靶向基因沉默 BRAF 协同光热效应使用新型 GAL-GNR-siBRAF 纳米系统抑制肝癌细胞生长
摘要
肝癌是全球最常见的恶性肿瘤之一。 RAF 激酶抑制剂可有效治疗肝细胞癌 (HCC);因此,抑制 BRAF/MEK/ERK 通路已成为新型 HCC 治疗的新治疗策略。然而,针对肿瘤的靶向特异性递送系统仍然是临床应用的重大障碍。半乳糖 (GAL) 可以靶向在肝癌细胞上高表达的去唾液酸糖蛋白受体 (ASGPR)。在这项研究中,我们设计了一种新型多功能纳米材料 GAL-GNR-siBRAF,它由三部分组成,GAL 作为肝癌靶向部分,金纳米棒 (GNR) 在近红外光下提供光热能力,siRNA 专门沉默 BRAF (siBRAF )。纳米载体 GAL-GNR-siBRAF 显示出高 siRNA 负载能力并抑制血清中 siRNA 的降解。与裸金纳米棒相比,GAL-GNR-siBRAF 具有更低的生物毒性和更高的基因沉默功效。用 GAL-GNR-siBRAF 处理显着下调 BRAF 的表达并损害肝癌细胞的增殖、迁移和侵袭。此外,GAL-GNR-siBRAF 的组合光热效应和 BRAF 敲低有效地引起了肿瘤细胞死亡。因此,我们的研究开发了一种新型靶向多功能纳米材料GAL-GNR-siBRAF用于治疗肝癌,为开发新的临床治疗方法提供了思路。
介绍
肝细胞癌 (HCC) 是一个主要的全球健康问题 [1]。它是世界上第六大最常见的癌症,也是癌症相关死亡的第三大原因 [2, 3]。大多数 HCC 病例发生在东亚和撒哈拉以南非洲。然而,在一些发达国家,包括法国、英国、日本和美国,发病率一直在上升[4]。早期 HCC 的标准治疗方法是手术切除肿瘤。手术后的 5 年生存率可达 89% 至 93% [5]。不幸的是,只有一小部分 HCC 患者(约 20-30%)在早期被诊断出来;大多数 HCC 患者(> 70%)发现于晚期,无法进行手术切除。其他治疗选择包括肝移植、经导管动脉化疗栓塞 (TACE) 和全身化疗 [6]。但肝移植受供应限制,TACE栓塞不完全会导致治疗失败,全身化疗副作用显着,总体预后较差。因此,临床上对肝细胞癌的新治疗方法提出了很高的要求[7]。
RAS/RAF信号通路在肝癌的发生发展中起着重要作用,BRAF是该通路中必不可少的癌相关基因之一。这些基因的遗传改变通常会导致两种级联疾病。 RAS/RAF 信号通路的异常激活与癌症患者的不良预后有关 [8]。 BRAF 是 RAF 家族中突变频率最高的基因,靶向 RAS/RAF 通路是治疗 HCC 的新治疗策略 [8,9,10]。由于 RAF 激酶抑制剂索拉非尼已被证明可用于治疗 HCC,因此 BRAF 突变已成为 HCC 治疗的首选靶点 [8]。更具体地说,BRAF 突变已成为治疗晚期 HCC 的理想靶点,因为在亚洲、欧洲和美国的 HCC 治疗中已发现 RAF 激酶抑制剂索拉非尼的临床开发 [11]。与安慰剂相比,索拉非尼增加了总生存期,从而延长了晚期 HCC 患者的中位总生存期 [12,13,14]。然而,索拉非尼的肿瘤靶向能力较差,可导致高血压、脱发、恶心等不良副作用[15]。高特异性靶向肝癌并阻断RAF的药物仍有待进一步探索。
去唾液酸糖蛋白受体 (ASGPR),也称为肝半乳糖受体,是一种表达于肝细胞正弦表面的 C 型凝集素 [16]。 ASGPR 被认为是肝脏纳米结构的重要靶点,因为它在结合、内化和消除具有末端半乳糖残基的物质方面发挥着重要作用 [17]。据报道,几种单糖(半乳糖、甘露糖、乳糖、N-乙酰半乳糖胺和唾液酸)与 ASGPR 有不同程度的相互作用,半乳糖对 ASGPR 表现出更高的亲和力 [18]。由于 ASGPR 强烈暴露于肝实质细胞表面 [19],该受体对半乳糖和半乳糖基化前药或靶向肝脏的递送系统具有很强的亲和力。半乳糖纳米粒[20]、半乳糖胶束[21]和半乳糖脂质体[22]已被确定为肝脏靶向药物系统,专门针对肝细胞类癌细胞。
金纳米棒(GNR)是棒状的金纳米粒子,作为纳米载体具有显着的优势[23]。金纳米棒(GNR)具有优异的生物相容性,可用于稳定递送siRNA[24];它们具有较大的比表面积,可以灵活修改特定的肿瘤靶向接头 [25];局域表面等离子体共振(LSPR)在近红外光照射下具有较高的光热转换效率,已成为一种优良的光热抗肿瘤材料[26]。通过调整金纳米棒(GNR)的纵横比可以实现最大的光热效率。金纳米粒子的体外和体内毒性取决于它们的大小、表面电荷和表面涂层 [27]。然而,十六烷基三甲基溴化铵(CTAB)是合成金纳米棒必不可少的活性剂,具有明显的细胞毒性,限制了生物应用[28]。
RNA 干扰 (RNAi) 已成为一种很有前景的癌症治疗方法,因为它通过小干扰 RNA (siRNA) 有效地敲除或沉默靶基因 [29]。最近,siRNA 分子已进入人体试验阶段,被认为是治疗多突变基因癌症和肿瘤的有前途的方法 [30]。然而,siRNA的应用仍然面临着血清不稳定性(在细胞外环境中被核酸酶降解)和脱靶效应等巨大挑战[31]。此外,siRNA 带负电,这会阻止它们与带负电的细胞膜结合 [32]。由于这些特性,siRNA 本身不太可能直接传递给细胞。 siRNA的稳定性可以通过siRNA的化学修饰或将siRNA装载剂插入保护性载体材料中来提高[33]。
在这项研究中,我们新构建了一种多功能纳米载体 GAL-GNR-siBRAF。该系统以具有光学发热能力的金纳米棒为内核,外部修饰的 GAL(d-半乳糖)具有特异性靶向肝脏肿瘤。该系统降低了CTAB对金纳米棒表面的生物毒性,并表现出较高的siRNA负载能力,可有效沉默肝癌中的BRAF基因。 GAL-GNR-siBRAF的应用显着减弱了肝癌细胞的增殖、侵袭和迁移。此外,GAL-GNR-siBRAF同时诱导BRAF基因沉默和光热效应,在杀伤肿瘤细胞能力方面实现协同效应,为肝癌临床治疗的发展提供了新思路。
材料和方法
细胞系
小鼠肝癌细胞系Hepa1-6购自中国科学院干细胞库。细胞在含有 10% FBS 的 DMEM 培养基(Life Technologies, Carlsbad, CA)中于 37 °C 和 5% CO2 中培养。
BRAF siRNA的合成
靶向 BRAF 基因的 siRNA 序列是 5'-GCUUACUGGAGAGGAGUUACA-3',由 Dharmacon, Inc. (Lafayette, CO, USA) 合成。使用市售的靶向荧光素酶基因siGL2作为阴性对照siRNA。
CTAB-GNR的合成
使用种子介导的生长途径合成水溶性金纳米棒 [34]。种子溶液的制备如下:1 mL 十六烷基三甲基溴化铵 (CTAB) (0.2 M) (Sinopharm) 与 1 mL HAuCl4 ( 0.5 mM) (国药集团)。将溶液在28°C搅拌,充分混合,加入0.12 mL冷NaBH4(0.01 M)(国药控股),直到得到的种子溶液变成棕色并保留备用。然后如下合成生长溶液:将 50 mL CTAB (0.2 M) 和 50 mL HAuCl4 (1 mM)、2.5 mL AgNO3 (4 mM) (Sinopharm) 在 28°C 下轻轻混合。彻底温和混合后,待溶液颜色由深黄色变为无色时,加入670 μL抗坏血酸(0.079 M)。在28℃温和搅拌下加入120微升种子溶液,颜色由紫色逐渐变为紫黑色。恒温搅拌24 h后,12000 rpm离心10 min,除去多余的CTAB,真空冷冻干燥成GNR粉末备用。
合成 MUA-PEI 和 GAL-PEI-MUA
将巯基十一烷酸 (MUA; 654 mg) 溶解在 30 mL 的氯仿 (CHCl3) 中,然后与 100 mmol 1-乙基-3-[3-二甲氨基丙基] 碳二亚胺盐酸盐 (EDC) 和 100 mmol N-羟基琥珀酰亚胺 (NHS) 孵育在室温下放置 15 分钟。接着,将100 mmol聚乙烯亚胺(PEI)加入上述溶液中。室温反应24 h后,用等体积的去离子水提取水溶性PEI-MUA。用同样的方法完成水溶液中d-半乳糖的活化。然后,在上述水溶性PEI-MUA溶液中加入过量的活化d-半乳糖(500 mmol),室温下充分反应24 h,得到水溶性GAL-PEI-MUA复杂。
GAL-PEI-MUA-GNR (GAL-GNR) 和 PEI-MUA-GNR (PEI-GNR) 的合成
将冻干的 CTAB-GNR 粉末(10 mg)悬浮在 10 mL 的水溶性 PEI-MUA 或 GAL-PEI-MUA 复合物中。当在室温下搅拌 24 h 时,CTAB 被 Au-S 键取代。溶液以12,000 rpm离心15 min去除多余的上清液,沉淀用蒸馏水洗涤3次。冻干制备PEI-GNR或GAL-GNR粉末,称重溶解于无核酶水中,用多功能酶分析仪(Spectra Max M5e,MD,USA)观察等离子体共振效应。
透射电子显微镜
将 GNR 或 GAL-GNR 悬浮在蒸馏水中,再悬浮液置于铜网上。铜网上的样品在空气中彻底干燥30 min后,使用透射电子显微镜(LIBRA 120,Carl Zeiss,Germany)对铜网上进行成像。
紫外可见分析
GNR、PEI-GNR 或 GAL-GNR 悬浮在蒸馏水中。使用多功能酶标仪(SpectraMax M5e,MD,USA)检测纳米材料在600~900 nm波长范围内的表面等离子体共振效应。
Zeta 电位和流体动力学直径分析
将GNR、PEI-GNR或GAL-GNR悬浮在蒸馏水中,然后在Zetasizer Nano ZS上测量纳米材料的zeta电位和流体动力学直径。
核磁共振分析
将 GAL-GNR 冻干粉溶解在重水(99%,Sigma)中制成均匀的悬浮液,然后将悬浮液转移到 NMR 样品管中。 GAL-GNR的组成通过核磁共振(NMR)(Bruker,600mhz,Germany)测定。
凝胶迁移分析
GAL-GNR 和 siBRAF 根据质量比(0:1、1:1、2:1、3:1、4:1、5:1、6:1、7:1)混合。在室温下孵育 30 分钟后,将 DNA 上样缓冲液加入混合物中。将所有样品加入含有 0.01% Goldview(Bioshop,美国)的 2% 琼脂糖凝胶中,然后在 90 V 下电泳 30 分钟。 siBRAF包裹程度在凝胶成像系统上可视化。
血清中siRNA的稳定性
将 GAL-GNR-siBRAF (6:1) 的混合物在新鲜鼠血清中在 37°C 下分别孵育 0 h、3 h、6 h、12 h、24 h 和 48 h。裸siBRAF加新鲜鼠血清组在相同条件下处理。将等体积的 2% 十二烷基硫酸钠 (SDS) 添加到 GAL-GNR-siBRAF 溶液中。在室温下孵育 30 分钟后,加入 DNA 上样缓冲液,并将所有样品上样到 2% 琼脂糖凝胶(含有 0.02% Goldview(Bioshop,美国))并在 90 V 下电泳 30 分钟。 siBRAF的条带亮度在凝胶成像系统上可视化。
GAL-GNR-siBRAF 的竞争抑制分析
2 × 10 5 Hepa1-6 细胞在 12 孔微孔板中培养过夜。然后,细胞用乳糖酸预处理12 小时,然后用GAL-GNR--siBRAF(Cy3标记)转染30 分钟。荧光显微镜测定细胞内荧光强度(比例尺=200 μm)
GAL-GNR-siBRAF 的肝癌靶向递送能力
将一微克 cy3 标记的 siBRAF 与 PEI-GNR (30 μg/mL)、GAL-GNR (30 μg/mL) 在室温下孵育 20 分钟,然后添加到 Hepa1-6 细胞的培养基中。孵育30 min后,用50% FBS洗涤细胞3次,荧光显微镜下测定细胞内荧光强度。
光热效应
将 20、40、60、80 和 100 μg GAL-GNR 和 100 μg GNR 冻干粉完全溶解在 1 mL 蒸馏水中。将溶液加入24孔板中,用803 nm近红外激光源(2 W/cm 2 ) 15 分钟。前5 min每0.5 min用红外测温仪记录各组体温,然后每1 min记录一次体温。
MTT 分析
200 微升细胞 (3.5 × 10 4 /mL) 在 96 孔微孔板中培养 24 h,然后与不同浓度的 GNR 或 GAL-GNR(0、15、30、45、60、75、90、105、120 μg/mL)一起培养。孵育 24 或 48 小时后,将 20 μL MTT 添加到培养系统中并再孵育 4 小时。将紫色晶体溶解在 150 μL DMSO 中,然后在酶标仪(SpectraMax M5e,MD,USA)中使用 650 nm 参比在 490 nm 处进行分光光度分析。
Calcein-AM 和 PI 染色测试
Hepa1-6 细胞 (1.5 × 10 5 ) 在 12 孔板中保持过夜,并用 PBS、激光、GAL-GNR-siBRAF (30 μg/mL:1 μg)、GAL-GNR-siGL2 (30 μg/mL:1 μg) + 激光或 GAL 处理-GNR-siBRAF (30 μg/mL:1 μg) + 激光 4 h, 然后用近红外光 (808 nm, 2 W/cm 2 ) 15 分钟。通过钙黄绿素-AM(活细胞)和PI(死细胞)染色实验检测GAL-GNR的细胞毒性。荧光显微镜下观察到钙黄绿素-AM的绿色荧光和PI的红色荧光。
实时定量 PCR
使用 Trizol(Trizol 试剂,Invitrogen)提取细胞总 RNA,并用作 cDNA 合成的模板。在 Stratagene Mx3000P qRT-PCR 系统(安捷伦科技公司,列克星敦,马萨诸塞州,美国)中使用基因特异性正向和反向引物(每个 0.5 μL × 10 μM)进行 Q-PCR。根据制造商的方案使用 SYBR green PCR MasterMix (Life Technologies)。管家基因β-肌动蛋白用作内参。引物序列为β-肌动蛋白:5'AGGGAAATCGTGCGTGACATCAAA-3'(正向)和5'ACTCATCGTACTCCTGCTTGCTGA-3'(反向); BRAF:5'-CAATTGGCTGGGACACGGACAT-3'(正向)和 5'-TTGACAACGGAAACCCTGGAAAAG-3'(反向)。计算基因表达差异并用2 −∆∆Ct 表示 方法。
蛋白质印迹
Hepa1-6 细胞的总蛋白通过 RIPA 缓冲液(Cell Signaling,Pickering,Ontario,Canada)获得,并通过二辛可宁酸(BCA)定量。蛋白质在 12% SDS-PAGE 中分离并转移到聚偏二氟乙烯 (PVDF)。管家基因β-肌动蛋白用作检测BRAF蛋白表达的内参。 ECL化学发光法检测目的蛋白的蛋白条带和内参。
划痕测试
转染的 Hepa1-6(1.7 × 10 5 /mL) 细胞接种到 12 孔板中过夜。用 10 μL 移液器吸头刮擦完全粘附在板上的细胞。细胞用 PBS 洗涤两次并更换为含有 2% FBS 的培养基。 0、24、48 h后在显微镜下观察伤口线的平均值。每个划痕测试一式三份进行。
Transwell 迁移和入侵检测
8 微米的 Transwell 室(Corning Life Science)用于评估细胞迁移和侵袭。将悬浮在200 μL无血清培养基中的Hepa1-6以1.7×10 5 的密度加入上室 mL 细胞/孔,然后将 500 μL 含有 12% FBS 的培养基加入下室。孵育24-48 h后,用4%多聚甲醛溶液固定细胞30 min,然后用1%结晶紫溶液染色30 min。最后,在倒置显微镜下对细胞进行拍照和计数。细胞侵袭实验中,细胞侵袭实验需要ECM凝胶包被,其余步骤一致。
统计数据
所有实验一式三份进行。数据表示为平均值±标准差和学生的t 测试(双尾)来确定两种方法之间的差异。单向方差分析用于多组比较。数据在 p 处被认为具有统计学意义 <0.05 (*p <0.05, **p <0.01, ***p <0.001).
结果
纳米载体 GAL-GNR-siBRAF 的合成和表征
我们设计了一种新型纳米载体 GAL-GNR,能够将小干扰 RNA (siRNA) 输送到肝细胞并同时保持金纳米棒的光热效应。 GAL-GNR 的合成过程如图 1 所示。该肝脏靶向系统包含三个功能组件。首先,GAL-GNR 的基本部分是 GNR 骨架,其长度约为 30 nm,直径约为 10 nm,如 TEM 图像(图 1a)所示,化学共轭 GNR(GAL-GNR)显示无显着性尺寸变化并且仍然具有良好的分散性(图 1b)。粒径数据显示,GNR的平均粒径为30.23 nm,与电镜结果一致,GAL-GNR(50 nm)和PEI-GNR(42.35 nm)的粒径均大于GNR,因为GAL 和 PEI 的结合增加了颗粒之间的水合作用(图 1c)。其次,GNR 表面具有生物毒性的 CTAB 被带正电荷的 MUA-PEI 所取代,该 MUA-PEI 可以加载带负电荷的 siRNA。 zeta 电位测量表明,当 GNR 分别用 PEI 或 GAL-PEI 修饰时,GNR 的表面电荷从 35.6 增加到 42.7 mV 或 41.8 mV,表明 GAL-GNR 具有很强的结合 siRNA 的能力(图 1d)。第三,我们应用GAL作为引导分子结合GNR纳米载体,其可用于肝细胞癌的特异性归巢。紫外-可见吸收光谱用于初步检测改性GNR的结构。未改性GNR的初始吸收光谱波长为763 nm;最初用 MUA-PEI 修饰观察到 7 nm 的波长偏移,在合成结束时观察到另一个 8 nm 的偏移,当 GAL 成功修饰 GNR 时(图 1e)。为了确认纳米系统中的 GAL,使用 NMR 图像来分析化学基团。结果表明,半乳糖的H信号仅在NMR氢谱上的GAL-GNR谱中发现,即δ:3.60、3.65、3.70、3.78、3.90、4.53。 NMR谱证实了GAL的化学结构,其中GAL成功地与GNR表面结合(图1f)。
<图片>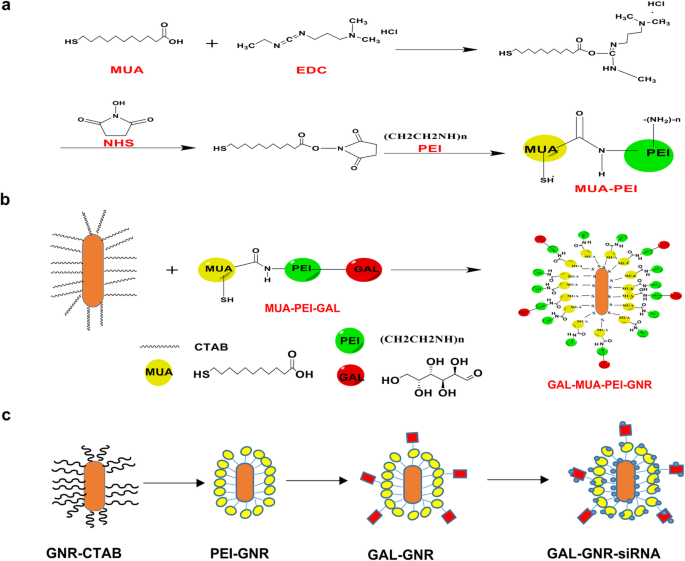
GAL-GNR 合成程序。 一 MUA-PEI的合成过程。 b d-半乳糖的活化和与 MUA-PEI 的化学反应。 c GAL-GNR的最终合成产物
图> <图片>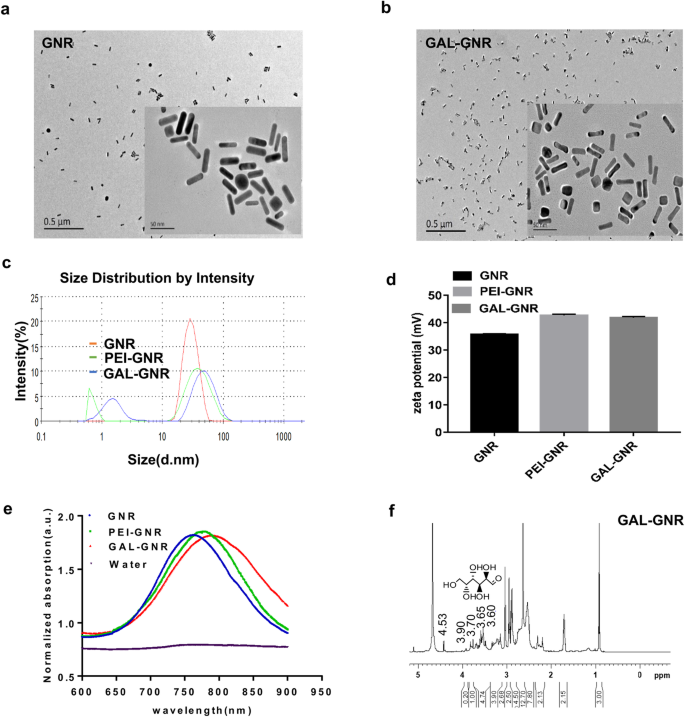
GNR 和 GAL-GNR 的表征。 一 GNR 的 TEM 显微照片(尺度 =0.5 μm/50 nm)。 b GAL-GNR 的 TEM 显微照片(尺度 =0.5 μm /50 nm)。 c 不同改性 GNR 的粒度分析。 d 不同修饰 GNR (GAL-GNR) 的 Zeta 电位分析。 e 不同改性 GNR 和水的归一化 UV-Vis 吸收光谱。 f GAL-GNR的NMR吸收光谱
图>GAL-GNR的siRNA封装能力和稳定性
GAL-GNR纳米骨架被带正电的PEI修饰,可以通过静电相互作用与带负电的siRNA结合。我们使用凝胶位移测定来评估 GAL-GNR 的 siRNA 结合能力。游离的 siRNA 可以沿着凝胶通道移动,而结合的 siRNA 会减慢或完全停止。此外,结合的siRNA将不再与溴化物有效结合,因此荧光强度将相应降低。凝胶电泳结果表明,用siBRAF饱和GAL-GNR的最佳比例为6:1(w/w)(图2a)。
<图片>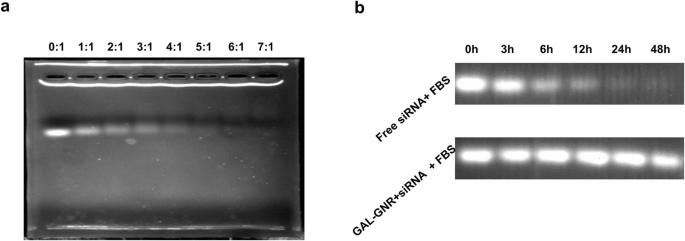
凝胶位移测定。 一 GAL-GNR 的 siRNA 负载能力。将 1 微克 siRNA 与不同质量比的 GAL-GNR 等体积混合。孵育 30 min 后,使用凝胶迁移试验评估 GAL-GNR 的 siRNA 负载能力。 b siRNA 在小鼠血清中的稳定性。将一微克 BARF siRNA 以 1:6 的质量比用 GAL-GNR 接种,然后加入到新鲜的鼠血清中。 37℃反应0、3、6、12、24、48小时后,GAL-GNR-siBARF中的siRNA用2%SDS提取,溴化乙锭观察
图>裸 siRNA 非常容易降解,尤其是在体内。传递系统的一个重要功能是保护 siRNA 免于降解。因此,我们测试了纳米载体 GAL-GNR 对新鲜血清中 siRNA 的保护作用。与裸siRNA相比,结合的siRNA在血清中储存的时间更长。如图 2b 所示,裸 siRNA 可以在血清中存在长达 12 小时,而结合的 siRNA 在 48 小时后仍然丰富。结果表明GAL-GNR能有效阻止siRNA在血清中的降解,保证体内靶向递送。
纳米材料的细胞毒性
低生物毒性是纳米材料的另一个重要特性,这使它们能够用于癌症治疗。为了评估这种新型 GAL-GNR 纳米结构的生物相容性,我们测试了其细胞毒性。如图 3 所示,未修饰 GNR 处理组中 Hepa1-6 的死细胞最初在 30 μg/mL 的低浓度下观察到。与75 μg/mL GNR孵育24 h后,细胞死亡率高达56.09%,孵育48 h后死亡率增加至75.5%。相反,GAL 修饰显着降低了细胞毒性。 GAL-GNR 孵育 48 h 后,即使在高浓度(105 μg/mL)下,仍有超过 80% 的细胞存活。这些数据表明纳米载体GAL-GNR与GNR相比具有较高的生物相容性,保证了应用的安全性。
<图片>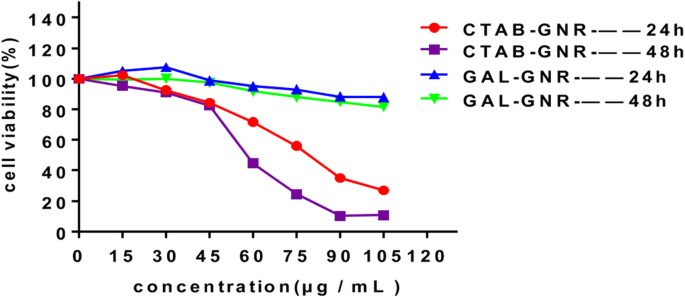
GAL-GNR 的毒性。 Hepa1-6 细胞在指定浓度下用 GNR (CTAB-GNR) 或 GAL-GNR 处理 24 或 48 小时。 MTT法测定细胞活力(n =5)
图>肝癌细胞靶向递送siRNA和体外BRAF基因沉默
将 siRNA 传递到癌细胞中的能力是纳米材料最重要的功能之一。为了研究 GAL-GNR 在 siRNA 递送中的潜力,我们在 Hepa1-6 细胞中使用 GAL-GNR 加载的 siBRAF 检测了基因沉默的功效。与空白组相比,GAL-GNR-siGL2(阴性对照)转染细胞中BRAF的表达无变化,而GAL-GNR-siBRAF转染细胞中BRAF的表达显着降低(图4a)。此外,沉默效应是剂量依赖性的,这与 GAL-GNR 浓度的增加有关。 qPCR结果表明,GAL-GNR-siBRAF的理想转染浓度为30 μg/mL,沉默效率可达90.29%,与lipofectamine 2000组(阳性对照)相似。 Western Blot结果与qPCR一致,转染48 h后BRAF蛋白表达下调(图4b)。
<图片>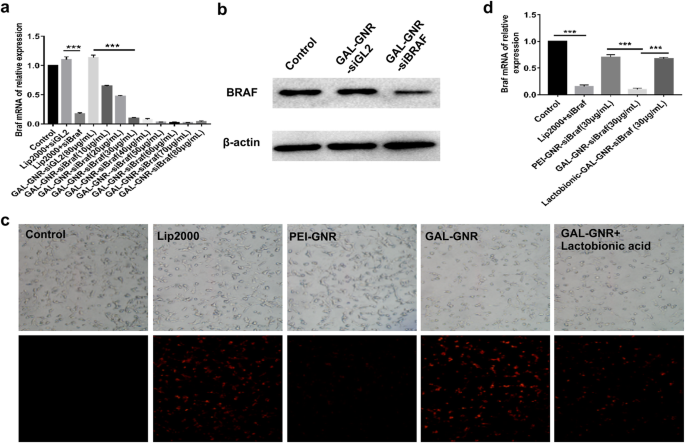
肝癌细胞靶向递送siRNA和体外BRAF基因沉默。 一 GAL-GNR-siBRAF转染后BRAF表达的mRNA。 Hepa1-6 细胞用不同浓度的 GA-GNR 转染,携带相同量的 BRAF siRNA (1 μg) 24 小时。通过qPCR测定BRAF mRNA的表达。误差棒代表三个实验的标准偏差 (***p <0.001)。 b GAL-GNR-siBRAF转染后BRAF在Hepa1-6中的蛋白表达。 Hepa1-6细胞用GAL-GNR-siBRAF、GAL-GNR-siGL2或PBS转染48 h,Western blot检测BRAF和β-actin水平。 c 和 d GAL-GNR-siBRAF 的肝癌靶向递送能力。将一微克 cy3 标记的 siBRAF 与 lip2000、PEI-GNR (30 μg/mL)、GAL-GNR (30 μg/mL) 在室温下孵育 20 分钟,然后添加到 Hepa1-6 细胞的培养基中。对于竞争性抑制测定,Hepa1-6 细胞用乳糖酸预处理过夜。在荧光显微镜下观察细胞内荧光强度(比例尺=200 μm),并通过q-PCR定量BRAF的mRNA表达。误差棒代表三个实验的标准偏差 (*p <0.05)
图>乳糖酸在结构上与GAL相似,可与表达于肝细胞癌细胞膜表面的去唾液酸糖蛋白受体(ASGPR)竞争性结合[35]。为了确认 GAL-GNR 的特异性靶向效率,将 Hepa1-6 细胞与乳糖酸预孵育,然后用 GAL-GNR-siBRAF 转染。未处理的细胞用作空白对照,经典转染试剂 lipofectamine 2000 用作阳性对照。如图4c所示,GAL-GNR转染细胞的红色荧光强度与阳性对照组相似,而PEI-GNR转染细胞的荧光强度明显降低。另一方面,单独转染 GAL-GNR-siBRAF 的细胞观察到 Cy3 的红色荧光比用乳糖酸预处理的 Hepa1-6 细胞更强(图 4c),qPCR 结果与红色荧光结果一致(图 4c)。图 4d)。这些结果进一步说明GAL有效地促进了Hepa1-6细胞对siRNA的内吞作用,可作为肝癌的靶向部分。
GAL-GNR-siBRAF 对 Hepa1-6 细胞增殖、迁移和侵袭的影响
BRAF 在 MAPK 通路中发挥重要作用,负责调节细胞增殖、迁移和侵袭 [36]。为了评估 GAL-GNR-siBRAF 对肝细胞癌细胞的影响,我们首先通过 MTT 测定分析了细胞增殖。如图 5a 所示,与 GAL-GNR-siGL2(阴性对照)或 PBS 处理的细胞相比,GAL-GNR-siBRAF 转染的 hepa1-6 细胞的增殖显着降低。 Next, we investigated the effect of GAL-GNR-siBRAF on cell migration by scratch and transwell assays. Compared with negative control cells, the migration ability of Hepa1-6 cells that transfected with GAL-GNR-siBRAF was evidently reduced (Fig. 5b–d).
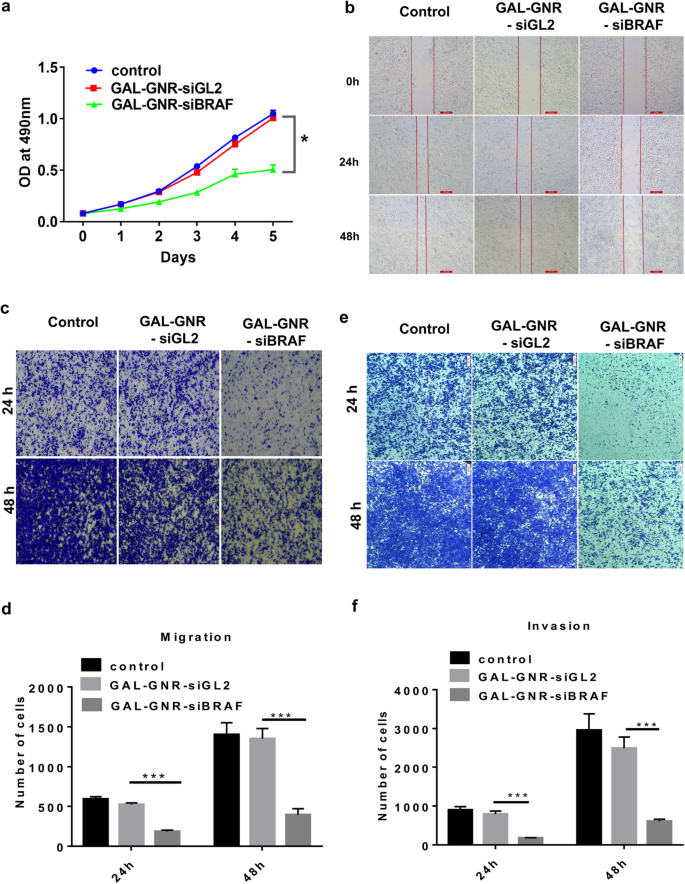
The impact of silencing BRAF gene on Hepa1-6 cells by GAL-GNR-siBRAF. 一 Proliferation of Hepa1-6 Cells. Hepa1-6 cells were transfected with GAL-GNR-siBRAF, GAL-GNR-siGL2, or PBS, and the cell proliferation was measured by MTT assay (n =5). The error bar represents the standard deviation of three experiments (*p <0.05)。 b Scratch test of Hepa1-6 cells. Hepa1-6 cells were transfected for 24 h as described above and then scraped with a 10 μL tip. The cell scratch images were taken at different time points (scale bar =200 μm). c 和 e Migration and invasion of Hepa1-6 cells. Hepa1-6 cells were transfected for 24 h as described above. Cell migration and invasion ability was determined by transwell assay, and imagines were taken at different time points (scale bar =200 μm). d 和 f Columnar statistical analysis of cell migration and invasive capacity (***p <0.001)
图>To further investigate the potential of GAL-GNR-siBRAF on the invasion, transwell assays were performed. The results showed that hepa1-6 cells transfected with GAL-GNR-siBRAF haven significantly decreased the invasion of Hepal-6, as compared with GAL-GNR-siGL2 or PBS (Fig. 5e and f). The above results implied that knockdown of BRAF gene using GAL-GNR-siBRAF complexes can effectively reduce migration and invasion of hepatocellular carcinoma cells.
Combination Treatment with Photothermal Effect and Gene Silencing of GAL-GNR-siBRAF
Our previous studies have demonstrated that gold nanomaterials can generate thermal energy under near infrared radiation [37]; this phenomenon is called photothermal effect due to LSPR properties of the GNR. Our results showed that, with the increase of GAL-GNR concentration, the heat production capacity of GAL-GNR was enhanced. When the concentration of GAL-GNR is 100 μg/mL, the temperature quickly rises from 24 to over 60 °C. In addition, there was no significant difference in heat production capacity between GNR and GAL-GNR (Fig. 6a).
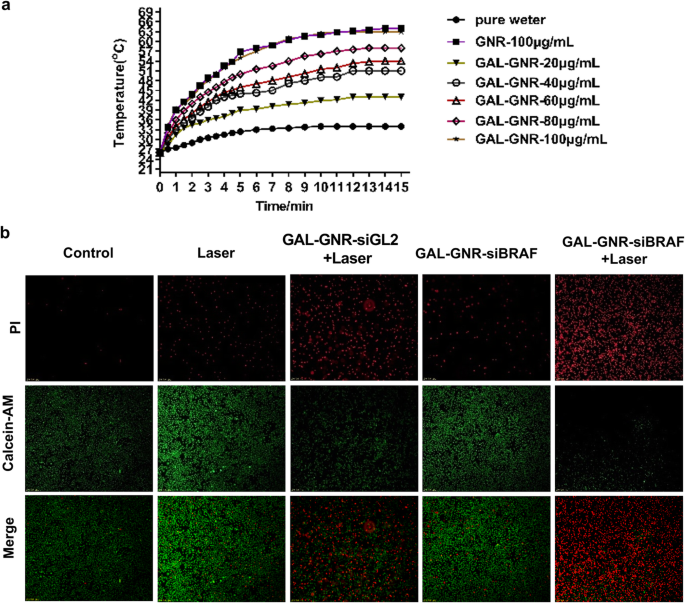
Photothermal effect induced by GAL-GNR and combination treatment of photothermal effect and gene silencing on liver cancer cells. 一 803 nm near-infrared laser source (2 W/cm 2 ) was used to irradiate the solution of GNR, GAL-GNR, or distilled water (blank control) for 15 min. The temperature was detected by infrared thermometer at different time point. b Hepa1-6 cells were treated with PBS, laser, GAL-GNR-siBRAF, GAL-GNR-siGL2 + Laser, or GAL-GNR-siBRAF + Laser, respectively. Green fluorescence of calcein AM (live cells) and red fluorescence of PI (dead cells) were observed under fluorescence microscope
图>The novel nano-system GAL-GNR-siBRAF possesses three individual characteristics:specific targeting of liver cancer, siRNA-based gene silencing of BRAF, and GNR-offered photothermal effects. Thus, we further study the synergistic therapeutic effect of GAL-GNR-siBRAF in anti-tumor treatment. Hepa1-6 cells without GAL-GNR kept higher viability even under near infrared radiation, suggesting laser itself had no significant effect on tumor cells. Once the laser irradiation was carried out on the basis of GAL-GNR-siGL2 treatment, the photothermal effect showed powerful killing ability on Hepa1-6 cells. Although BRAF gene silencing alone induced cell death, the effect was not conclusive. The treatment of cells with the nano-material GAL-GNR-siGL2 plus laser irradiation resulted in much stronger cell-killing ability than BRAF gene silencing individual (Fig. 6b). These data indicates that the BRAF gene silencing and photothermal effect can synergistically increase the potential of killing tumor cells.
Discussion
Difficulties in tumor-targeted delivery are major obstacles in the clinical treatment of tumors. This study demonstrated for the first time that we have developed a novel multifunctional nanocarrier GAL-GNR-siBRAF, and it was provided with many advantages. The modified GAL-GNR not only possessed good biocompatibility but also maintained the LSPR phenomena of GNR skeleton and still has excellent photothermal conversion ability. GNR modified with GAL can home hepatocellular carcinoma cells through ASGPR, which has great potential in targeted molecular therapy of hepatocellular carcinoma. Further studies showed that BRAF gene silencing inhibited the proliferation, migration, and invasion of hepatoma cells. It is worth noting that GAL-GNR-siBRAF shows a stronger cell killing ability in the combination of photothermal effect and gene silencing of BRAF.
RNA interference (RNAi) has attracted much attention due to its crucial role in gene expression and regulation. Using siRNA to knock down sequence-specific genes in cancer cells for gene therapy is an effective and specific targeted gene therapy, and which has become a new therapeutic tool for many diseases including cancer [29, 31]. However, low stability in serum and poor cell uptake limit siRNA in clinical application [38]. On the other hand, siRNA possess negative charge that prevents it from binding negatively charged cell membranes, and siRNA itself is unlikely to be delivered directly into cells [32, 39]. In addition, there are problems associated with low transfection efficiency and poor cell internalization in siRNA therapy [40]. These barriers impede the delivery of siRNA to target cells. To enhance siRNA stability and to strengthen gene silencing efficacy, we synthesized a novel nanocarrier, GAL-GNR-siBRAF, which includes three components (Scheme 1). This nanocarrier has low molecular size (about 30-nm longitude and 10-nm diameter) and good dispersibility (Fig. 1). Additionally, GAL-GNR-siBRAF could effectively load large amount of siRNA at low concentration (Fig. 2a) and protect siRNA degradation from RNase in serum; the siRNA was evidenced by stability for at least two days at 37 °C in the presence of fresh murine serum (Fig. 2b).
Good biocompatibility is essential for nanomaterials used in the field of biotherapy. CTAB is an essential active reagent in the synthesis of gold nanorods although it has apparent cytotoxicity that limits its biological application [41]. In order to reduce the cytotoxicity of the material, we manipulated the GNR by external modification of positive charge PEI and targeting adaptor GAL (d-galactose). Our results showed that, compared with unfunctionalized GNR, GAL-modified GNR has a better biocompatibility. The cell viabilities maintained over 80% even at very high concentrations (105 μg/mL) of GAL-GNR for 48 h (Fig. 3). In addition, the small molecular size and cylindrical shape of the nanorod facilitate its penetration through the cell membrane into the cell [37, 38, 42]. This phenomenon is particularly evident in targeted therapy of cancer cells. Nano-construct modified with the galactose-targeting moieties resulted in high accumulation of GAL-GNR-siBRAF in tumor cells, inducing effective downregulation of BRAF gene expression (Fig. 4). Moreover, according to the competitive inhibition experiments, we found that GAL-GNR entered cells mainly through ASGPR surface receptors of Hepa1-6 cells (Fig. 4c).
It was reported that HCC with high expression of BRAF and RAF1 tends to have rapid proliferation and growth [41, 43, 44]. B-Raf and Raf1 mainly act on the downstream of ERK/MAPK pathway, regulating nuclear factors through cascade amplification. Activation of this pathway accelerates the proliferation and differentiation of HCC cells abnormally [41, 44, 45]. RAF gene can also promote the expression of matrix metalloproteinases (MMPs), which can change the adhesion of tumor cells, degrade extracellular matrix (ECM) and basement membrane, and promote the invasion and metastasis of tumors [44, 45]. BRAF kinase regulates the RAS-RAF-MEK-ERK pathway, which promotes tumor cell proliferation, invasion and metastasis, and allows cell death through apoptosis [5, 46, 47]. In previous work, we found that BRAF gene was highly expressed in Hepa1-6 cells (data were not shown). It is speculated that the knock down of BRAF expression in Hepa1-6 will block the RAS-RAF-MEK-ERK pathway and lead biological changes of Hepa1-6. To verify our hypothesis, we transfected hepatocellular carcinoma cells with GAL-GNR-siBRAF and found that it can restrain the cell proliferation, migration, and invasion significantly (Fig. 5), providing a new strategy for clinical treatment of hepatocellular carcinoma.
Another important advantage of GNR is that it possesses local surface plasmon resonance (LSPR), which can convert absorbed light energy into heat energy under near infrared light irradiation, thereby killing and destroying cells [42]. Then, we explored the light-to-heat conversion ability of GAL-GNR, and the results showed that the modified GAL-GNR can induce heat energy effectively under the irradiation of near infrared light (Fig. 6a). Next, we further investigated the synergistic effects of GAL-GNR-siBRAF. Whereas BRAF gene silencing showed limited cytotoxicity, the treatment of GAL-GNR-siGL2 + laser had a much stronger killing ability on tumor cells. At the same time, the combination of photothermal hyperthermia and BRAF gene silencing could cause more than 85% cell death (Fig. 6b) which indicates that the synergy of GAL-GNR-siBRAF and photothermal effects could be an ideal strategy to inhibit liver cancer.
Conclusion
This study showed that GAL-GNR-siBRAF overcome the obstacle of siRNA degradation, effectively increased the stability of siRNA in serum in vitro. In addition, GAL-GNR-siBRAF can target deliver siRNA to hepatocellular carcinoma cells and knockdown the expression of BRAF and inhibit the cell proliferation, invasion, and migration significantly. More importantly, GAL-GNR-siBRAF, as a new multifunctional nanocarrier, can greatly enhance the ability of killing tumor cells when combined with near-infrared light. In conclusion, GAL-GNR-siBRAF has great potential in treatment of hepatocellular carcinoma and provides new ideas for clinical application of liver cancer.
数据和材料的可用性
All data generated and materials used in this study are included in the manuscript and corresponding additional files.
缩写
- GAL:
-
d-galactose
- GNR:
-
Golden nanorods
- siBRAF:
-
Small interfering RNA specific for BRAF
- HCC:
-
肝细胞癌
- ASGPR:
-
Asialoglycoprotein receptor
- TACE:
-
Transcatheter arterial chemoembolization
- LSPR:
-
局域表面等离子体共振
- CTAB:
-
cetyltrimethylammonium bromide
- MUA:
-
Mercaptoundecanoic acid
- EDC:
-
1-ethyl-3-[3-dimethylaminopropyl] carbodiimide hydrochloride
- NHS:
-
N-hydroxysuccinimide
- PEI:
-
Polyethyleneimine
- TEM:
-
透射电子显微镜
- NMR:
-
Nuclear magnetic resonance
- FBS:
-
胎牛血清
- DMSO:
-
Dimethyl Sulfoxide
- BCA:
-
Bicinchoninic acid
- PVDF:
-
聚偏二氟乙烯
纳米材料
- 负载 ICA 的 mPEG-ICA 纳米颗粒的制备及其在治疗 LPS 诱导的 H9c2 细胞损伤中的应用
- 131I 追踪的 PLGA-脂质纳米颗粒作为靶向化疗治疗黑色素瘤的药物递送载体
- 一种无需有机溶剂的靶向纳米药物增强抗癌功效的新方法
- 原位退火处理对基于 TIPS-并五苯的有机场效应晶体管的迁移率和形态的影响
- 斜角溅射 ITO 电极在 MAPbI3 钙钛矿太阳能电池结构中的影响
- 用 6-巯基嘌呤和神经元穿透肽修饰的金纳米颗粒促进 SH-SY5Y 细胞生长
- PEG 包覆的 CoFe2O4 纳米颗粒的毒性与姜黄素的处理效果
- 使用薄 Ti 中间层在非晶石英上直接生长基于 III 族氮化物纳米线的黄色发光二极管
- 圆形金纳米粒子:粒径和浓度对拟南芥根系生长的影响
- 使用双面金字塔光栅进行薄膜硅太阳能电池的有效光吸收
- 石胆酸修饰的金纳米粒子对肝癌细胞的凋亡作用
- 微小的稀土氟化物纳米粒子通过电极性相互作用激活肿瘤细胞生长