F-肌动蛋白通过 MKL1 和 YAP/TAZ 调节 TiO2 纳米管上间充质干细胞的成骨细胞分化
摘要
钛及钛合金广泛用于骨科植入物。修改纳米形貌提供了一种新的策略来改善钛基底的骨整合。丝状肌动蛋白(F-actin)聚合作为一种机械加载结构,通常被认为参与细胞迁移、内吞、细胞分裂和细胞形态维持。 F-肌动蛋白是否参与以及它如何在纳米管诱导的间充质干细胞(MSCs)成骨分化中发挥作用仍有待阐明。在这项研究中,我们通过阳极氧化在钛基底表面制备了 TiO2 纳米管,并通过扫描电子显微镜 (SEM)、X 射线能量色散分析 (EDS) 和原子力显微镜 (AFM) 表征了它们的特征。进行碱性磷酸酶 (ALP) 染色、蛋白质印迹、qRT-PCR 和免疫荧光染色以探索成骨潜力、F-肌动蛋白水平以及 MKL1 和 YAP/TAZ 的表达。我们的结果表明,随着阳极氧化电压从 30 V 增加到 70 V,TiO2 纳米管的内径和粗糙度增加,而它们的高度始终为 2 μm。此外,管径越大,TiO2纳米管促进MSCs成骨分化的能力越强。通过 Cyto D 抑制 F-肌动蛋白聚合可抑制 MSC 的成骨分化以及粘着斑复合物中所含蛋白质的表达,如纽蛋白 (VCL) 和粘着斑激酶 (FAK)。相反,在 Jasp 处理后,F-肌动蛋白的聚合增强了 RhoA 和转录因子 YAP/TAZ 的表达。基于这些数据,我们得出结论,TiO2 纳米管促进了 MSCs 的成骨分化,并且这种能力随着纳米管直径在一定范围内(30-70 V)的增加而增强。 F-actin 通过 MKL1 和 YAP/TAZ 介导这一过程。
介绍
钛及钛合金由于其优异的生物相容性、耐腐蚀性和机械性能,被广泛用于全关节置换和牙种植体等临床应用[1,2,3]。然而,仍有许多挑战有待解决,包括无菌性松动和感染 [4, 5]。近年来,已经进行了许多旨在增强骨整合和抗菌性能的研究。例如,钛种植体上的 MoS2/PDA-RGD 涂层不仅可以促进钛种植体与宿主骨的结合,还可以高效抑制细菌生长 [6]。此外,表面形貌也越来越受到关注,形貌修饰不同于化学修饰,只是改变了微米和纳米级的结构。化学信号对细胞的刺激不稳定且具有细胞毒性。相比之下,安全可控的物理信号可以避免一些化学分子带来的副作用。因此,种植体表面的形貌改变和通过形貌结构调节骨结合可以为解决假体植入后骨结合不良的临床问题提供新的途径。
在骨组织工程和骨再生领域,细胞-形态学相互作用被认为是精确控制种子细胞功能和分化的有前途的管理策略。同时,骨骼本身在纳米和微米范围内具有优雅的层次结构[7]。因此,表面形态可以提供类似的生态位,它可以模拟天然骨结构并促进宿主骨和植入物表面间充质干细胞的成骨分化。表面形态可以由许多不同的结构组成,包括纳米管、纳米线、纳米孔等。特别是纳米管阵列由于其独特的表面特性,如高比表面积、生物可塑性和高吸附能力,近年来在许多领域引起了广泛的兴趣。例如,一项新研究表明,氮化硼纳米管(BNNT)构成一种气敏材料,可用作气体传感器,通过检测油中溶解气体的成分和含量来监测变压器的运行情况[8]。在生物医学中,表面形貌还能够指导细胞行为,包括细胞迁移、粘附、增殖和分化。最新研究表明,纳米级拓扑结构可以引导间充质干细胞 (MSC) 分化为成骨细胞,从而加强早期骨整合 [9,10,11,12]。甚至有报道称,结合微米级和纳米级的表面修饰可以使间充质干细胞分化为收缩性平滑肌细胞 [13]。然而,表面形貌如何指导细胞命运的分子机制尚未阐明,这对材料安全性评估和材料设计具有重要意义。
丝状 (F)-肌动蛋白,也称为微丝,是真核细胞细胞骨架的三大主要成分之一。它由球状 (G)-肌动蛋白聚合物组成,并被许多其他蛋白质修饰。 F-肌动蛋白具有结构极性,因为所有微丝的亚基都指向同一端。带倒刺的末端指向不同的相邻单体,而尖端具有暴露 ATP 结合位点的肌动蛋白亚基。也就是说,ATP 参与了 G-actin 和 F-actin 之间的转化过程。该过程处于动态平衡状态,聚合和解聚同时发生,也称为踏步,常见于片状伪足和丝状伪足[14]。因此,很明显,肌动蛋白动力学在细胞迁移、细胞分裂和细胞形状维持等细胞功能中起着重要作用。然而,F-肌动蛋白不仅作为支持机械负荷的物理结构,还参与其他生物学行为,如信号转导和基因表达。越来越多的证据表明,F-肌动蛋白可以通过与其他蛋白质相互作用将物理信号转化为化学信号 [15,16,17,18]。例如,生物力学和几何重建通过阻止肌动蛋白单体聚合与 F-肌动蛋白的叠加来促进肿瘤细胞凋亡 [15]。超声脉冲通过抑制 F-肌动蛋白的解聚来增强人类间充质干细胞的成骨 [16]。我们之前的研究还表明,机械应变会增加 F-肌动蛋白的稳定性 [17]。由于纳米管的中空结构为细胞提供了较少的粘附位点,因此为了维持生物力学平衡,细胞骨架的重排是不可避免的。因此,我们完全有理由相信F-actin可能介导纳米形貌诱导的细胞分化。
在这项研究中,我们制造了 TiO2 纳米管,通过阳极氧化改变了它们的形貌,并探索了它们促进 MSCs 成骨分化的能力。接下来,我们研究了 F-肌动蛋白是否在机械转导中起关键作用。细胞松弛素 D (Cyto D) 与 F-肌动蛋白的倒刺末端竞争性结合以防止 G-肌动蛋白掺入细丝,用于抑制 F-肌动蛋白聚合,而茉莉花内酯 (Jasp) 用于增强 F-肌动蛋白的稳定性肌动蛋白组装。此外,我们还想阐明 F-肌动蛋白如何将物理线索转化为生化信号。基于我们之前的研究结果,我们假设 MAPK 通路可能参与了这个过程 [17]。转录因子,如 Yes 相关蛋白 (YAP)/具有 PDZ 结合基序 (TAZ) 和 MKL1 的转录共激活因子,它们被认为是机械传感器和机械转导器,也是我们研究的主题,以筛选 F-肌动蛋白如何影响干细胞命运,因为其他领域的一些研究暗示它们与 F-肌动蛋白有关 [19,20,21]。总的来说,我们希望阐明F-actin在纳米管诱导干细胞分化过程中的作用,以指导纳米管修饰植入物的材料设计和生物安全性评估。
材料和方法
TiO2 纳米管的制造
纯钛片(纯度 99.9%,厚度 2 mm;中国河北盛世达)用 400 号和 1500 号碳化硅砂纸打磨。然后在超声波清洗机中依次用丙酮、无水酒精和去离子水清洗样品,最后在室温下干燥3小时。为了制造纳米形貌,将预处理过的样品固定为阳极,同时使用铂片作为反阴极,在 0.15 M NH4F 和 90% 乙二醇的电解质水溶液中放置 1 小时。阳极氧化电压为 30、40、50、60 或 70 V 的恒定电压。阳极氧化后,每个样品用去离子水冲洗 30 分钟,然后在超声波清洗机中用无水酒精清洗 15 分钟。最后,所有样品在120 ℃高压釜中灭菌1 h,然后用培养基润湿后使用。
纳米管制备的反应机理尚不清楚,目前主流的理论是场增强溶解理论。纳米管阵列的形成是在场氧化、场溶解和化学溶解作用下动态平衡的结果(图1b)。阳极氧化过程可描述如下:第一步,在电解质-金属界面上形成氧化物阻挡层:
$$ {\mathrm{Ti}}^{4+}+2{\mathrm{H}}_2\mathrm{O}\to {\mathrm{Ti}\mathrm{O}}_2+4{\mathrm{ H}}^{+} $$ (1)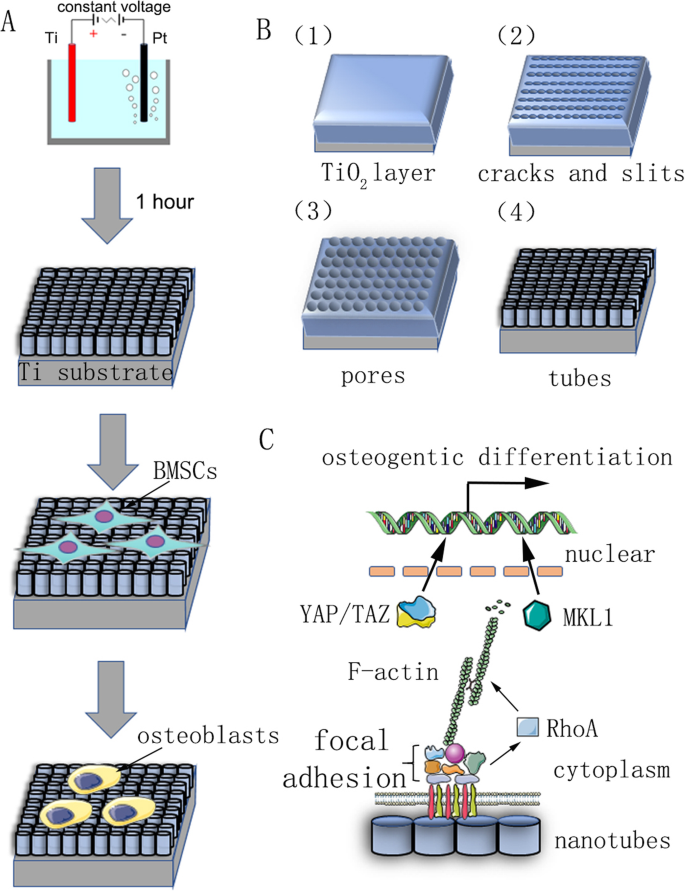
我们研究的总结图表。 一 显示阳极氧化和细胞分化诱导的流程图。 b 将预处理过的钛基材作为阳极固定在含有 0.15 M NH4F 和 90% 乙二醇的电解质水溶液中,在恒定电压下保持 1 小时。预计自组装纳米管会均匀形成。其反应机理在材料和方法中有描述。 c 纳米管诱导干细胞成骨分化机制示意图
图>然后,由于氧化层的场增强溶解,在表面上出现裂纹和窄缝。 F − 的扩散 离子进入这些裂缝和狭缝会提高溶解速度。裂缝扩大并与相邻的裂缝相连。最后,氧化钛层的形成速率和溶解速率达到动态平衡,纳米管不再生长:
$$ {\mathrm{TiO}}_2+6{\mathrm{F}}^{-}+4{\mathrm{H}}^{+}\to {\left[{\mathrm{TiF}}_6 \right]}^{2-}+2{\mathrm{H}}_2\mathrm{O} $$ (2)表面表征
用不同电压(30、40、50、60 或 70 V)制造的样品用乙醇和去离子水冲洗 15 分钟,然后在室温下干燥。扫描电子显微镜(SEM450,FEI Nova Nano SEM;Thermo Fisher Scientific,Waltham,MA,USA)用于表征表面结构,并在样品涂上一层薄金后测量纳米管的内径和高度。同时,进行 X 射线能量色散分析 (EDS) 以分析纳米管的元素组成。原子力显微镜(AFM,NanoManVS,Bruker Nano Surfaces,Bruker MicroCT,Kontich,比利时)用于研究样品的表面形态和表面粗糙度。每个样品选取3个不同区域,重复测量3次。
细胞培养
4 周龄雄性 Sprague-Dawley (SD) 大鼠购自上海第九人民医院(中国上海)实验动物中心。从股骨和胫骨中无菌分离大鼠骨髓间充质干细胞 (BMSCs)。 BMSC 在含有 10% (v/v) 胎牛血清 (FBS)(Gibco/Life Technologies,Carlsbad,CA,USA)的 α-最低必需培养基(α-MEM;Hyclone,Logan,UT,USA)中纯化并进一步扩增)、100 mg/mL 链霉素 (Gibco) 和 100 U/mL 青霉素 (Gibco),并在 37 °C 下在由 95% 空气和 5% CO2 组成的加湿气氛中培养。每 2 天更换一次培养基,将细胞胰蛋白酶消化并传代培养至 80% 汇合。本研究中使用的所有细胞都在第 3 代和第 5 代之间。成骨诱导培养基由补充有 100 nM 地塞米松、10 mM β-甘油磷酸和 50 mM 抗坏血酸(Sigma-Aldrich,St Louis,MO,USA)的生长培养基组成.
细胞增殖分析
将TiO2纳米管修饰的钛片切成圆形,放入24孔细胞培养板的孔中。 3-5代之间的BMSCs在TiO2纳米管上以3×10 4 的密度培养 细胞/盘在生长培养基或成骨培养基中。细胞培养 2 天后,将用于破坏 F-肌动蛋白聚合的细胞松弛素 D(Cyto D,Sigma-Aldrich)和茉莉花内酯(Jasp,Sigma-Aldrich)每天添加到培养基中,持续 3 天。 Cyto D 和 Jasp 的终浓度和作用时间分别为 5 μM、1 h 和 2 μM、3 h。与试剂孵育后更新培养基。在 Cyto D 或 Jasp 处理后 12 小时,使用 Cell Counting Kit-8 (CCK8) 测定法(Dojindo,Kumamoto,Japan)评估细胞活力和增殖。将细胞与 10% (v/v) CCK8 溶液在细胞培养箱中于 37°C 和 5% CO2 下孵育 2 小时。然后我们将 100 μL 反应混合物转移到 96 孔板的孔中,并使用 Multiscan 紫外可见分光光度计(Safire2;TECAN,Mannedorf,Switzerland)在 450 nm 处测量培养物中甲臜染料产物的吸光度(OD) )。此外,我们还使用自动细胞计数器(AMQAX1000,Life Technologies)计算了近似细胞数。在细胞计数之前,将 BMSCs 从 TiO2 纳米管上酶促分离并用台盼蓝(Sigma-Aldrich)染色。
碱性磷酸酶染色和 ALP 活性分析
将 BMSCs 以 3 × 10 4 的密度接种到五种不同的 TiO2 纳米管改性钛切片(30、40、50、60 或 70 V)上 每孔并在成骨培养基中培养。如上所述添加试剂。培养7天后,将在TiO2纳米管上培养的BMSCs用PBS洗涤3次,用4%多聚甲醛固定,按照制造商的说明(虹桥,上海,中国)在ALP试剂盒的碱性磷酸酶(ALP)工作液中培养. PBS洗涤后在体视显微镜下观察结果。
对于 ALP 活性分析,首先用不含蛋白酶和磷酸酶抑制剂的 RIPA 缓冲液裂解细胞,然后按照提供的方案使用 ALP 检测试剂盒(Beyotime Institute of Biotechnology,中国江苏)对离心后的裂解物进行检测。最后将活性归一化为相应裂解物的蛋白质浓度。
免疫细胞化学
Cyto D和Jasp处理3天后,BMSCs用4%多聚甲醛在室温下固定20分钟,然后用PBS洗涤3次。细胞用 0.3% Triton-X 100 透化 30 分钟,用 PBS 洗涤 3 次,并在室温下用罗丹明缀合的鬼笔环肽染色 1 小时。然后用 PBS 冲洗细胞,并在室温下用 DAPI(Beyotime Institute of Biotechnology)复染 10 分钟。用 PBS 洗涤 3 次后,将样品固定在载玻片上,共聚焦显微镜观察。
蛋白质印迹
为了评估蛋白质表达,用胰蛋白酶 (Gibco) 收获在 TiO2 纳米管上培养的 BMSC。细胞用 PBS 洗涤 3 次,并用补充有蛋白酶和磷酸酶抑制剂混合物的 RIPA 缓冲液在冰上裂解 30 分钟。通过以 12,000×g 离心收集裂解物 在 4°C 下保持 15 分钟。根据制造商的说明,使用二辛可宁酸 (BCA) 蛋白质测定试剂盒 (Beyotime) 测量上清液中总蛋白质的浓度。将上样缓冲液加入上述蛋白质样品中,然后在 95°C 下煮沸 15 分钟。对于蛋白质印迹分析,将 10 μL 蛋白质制剂加载到 12.5% SDS-PAGE 凝胶(EpiZyme Inc.,Cambridge,MA,USA)上并在 120 V 下进行电泳 1 小时,然后电转移到聚偏二氟乙烯上(PVDF) 膜在 250 mA 下保持 2 小时。然后将膜在室温下用 TBST 中的 5-10% 脱脂奶粉在振荡器上封闭 1 小时,并与稀释缓冲液 (Beyotime) 中稀释的一抗在 4°C 下孵育过夜。 TBST洗涤3次5分钟后,加入稀释缓冲液稀释的荧光偶联二抗,室温避光孵育1小时。通过双色红外荧光成像系统(Odyssey,LiCor Biosciences,Lincoln,NE,USA)检测蛋白质条带。特别是,如果内参蛋白的条带是统一的,则将膜剥离并用另一种一抗重新探测,然后进行相同的过程。我们使用 GAPDH 抗体作为我们的内部参考蛋白来标准化蛋白质表达,本研究中使用的其他一抗是抗纽蛋白(1:1000 稀释,Abcam,Cambridge,MA,USA)、抗 FAK(1:1000 稀释) , Cell Signaling Technology, Danvers, MA, USA)、抗 Runx2(1:1000 稀释,Cell Signaling Technology)、抗 RhoA(1:1000 稀释、Cell Signaling Technology)、抗 F-肌动蛋白(1:500 稀释) , Abcam)、抗 Osx(1:500 稀释,Abcam)和抗 pYAP(1:1000 稀释,Cell Signaling Technology)。二抗为山羊抗小鼠 IgG H&L(IRDye® 680RD,1:5000 稀释,Abcam)和山羊抗兔 IgG H&L(IRDye® 680RD,1:5000 稀释,Abcam)。
定量实时 PCR
在第 7 天进行定量实时 PCR 以评估 runt 相关转录因子 2 (Runx2)、Osterix (Osx)、Alp、骨钙素 (OCN)、RhoA、YAP、TAZ、vinculin (VCL)、黏着斑激酶 (FAK) 和巨核细胞白血病 1 (MKL1) 在 TiO2 纳米管上的成骨培养基中生长的细胞中。使用总 RNA 试剂盒(R6812-01HP,Omega Bio-Tek Inc.,Norcross,GA,USA)从细胞中提取总 RNA。 RNA 样品的浓度和纯度通过 260 波长下的光密度确定,仅分析 A260/280 比和 A260/230 比均高于 1.8 的样品。根据制造商的说明,使用 qScript cDNA 合成试剂盒(Takara,Shiga,Japan)将 RNA 样品逆转录成 cDNA。使用 QuantStudio 6 Flex 实时 PCR 系统(Life Technologies)使用 SYBR® Premix Ex Taq™(Takara)进行定量实时 PCR。 GAPDH,一种看家基因,被用作内部参考。使用比较 Ct (2 -ΔΔCt ) 方法并表示为与对照相比的倍数变化。所用引物序列见表1。
图>统计分析
除非另有说明,所有数据均代表使用一式三份样品的至少三个独立实验。数据表示为平均值 ± 标准偏差 (SD)。通过单向方差分析评估组间差异,然后是 Student-Newman-Keuls 事后检验或 Student t 测试。 P <0.05 的值被认为具有统计学意义。
结果
表面表征
为了制造纳米形貌,通过使用阳极氧化设备在不同的恒定电压(30、40、50、60 和 70 V)下持续 1 小时,在纯钛基底上形成 TiO2 纳米管(图 1)。通过扫描电子显微镜 (SEM) 观察到均匀分布的自组装纳米管阵列。纳米管的侧视图和俯视图如图 2a、b 所示。本研究中所有样品中纳米管的高度约为 2 μm,而纳米管的内径约为 74 nm (30 V)、92 nm (40 V)、112 nm (50 V)、128 nm (60 V) 和 148 nm (70 V)(图 2c)。这表明纳米管的高度与阳极氧化时间有关,内径与阳极氧化电压有关。然后进行 X 射线能量色散分析 (EDS) 以分析纳米管的元素组成。这表明纳米管仅由两种元素 O 和 Ti 组成(图 2d、e)。原子力显微镜(AFM)用于检测纳米管结构并测量纳米管轮廓的算术平均偏差作为表面粗糙度(Ra)(图2f)。数据表明,纳米管的表面粗糙度随着直径(即阳极氧化电压)的增加而增加(图2g)。
<图片>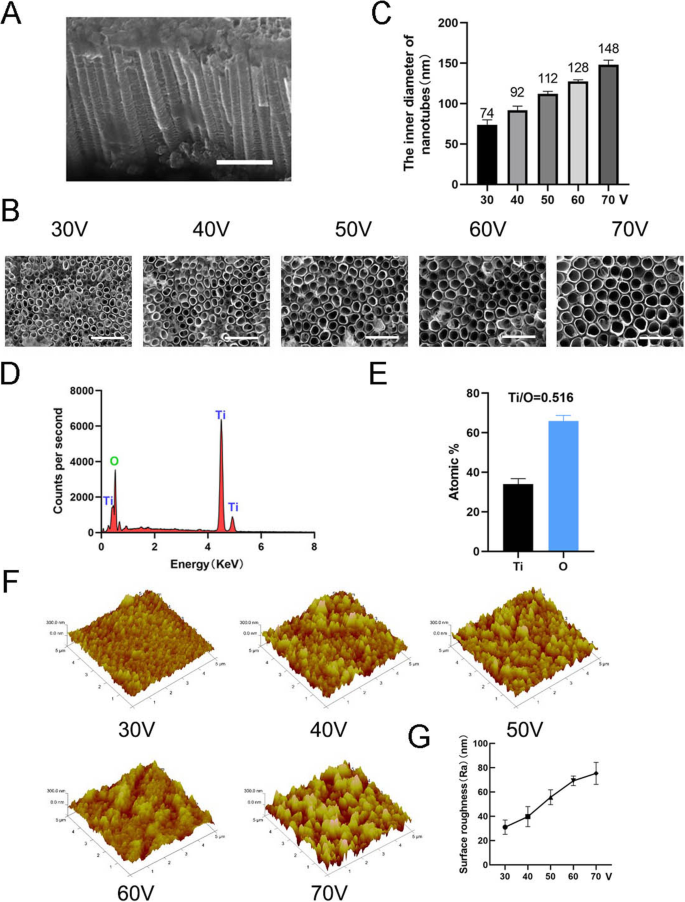
纳米管的表面表征。 一 纳米管的侧视图。比例尺:1 μm。 b 纳米管的顶视图。比例尺:500 nm。 c 在五种不同的恒定电压(30、40、50、60、70 V)下纳米管的内径。 d 纳米管结构的 EDS 化学元素组成。 e Ti和O的元素比。f 纳米管结构的原子力显微镜 (AFM) 图像。 g 纳米管的平均表面粗糙度(Ra)
图>TiO2 纳米管诱导间充质干细胞成骨分化
成骨诱导 7 天后,首先进行 ALP 染色以评估 MSCs 的成骨分化。染色结果表明,在TiO2纳米管上培养的MSCs比在光滑钛基底上培养的细胞(对照组)具有更高的ALP活性(图3a)。染色区域的统计分析表明,与对照组相比,纳米管诱导成骨分化的能力显着增强。同时,我们观察到一个趋势,在本实验的直径范围内,TiO2 纳米管的直径越大,诱导成骨分化的能力越强(图 3b)。因此,在后续实验中使用 70 V 组以更好地显示结果。接下来,我们分析了第 3 天和第 7 天的成骨基因表达。与对照组相比,在 TiO2 纳米管上培养 3 天和 7 天的 MSC 均显示出显着促进成骨基因(RUNX2、ALP、OCN 和 OSX)的表达(图 3d-g)。蛋白质印迹结果证实 RUNX2 和 OSX 的蛋白质表达在骨诱导 7 天后也增加(图 3c)。有趣的是,我们发现 F-肌动蛋白在 TiO2 纳米管组中被上调。因此,很明显 TiO2 纳米管将 MSCs 导向成骨细胞分化,这与纳米管的直径有关。我们的研究结果还表明 F-actin 参与了这一过程。
<图片>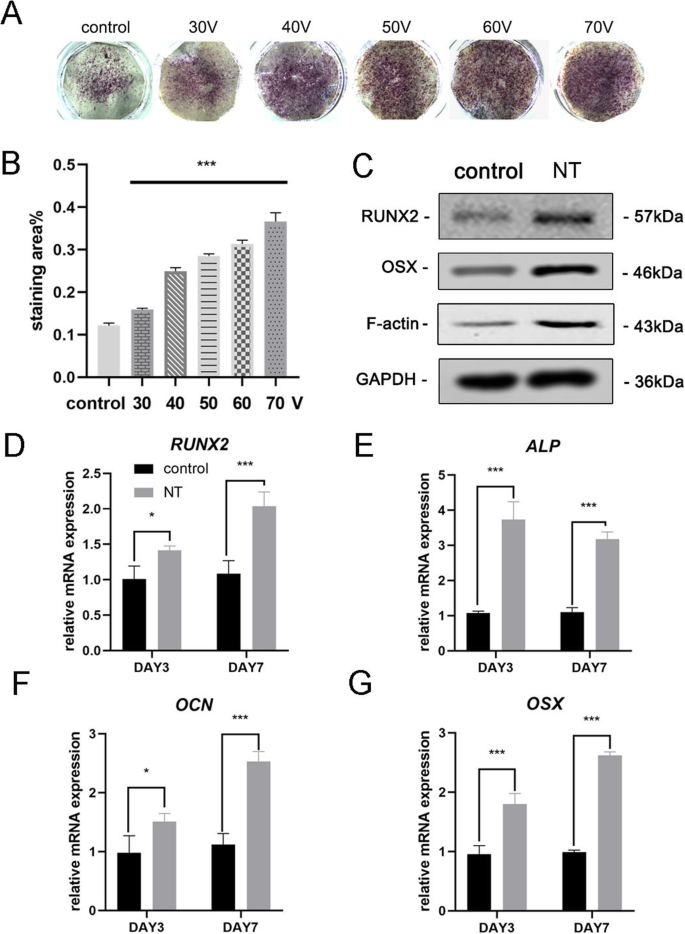
TiO2 纳米管增强了 BMSCs 的成骨基因表达。 一 平滑钛基材和五种不同的纳米管基材的 ALP 染色。细胞用成骨培养基诱导7天。 b 染色面积的统计分析使用 ImageJ 进行。 c 在第7天通过Western印迹分析MSC中的成骨相关蛋白(RUNX2和OSX)和F-肌动蛋白。RUNX2的mRNA表达(d ), ALP (e ), OCN (f ) 和 OSX (g ) 在第 3 天和第 7 天,通过 qRT-PCR 进行分析。 新台币 纳米管组。数据代表三个样品的平均值 ± SD。 *P <0.05, **P <0.01 和 ***P <0.001
图>F-肌动蛋白介导的 TiO2 纳米管上 MSCs 的成骨细胞分化
为了进一步探索 F-肌动蛋白是否参与纳米拓扑诱导的细胞分化,我们使用了两种试剂 jasplakinolide (Jasp) 和细胞松弛素 D (Cyto D),分别以正和负方式调节 F-肌动蛋白聚合。罗丹明-鬼笔环肽染色的共聚焦显微照片显示,Cyto D 处理组中的 F-肌动蛋白几乎解聚,纤维结构很少见,而 Jasp 稳定和聚合 F-肌动蛋白,证实了比观察到的更清晰、更明亮的束状结构在对照组中(图 5a)。此外,Western blot分析也证实了F-actin的蛋白表达受到影响,证明Cyto D和Jasp都如预期的那样起作用(图5b)。细胞增殖试验表明,Cyto D 显着抑制细胞增殖,而 Jasp 促进细胞生长(图 4a)。细胞计数结果与该发现一致(图4b)。
<图片>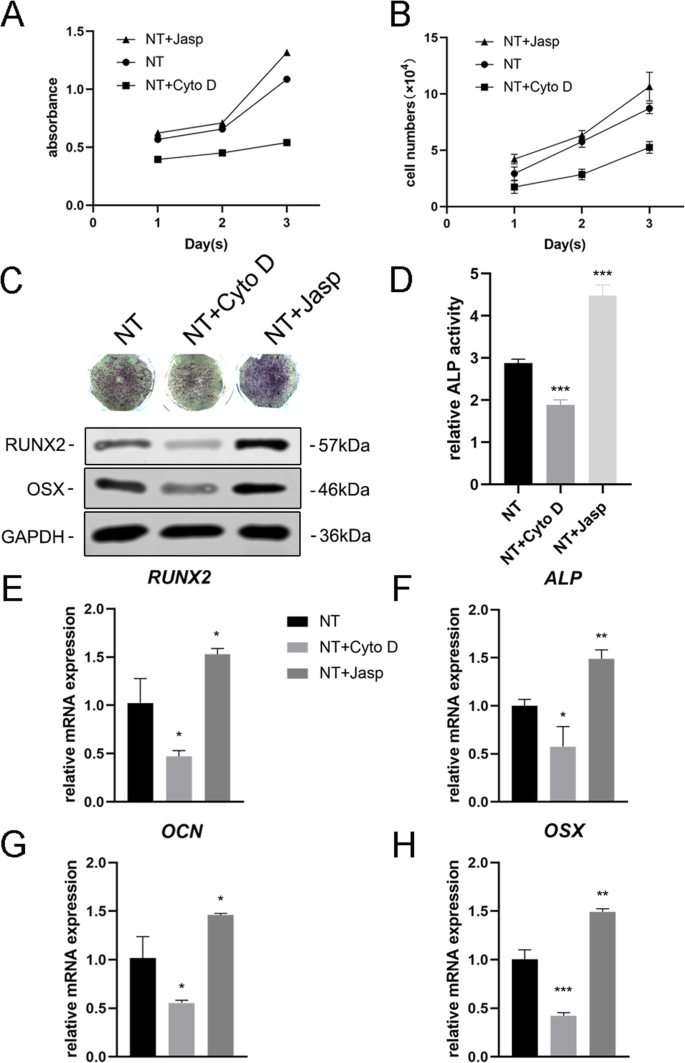
F-肌动蛋白组装调节 BMSCs 中成骨基因的表达。 一 , b 在第 1、2 和 3 天使用 CCK-8 测定或自动细胞计数器测定 Cyto D 和 Jasp 处理后的细胞增殖。c , d 进行ALP染色和ALP活性分析以评估药物处理3天后MSCs中ALP的表达。使用 ImageJ 分析染色区域。 c , e –h 采用Western blotting和qRT-PCR比较NT+ Cyto D组和NT+ Jasp组与对照组(未药物治疗)成骨相关标志物的变化。 新台币 纳米管组。数据代表三个样品的平均值 ± SD。 *P <0.05, **P <0.01 和 ***P <0.001
图>接下来,我们评估了 MSCs 分化为成骨细胞的能力,以研究 F-肌动蛋白是否介导了这一过程。我们首先检测到 ALP 作为成骨的早期标志物。与对照组相比,Cyto D 处理降低了 ALP 的表达及其活性,而 Jasp 处理组则上调(图 4c、d)。与该结果一致,Jasp 处理导致 RUNX2 和 OSX 的蛋白质水平增加,而 Cyto D 具有相反的效果(图 4c)。与此一致,骨特异性基因(包括 RUNX2、ALP、OCN 和 OSX)的 mRNA 表达水平在药物治疗后显示出相同的趋势(图 4e-h)。最重要的是,这些数据表明 F-肌动蛋白在 TiO2 纳米管诱导的 MSCs 成骨分化过程中起重要作用。促进F-肌动蛋白解聚抑制纳米形貌诱导的成骨细胞分化,而F-肌动蛋白的稳定和聚合促进成骨细胞分化。
F-肌动蛋白通过 MKL1 和 YAP/TAZ 在 TiO2 纳米管上调节 MSCs 的成骨细胞分化
为了剖析涉及 F-肌动蛋白调节 MSC 命运的潜在机制,我们研究了直接与 F-肌动蛋白相互作用或影响 F-肌动蛋白聚合的蛋白质/分子。首先,我们试图确定纳米形貌如何影响 F-肌动蛋白和 G-肌动蛋白之间的平衡。 TiO2 纳米管作为物理信号不同于可渗透膜的化学信号,因此必须使用细胞膜的某些成分将刺激传递到细胞中。越来越多的证据表明,粘着斑复合物,包括整联蛋白、talin、粘着斑激酶 (FAK)、纽蛋白 (VCL)、张力蛋白和其他蛋白质,作为信号载体,将细胞外基质 (ECM) 的状况告知细胞),从而影响它们的生物学行为 [22, 23]。更重要的是,F-肌动蛋白通过这种粘着斑复合物与整联蛋白结合,从而在细胞内肌动蛋白束和 ECM 之间形成机械连接 [24]。因此,我们接下来分析了粘着斑复合物组分的表达。结果表明,VCL和FAK的蛋白和mRNA表达与F-actin的变化一致,表明粘着斑复合物参与了TiO2纳米管诱导MSCs的成骨分化过程(图5b和6a,b) )。此外,我们还发现 RhoA(GTP 酶 Rho 家族中的一种小 GTPase 蛋白)在 Jasp 处理组中上调并被 Cyto D 抑制(图 5b 和 6a、b)。 RhoA 是 MAPK 通路中重要的上游信号转导分子,可受 FAK 调控 [25, 26]。 RhoA 的主要功能是促进应力纤维(F-肌动蛋白)的聚合和稳定性以及粘着斑复合物的组装 [27]。总之,这些数据表明TiO2纳米管可以通过粘着斑复合物和RhoA影响F-肌动蛋白聚合。
<图片>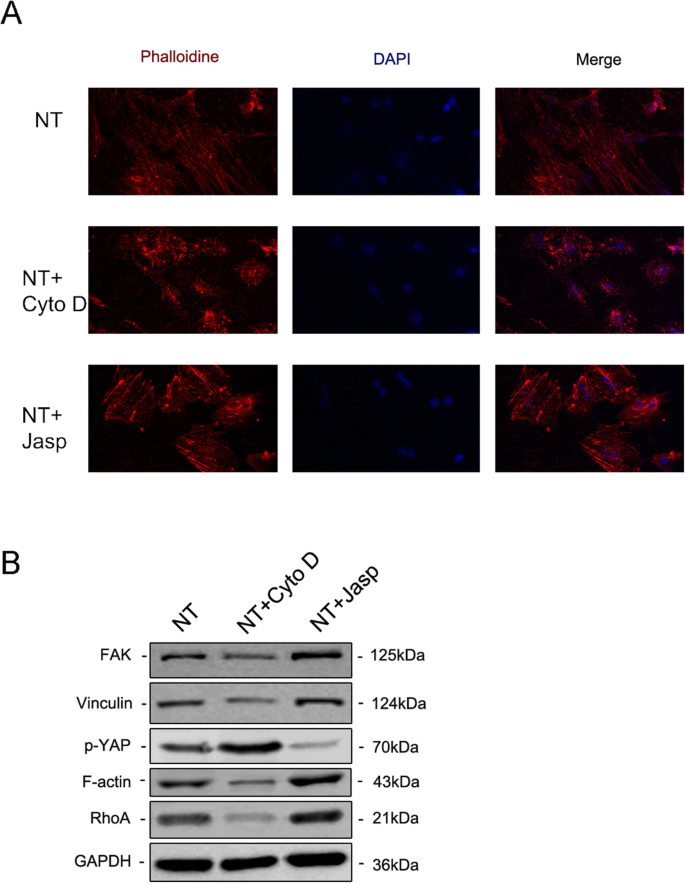
免疫荧光染色通过用罗丹明偶联的鬼笔环肽 (a )。用蛋白质印迹法研究粘着斑复合物、RhoA 和磷酸化 YAP 中 FAK 和 VCL 的蛋白表达(b )。 新台币 纳米管组
图> <图片>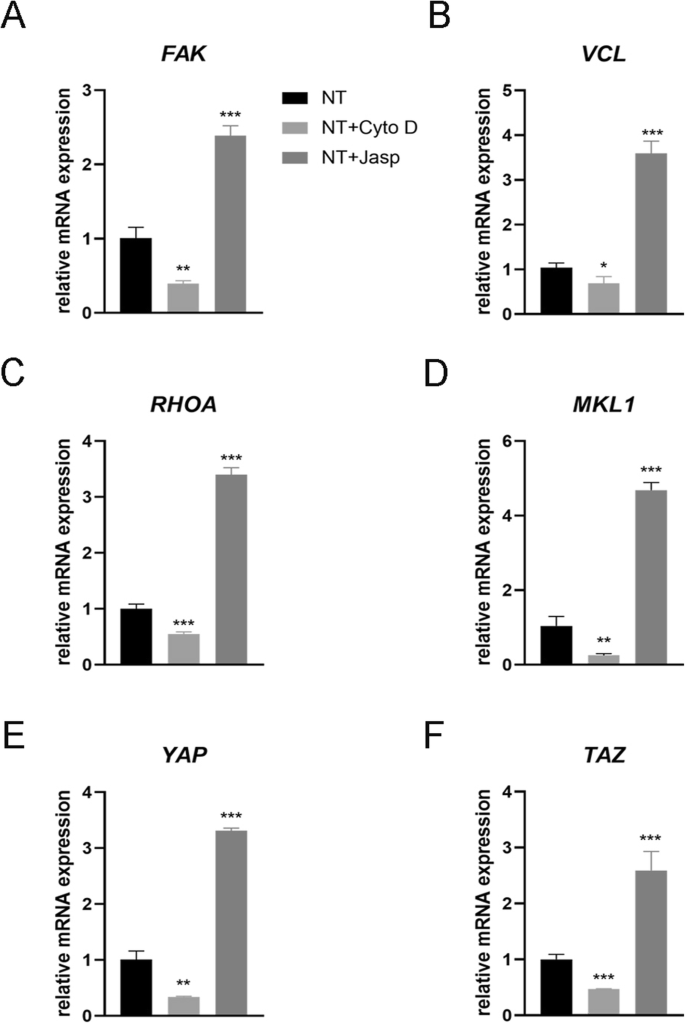
Effect of F-actin assembly on FAK (a ), vinculin (VCL) (b ), RhoA (c ), MKL1 (d ), YAP (e ), and TAZ (f ) gene expression in MSCs. NT the nanotubes group. Data represent the mean ± SD of three samples. *P <0.05, **P <0.01, and ***P <0.001
图>But how does F-actin regulate cell fate? Most studies have demonstrated that F-actin is involved in cell migration, cell division, endocytosis, and especially tumor cell invasion [28,29,30]. Few studies have suggested that F-actin could also regulate cell differentiation, let alone its specific molecular mechanism [31, 32]. Consequently, we searched for articles that mentioned the F-actin changes and found that YAP/TAZ, two closely related transcriptional co-activators in the Hippo signaling pathway, which shuttle between the cytoplasm and the nucleus, may serve as mechanotransducers in regulating MSC differentiation [33,34,35]. In addition, we also found that MKL1, a key regulator of smooth muscle cell differentiation, which interacts with the transcription factor serum response factor, could bind to G-actin and also circulate between the cytoplasm and the nucleus [21, 36]. Our results ultimately identified the involvement of YAP/TAZ and MKL1 in nanotube-induced osteoblast differentiation mediated by F-actin (Figs. 5b and 6d–f). Interestingly, the protein expression of phosphorylated YAP showed the opposite trend, indicating that not only was the expression of YAP changed, but the phosphorylation of YAP was also changed by Cyto D and Jasp (Fig. 5b). This result was consistent with the report that the phosphorylation of YAP/TAZ could be sequestrated in the cytoplasm [35].
In summary, our results preliminarily demonstrated that F-actin regulated osteoblast differentiation of MSCs on TiO2 nanotubes through MKL1 and YAP/TAZ (Fig. 7).
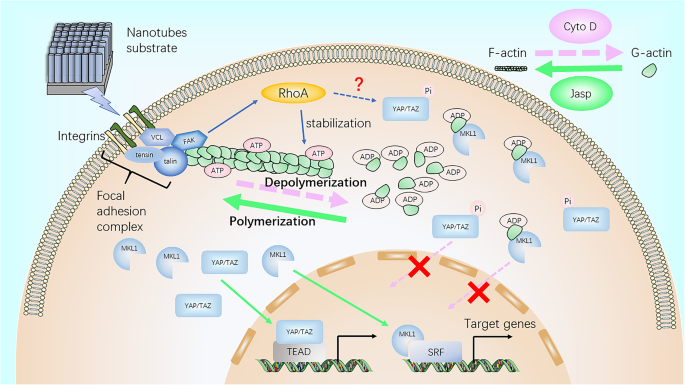
Schematic representation of F-actin assembly induced by nanotubes, and the putative role of MKL1 and YAP/TAZ in acting as the downstream mediators of F-actin signaling to regulate gene expression
图>Discussion
Titanium and titanium alloys are the most widely used metal materials in orthopedic clinical implants due to the good properties of titanium [3]. However, aseptic loosening is still an urgent problem to be solved and improved, and the key is likely to lie in improving the integration of the implant and the host bone. Previous studies have shown that surface coating and modification or immobilization of biofunctional molecules will be beneficial to osseointegration [37]. Recently, the surface topography of implants has attracted the attention of many researchers thanks to studies into the cell response to physical cues [9,10,11, 13, 38]. In this study, we demonstrated the ability of nanotubes to promote osteogenic differentiation of MSCs, and this ability was enhanced with increasing inner diameter of the nanotubes (30–70 V). This will help guide the diameter of the nanotubes on the surface of the implants.
As a topographical structure, nanotubes first change the physical properties of the material, such as adsorption capacity and electrical and thermal conductivity. These physical properties determine their application in the industrial field. For example, most high-voltage power transformers need to be filled with insulating material, which is usually transformer oil or insulating gas. When the insulation of a transformer fails due to overheating and partial discharge, a serious discharge accident will occur. Therefore, finding an effective method that accurately detects the concentration and types of dissolved gases or insulating gas decomposition components in a transformer is necessary to monitor the operating state of the transformer [8, 39,40,41]. The traditional approach is to look for materials with good gas adsorption in transition elements, which are rich in d electrons, such as Pd(1 1 1) [39]. Nowadays, nanotubes are widely studied for their good gas adsorption properties.他等人。 found that CuO-BNNT was suitable for the adsorption of C2H2, because of its stronger adsorption on C2H2 [8]. Meanwhile, TiO2 itself can be a gas-sensing material. Gui et al. found that Co-doped TiO2 further enhanced gas adsorption capacity and exhibited a superior adsorption ability and conductivity change toward C2H4 molecules [40]. Consistent with this study, Mn-doped graphene also exhibited enhanced conductivity and superior capability of C2H2 and CO detection than pristine graphene [41]. The above research indicates that the TiO2 nanotubes prepared in our experiment have a potential application in the field of monitoring the operative state of a transformer. However, the adsorption capacity and electrical conductivity of the nanotubes to gases need to be further studied, especially whether these properties are enhanced after doping with transition elements (e.g., Mn).
In addition to changing the physical properties of a surface, nanoscale morphology also affects the biological behavior of the cells attached to it. Cells first adhere to the surface of the material and then migrate, proliferate, and differentiate. Compared to a flat surface, the hollow structure of the nanotubes provides fewer adhesion sites for cells. Therefore, in order for the cells to adhere to the nanotube surface steadily and maintain the biomechanical balance within the cell, the focal adhesion complex begins to assemble and mature, and F-actin becomes strong and stable.
F-actin, a linear polymer microfilament consisting of G-actin monomers, is one of the three major components of the cytoskeleton. As a mechanical-loading structure, F-actin is generally believed to be involved in cell division, cell migration, endocytosis, and tumor cell invasion [28,29,30], but some recent studies showed that it can also affect cell differentiation [31,32,33, 36]. For example, actin cytoskeletal depolymerization by simvastatin induces chondrocyte differentiation [31], and actin depolymerization enhances adipogenic differentiation in human stromal stem cells [32]. Our results also revealed that, compared with the control group, MSCs cultured on nanotubes had higher F-actin levels and a more obvious fibrous structure. Meanwhile, promotion of F-actin polymerization by Jasp enhanced osteogenic differentiation, while the depolymerization of F-actin inhibited osteogenic differentiation, suggesting that F-actin mediates TiO2 nanotube-induced osteoblastic differentiation of MSCs.
F-actin can be regulated by Rho GTPases, members of the Ras superfamily [23, 42], and Rho can induce actin reorganization through at least two effectors, ROCK and Dia. ROCK is activated by binding to Rho-GTP and then myosin light chain (MLC), the substrate of ROCK, plays an important role in F-actin assembly. ROCK inhibits the activity of MLC phosphatase, leading to an increase in MLC phosphorylation, which stimulates the ATPase activity of myosin II and promotes the assembly of F-actin. In addition, ROCK also targets LIM kinase (LIMK). Phosphorylated LIMK inactivates cofilin by phosphorylation, which can disassemble F-actin in its active state. Another effector is Dia, a member of the formin-homology (FH) family of proteins which contains two FH domains. These domains contain multiple proline-rich motifs which bind to the G-actin-binding protein, profilin. This interaction contributes to actin polymerization and F-actin organization [42]. We detected one of the Rho GTPases, RhoA, and found that the expression of RhoA was consistent with the level of F-actin. However, we were unable to clearly describe how the nanotubes regulate the expression of RhoA, because there are many other regulators, including integrin signaling, other adhesion receptors, G protein-coupled receptors (GPCRs), soluble factors such as LPA, receptor tyrosine kinase signaling, and so on [43].
Knowing that F-actin can be regulated by RhoA, we next asked what role focal adhesion played in this process, because focal adhesion complexes, containing integrins, talin, vinculin, paxillin, and focal adhesion kinase (FAK), are formed and mature when cells attach to the surface of nanotubes. Integrins are transmembrane heterodimers that couple the ECM to the other focal adhesion proteins so as to facilitate cell attachment. They not only act simply as hooks but also transmit to the cell critical signals about the nature of its surroundings, which along with other signals such as EGFR, prompt the cell to make decisions about its biological behaviors. These signals are further transmitted to F-actin, which is directly connected to the focal adhesion complexes. On the one hand, the nanoscale morphology causes focal adhesion complex assembly and maturation. On the other hand, kinases such as FAK and Src kinase family members will recruit molecules such as CRK to self-regulate the assembly and maturation of focal adhesion complexes [44,45,46]. Our results demonstrated that the formation and maturation of focal adhesion complexes were impaired by F-actin depolymerization, suggesting that there was a feedback from focal adhesion complexes to actin assembly in line with published reports.
However, it should not be ignored that these proteins contained in focal adhesion complexes have the function of signal transduction [47]. That is to say, nanotubes may directly regulate gene expression through signal cascades, and F-actin may just participate in or be affected by this process. For instance, the dual kinase complex of FAK and Src can regulate Rho GTPases such as RhoA. This shows that nanotubes can regulate RhoA through integrins and the FAK/Src complex. In addition Src, a non-receptor tyrosine kinase protein, can activate Ras (small GTPase) by phosphorylating FAK at tyrosine residue 925 [47, 48]. Then, Ras activates numerous biochemical pathways, including the well-studied MAPK pathway and the PI3K/AKT/mTOR pathway. In the MAPK pathway, Ras activates c-Raf, followed by mitogen-activated protein kinase kinase (MAP2K) and then MAPK1/2, also known as extracellular signal-regulated kinase (ERK). ERK in turn activates transcription factors such as serum response factor (SRF) and c-Myc that are involved in regulating growth and differentiation [49]. What is more, Runx2, a key transcription factor in osteogenic differentiation, can also be regulated by ERK [50], and our previous study confirmed that mechanical strain promoted osteogenic differentiation of BMSCs through the FAK-Erk1/2-Runx2 pathway [17]. Therefore, we cannot rule out that ERK plays a role in nanotube-induced osteogenic differentiation and further study is still needed.
So what exactly is the role of F-actin in inducing differentiation of nanotubes, because its change can affect cell differentiation? One possibility is that the change of F-actin assembly can inversely regulate the level of FAK so as to induce osteogenic differentiation through the FAK-Erk1/2-Runx2 pathway as described above, because in our results, focal adhesion complexes and actin polymerization showed the same trends of change, indicating that they act as a whole in response to the extracellular environment. However, some other possibilities also exist, and a number of articles have shown that MKL1 and YAP/TAZ act downstream of the actin dynamic balance [20, 51,52,53,54]. Both of them shuttle between the cytoplasm and the nucleus, and may help to transduce signals from the cytoskeleton to the nucleus.
MKL1, also termed myocardin-related transcription factor A, is sensitive to changes in G-actin levels. When cytoplasmic G-actin levels increase, monomeric G-actin binds to MKL1 and prevents it from binding to SRF and activating transcription. SRF target genes include actins such as smooth muscle actin (SMA) as well as other actin-binding proteins, including immediate early genes like c-fos and egr1. Recent studies have demonstrated that changing SRF activity could regulate adipogenesis by activating the adipogenesis transcription factor peroxisome proliferator-activated receptor γ (PPARγ), and also regulate bone formation via IGF-1 and Runx2 signaling [55, 56].
YAP and TAZ are two transcriptional coactivators in the Hippo signaling pathway, identified as an important regulatory pathway that restricts cell proliferation, thereby controlling organ size and morphogenesis [20]. Large tumor suppressor genes 1 and 2 (LATS1/2) phosphorylate them, thereby creating a binding site for 14-3-3 proteins, the binding of which prevents their nuclear import [53, 54]. As a consequence, phosphorylated forms of YAP/TAZ are sequestered in the cytoplasm, preventing the expression of genes like Ctgf and Areg. In addition, some studies have shown that YAP/TAZ can interact with T-box 5 (TBX5), RUNX2, and p73 to regulate gene expression [57,58,59]. Further, cell adhesion to cell matrix proteins has been shown to trigger YAP nuclear localization through an integrin/FAK/Src axis. In our study, the results suggested that this pathway was possibly involved in nanotube-induced differentiation. Further study into the downstream mediators of the integrin/FAK/Src axis should be carried out to clarify the specific mechanism.
On the other hand, more and more studies illustrate that F-actin interacts with Hippo signaling, and somehow inhibits the phosphorylation of YAP [54, 60], which is consistent with our experimental results that promoting F-actin polymerization reduces the expression of phosphorylated YAP. We hypothesize that ATP involved in the process of the transformation between G-actin and F-actin may also play an important role in the phosphorylation of YAP, which is yet to be studied.
After understanding the above possible molecular mechanisms, we can try to explain some of the experimental phenomena found in this study. Our results revealed that the larger the diameter of the nanotubes, the stronger the ability of the nanotubes to promote osteogenic differentiation. This is consistent with previous research [61, 62]. The reason for this phenomenon is that the larger the diameter of the nanotubes, the less adhesion sites they can provide to the cells, and the greater the assembly and maturity of focal adhesion complexes. Along with these, stress fibers made of F-actin will have greater strength and stability. These structures enhance the signaling that promotes osteogenic differentiation. Predictably, however, this effect is significantly reduced when the nanotubes become too large in diameter, making it difficult for the cells to adhere to the surface [12]. Similarly, when the height of the nanotubes is inconsistent, the differences in height can result in a change of adhesion site and rearrangement of the cytoskeleton, which will further affect cell differentiation. Intriguingly, even flat surface materials without nanotube modification can induce changes in cell differentiation. A number of studies have demonstrated that focal adhesion formation and stress fiber organization are regulated by substrate stiffness [63,64,65], and YAP/TAZ also plays an important role in this process. Therefore, it is obvious that the integrins–FAs (focal adhesions)–F-actin axis plays a role in the transduction of physical signals into intracellular chemical signals.
In summary, our results demonstrated that F-actin regulates osteoblastic differentiation of mesenchymal stem cells on TiO2 nanotubes through MKL1 and YAP/TAZ, whose target genes partly explained the proliferation and differentiation of MSCs. We know that there is no single change in the signal network and any change is regulated by numerous molecules and proteins. One type of biological behavior must be the result of the regulation of a series of signaling pathways. Nanotubes induce cell differentiation by triggering a complex network of signals, including integrins, proteins contained in focal adhesion complexes, FAK, Src, Rho GTPase, the MAPK pathway, the Hippo pathway, and other reported signaling pathways. At least as important, there are many signal cycles in the signal network and a downstream signal can regulate the upstream signal via feedback. In this study, we found that vinculin and FAK can be regulated backwards by F-actin assembly, increasing the uncertainty of molecular function. Therefore, more details of the molecular mechanism await further study.
结论
Our results showed that TiO2 nanotubes promoted the osteogenic differentiation of MSCs, and this ability was enhanced with the increasing diameter of nanotubes within a certain range (30–70 V). F-actin mediated nanotube-induced cell differentiation through MKL1 and YAP/TAZ, providing a novel insight into the study of cell differentiation.
数据和材料的可用性
本研究中使用和分析的数据集可向相应作者索取合理要求。
缩写
- MSCs:
-
Mesenchymal stem cells
- SEM:
-
扫描电镜
- EDS:
-
X-ray energy dispersive analysis
- 原子力显微镜:
-
原子力显微镜
- ALP:
-
Alkaline phosphatase
- Cyto D:
-
Cytochalasin D
- Jasp:
-
Jasplakinolide
- VCL:
-
Vinculin
- FAK:
-
Focal adhesion kinase
- BCA:
-
Bicinchoninic acid
- PVDF:
-
聚偏二氟乙烯
- Runx2:
-
Runt-related transcription factor 2
- Osx:
-
Osterix
- OCN:
-
Osteocalcin
- YAP:
-
Yes-associated protein
- MKL1:
-
Megakaryoblastic leukemia 1
- FBS:
-
胎牛血清
- ECM:
-
Extracellular matrix
- MLC:
-
Myosin light chain
- LIMK:
-
LIM kinase
- FH:
-
Formin-homology
- GPCR:
-
G protein-coupled receptors
- MAP2K:
-
Mitogen-activated protein kinase kinase
- ERK:
-
Extracellular signal-regulated kinase
- SRF:
-
Serum response factor
- SMA:
-
Smooth muscle actin
- PPARγ:
-
Peroxisome proliferator-activated receptor γ
- LATS1/2:
-
Large tumor suppressor gene 1 and 2
- TBX5:
-
T-box 5
纳米材料
- 二氧化钛 - TiO2 - 价格、市场和分析
- 走向 TiO2 纳米流体——第 1 部分:制备和性质
- 纳米技术灭活癌症干细胞
- 迈向 TiO2 纳米流体——第 2 部分:应用和挑战
- TiO2 中金纳米粒子分布对染料敏化太阳能电池光学和电学特性的影响
- ZnO 纳米晶体的合成及其在倒置聚合物太阳能电池中的应用
- 制备钙钛矿太阳能电池二氧化钛致密层的最佳钛前驱体
- 弹性刚度和表面附着力对纳米粒子弹跳的影响
- 嵌入TiO2致密层的不同尺寸和浓度的Ag纳米颗粒对钙钛矿太阳能电池转换效率的影响
- 一种新型 Ho3+-Yb3+-Mg2+ 三掺杂 TiO2 上转换材料及其在钙钛矿太阳能电池中的应用
- 碳纳米管及其衍生物对体外肿瘤细胞和生化参数、体内细胞血液成分的影响
- 基于原子力显微镜的软骨分化人类脂肪干细胞纳米显微镜:纳米结构和整合素 β1 表达