cetyltrimethyl ammonium bromide
- CVD:
-
化学气相沉积
- DDT:
-
1-Dodecanethiol
- Dox:
-
阿霉素
- DPA:
-
2,2-Dipicolylamine
- F127:
-
Poly (ethylene glycol)-block-poly (propylene glycol)-block-poly (ethylene glycol)
- FIR:
-
Fluorescence intensity ratio
- FITC:
-
Fluorescein isothiocyanate
- FL-SiO2 :
-
Fluorescent mesoporous silica
- FRET:
-
Förster resonance energy transfer
- FSCHP:
-
Sensitive fluorescent sensor
- FSNP:
-
Fluorescent silica nanoparticle
- G:
-
Green
- H:
-
The ratio of water/TEOS
- HPTS:
-
8-Hydroxypyrene-1,3,6-tresulfonic acid
- HRTEM:
-
高分辨透射电子显微镜
- IgG1:
-
Anti-Escherichia coli
- KH-792:
-
N-(3-(trimethoxysiyl)propyl)ethylenediamine
- LEDs:
-
Ligh-emitting diodes
- LSN:
-
Luminescent silica nanoparticle
- LSPR:
-
Local surface plasmon resonance
- MPS:
-
3-Mercaptopropyltrimethoxysilane
- MPs:
-
Magnetic particles
- MQDs:
-
Magnetic quantum dots
- MRI:
-
Magnetic resonance imaging
- MTT:
-
Methyl tetrazolium
- 近红外:
-
近红外
- NTSC:
-
National Television System Committee
- OLEDs:
-
Organic light-emitting diodes
- OSNC:
-
Organosilica nanocrystal
- OTES:
-
n-Octyltriethoxysilane
- PBS:
-
磷酸盐缓冲盐水
- Pdots:
-
Polymer dots
- PEI:
-
Polyethyleneimine
- PET:
-
Photoinduced electron transfer
- PVA:
-
Poly (vinylalcohol)
- PVIS:
-
Poly (1-vinylimidazole-co-vinyltrimethoxysilane)
- QD655:
-
A kind of commercial quantum dots
- QD-LEDs:
-
Quantum dot-based light-emitting diodes
- 量子点:
-
量子点
- R:
-
Red
- R:
-
The ratio of water/surfactant
- RBL-2H3:
-
Rat basophilic leukemia mast cells
- SEM:
-
扫描电子显微镜
- TEM:
-
透射电子显微镜
- TEOS:
-
Tetraethoxysilane
- TPETPAFN:
-
A typical fluorogen consisting of two tetraphenylethylene pendants and an intramolecular charge transfer core
- TRITC:
-
Tetramethylrhodamine isothiocyanate
- UC:
-
Upconversion
- UCNP:
-
Upconversion nanoparticles
- UCNPs@SiO2@EuTP:
-
NaGdF4:Yb,Er@SiO2@Eu (TTA)3Phen
- 紫外线:
-
紫外线
- VTES:
-
Vinyltriethoxysilane
- WLED:
-
White light-emitting diode
纳米材料
- 钨及钨合金的应用
- 染料的应用分类
- 玻璃纤维的分类及应用
- 用于改进诊断和治疗应用的多功能金纳米粒子:综述
- 负载 ICA 的 mPEG-ICA 纳米颗粒的制备及其在治疗 LPS 诱导的 H9c2 细胞损伤中的应用
- 氧化铜纳米颗粒对大肠杆菌的生物合成、表征和抗菌潜力评估
- 生物传感器和纳米传感器在农业生态系统中的应用综述
- 小型硒纳米晶体和纳米棒的简便合成和光学特性
- 通过溶胶-凝胶工艺制备的纳米结构二氧化硅/金-纤维素-键合氨基-POSS 混合复合材料
- 涂有 CuS 纳米粒子的有色导电 CuSCN 复合材料的简便合成
- 水溶性α-NaGdF4/β-NaYF4:Yb,Er核壳纳米粒子的合成和发光特性
- 用于细胞内蛋白质递送的二氧化硅纳米颗粒:一种使用绿色荧光蛋白的新型合成方法