一维混合二元氧化物 CeO2-LaO x 支持的金催化剂的合成和 CO 氧化活性
摘要
通过水热法合成了具有不同 La 含量(Ce 和 La 的摩尔比为 1:0、3:1、1:1、1:3 和 0:1)的一维 (1D) Ce-La 纳米棒。 Au/Ce-La 纳米棒催化剂是通过改进的沉积-沉淀法获得的。通过N2吸附-解吸(BET)、ICP、X射线衍射(XRD)、SEM、TEM、EDX、X射线光电子能谱(XPS)、UV-vis漫反射光谱(UV-vis DRS)对样品进行表征, 和程序升温还原 (H2-TPR)。它揭示了 La 以 LaO x 的形式存在 在一维纳米棒中。催化结果表明,混合二元 Ce-La 纳米棒氧化物可以作为金催化剂的良好载体。 La的含量对Au/Ce-La纳米棒催化剂的催化性能有重要影响。在催化剂中,当 Ce/La 摩尔比为 3:1 时,300°C 预处理的 1.0%Au/Ce0.75-La0.25 纳米棒在催化剂中表现出最佳的 CO 氧化活性,可以完全转化 CO在 30°C。该催化剂在40、70和200°C的反应温度下也表现出耐高温和良好的CO氧化稳定性。
背景
作为一种非常有害的气体,CO 可以与血液中的血红蛋白中的铁原子强烈结合,从而阻止氧气的释放。因此,它在室内的存在甚至可以在短时间内造成人类和动物的死亡。它已成为日益严重的空气污染问题。催化 CO 氧化已成为解决此类严重环境问题的最有效的 CO 去除解决方案之一 [1,2,3,4,5,6,7,8]。最近,在汽车尾气净化、室内空气净化和低温 CO 传感器等污染控制装置领域也受到科学界的广泛关注 [6,7,8,9,10]。在许多情况下,分散在具有高储氧能力的特定金属氧化物(如 CeO2、TiO2 和 Fe2O3)上的珍贵 Au 是 CO 氧化的高效候选者 [11,12,13]。在过去的几十年中,对低温 CO 氧化的负载型金催化剂的研究产生了意想不到的观察结果。人们普遍认为,Au 催化剂的催化活性在很大程度上取决于 Au 纳米粒子的性质和载体的性质,例如金粒径、Au 金属-载体相互作用和载体的还原性 [14,15,16 ,17,18]。
作为最重要的稀土氧化物之一,CeO2 因其独特的物理和化学性质而被广泛应用于三元催化剂中作为有效的催化剂载体 [6, 8, 15, 17]。 CeO2由于能够转换Ce 4+ 而具有优异的氧储存和释放能力 /Ce 3+ ,这使得 CeO2 成为各种氧化还原催化反应中使用的各种氧化催化剂的活性氧化物组分 [17,18,19,20,21,22,23,24,25,26,27,28,29,30,31, 32]。表面积、介孔结构、晶格缺陷以及与其他掺杂剂的协同效应都可以促进氧化铈纳米材料的催化性能 [3, 22]。为了进一步提高Au-CeO2催化剂在CO氧化反应中的性能,人们尝试了许多策略,例如沉积-沉淀、共沉淀和尿素-凝胶共沉淀等制备方法,用于控制和优化催化剂的相互作用。 Au-O-Ce 结构,以及氧化铈的大小和形状 [33,34,35]。还尝试通过载体的表面改性 [4, 5, 22, 24, 26, 36,37,38]。已经发现,使用二元混合氧化物作为载体可以为金纳米颗粒的稳定提供良好的解决方案。此外,贵金属或过渡金属的促进增强了氧化铈的还原性并促进了表面氧空位的形成。同时,掺杂过渡金属阳离子已被证明是提高一维 (1D) 纳米结构纳米材料的理化性质(如催化活性)的有效方法 [38,39,40]。王等人。 [5] 用高度分散的 CoO x 修饰 Au/CeO2 的表面 并在低温 CO 氧化中表现出优异的催化活性。马等人。 [37] 报道说,CaO、NiO、ZnO、Ga2O3、Y2O3、ZrO2 和稀土添加剂添加到金-二氧化钛催化剂中有利于 CO 氧化,并且掺杂的催化剂在 500°C 老化后在环境温度下可以显示出显着的活性。帕克等人。 [38] 报道称,CeO x 改性TiO2载体是水煤气变换反应的良好催化剂。已经有很多关于混合金属氧化物用于 CO 催化氧化的研究。这些掺杂的金属离子要么以氧化物颗粒的形式沉积在载体表面,要么沉积在载体的晶格中,不能形成单独的氧化物相。本研究的目标是制备一维二元 Ce-La 纳米棒,它是非钙钛矿或固溶体类型的混合氧化物。也就是说,在一维纳米棒结构中,两种金属氧化物共存,结合两种成分的优点,使协同效应最大化。由于潜在的技术应用,包括纳米棒、纳米线和纳米管在内的许多一维纳米材料在过去几年中得到了广泛的研究 [2, 4, 41, 42]。这些一维纳米结构材料,尤其是一维纳米棒材料,已被研究作为催化、光学和电化学领域的重要载体或活性成分,例如用于太阳能电池的可控硅纳米线[42]。已经发现,一维结构材料的催化活性等性质往往与其晶体结构和形状密切相关。因此,开发一维纳米棒材料以定制其电子和催化性能被证明是有趣和有价值的。
在此,我们报告了一种简单的溶剂热策略来制备一系列混合的 Ce-La 纳米棒复合材料。在合成过程中,LaO x 和 CeO2 可以在一根棒中一起生长。最终产品的形态不受影响。 XRD和TEM结果表明La阳离子以LaOx的形式存在 .发现 LaOx 的掺杂 对金-氧化铈催化剂的活性显示出积极的影响。 Au/Ce0.25-La0.75纳米棒对CO氧化表现出优异的催化活性。
实验
本文中所有化学品均为分析纯,未经任何纯化直接使用。
支持准备
Ce-La纳米棒采用常规水热法合成。在典型的合成中,将 NaOH (9 mol/L) 和 Ln(NO3)3 (Ln =Ce, La, 0.8 mol/L) 溶液混合并在室温下剧烈搅拌 30 分钟。将所得悬浮液倒入衬有聚四氟乙烯的不锈钢高压釜中。将高压釜密封并在 110°C 下保持 14 小时,然后风冷至室温。将所得产物过滤,用去离子水和无水酒精洗涤,在 80°C 下干燥 12 小时,然后在 400°C 空气中以 5°C min -1 的加热速率煅烧 在支持金纳米粒子之前。具有不同 La 含量的最终产品(Ce 和 La 的摩尔比为 1:0、3:1、1:1、1:3 和 0:1)表示为 Ce 纳米棒,Ce0.75-La0.25纳米棒、Ce0.50-La0.50纳米棒、Ce0.25-La0.75纳米棒、La纳米棒。
催化剂制备
进行沉积-沉淀过程以制备Au/Ce-La纳米棒催化剂。简而言之,将所需量的 Ce-La 纳米棒分散在 100 mL 去离子水中,然后与一定量的 0.01 mol/L HAuCl4 溶液混合。由于最终的 HAuCl4 溶液的 pH 值约为 7,这与载体的碱性和 HAuCl4 的酸度有关,因此溶液的 pH 值不会被调整。悬浮液保持搅拌 12 小时并在 100°C 下回流 4 小时。在沉积-沉淀程序之后,将沉淀物离心,用水洗涤以除去Cl - 离子,并在 80°C 下在空气中干燥 12 小时。金的浓度以质量百分比表示。
表征技术
Au/Ce-La 纳米棒催化剂的金负载量通过电感耦合等离子体原子发射光谱法(ICP-9000,美国 Thermo Jarrell-Ash 公司)测定。使用 Micromeritics Tristar II 3020 装置在 - 196°C 下通过氮吸附测量 Ce-La 纳米棒样品的布鲁瑙尔-埃米特-特勒 (BET) 表面积。 XRD 研究在 Rigaku D/Max-2500 X 射线衍射仪 (Kα λ =0.154 nm) 在 2θ 3–80° 的范围。在紫外-可见近红外分光光度计(JASCO Corp V-570)上收集催化剂的紫外-可见DRS。 TEM 观察和能量色散 X 射线分析 (EDX) 是用 JEM-2100 透射电子显微镜在 200 kV 下操作获得的。 SEM 数据和元素映射图像是使用在 15 kV 下运行的 JSM-7500F 扫描电子显微镜获得的。在使用单色 Al Kα 的 Kratos Axis Ultra DLD X 射线光电子能谱仪上记录 XPS 以鉴定催化剂的化学成分和氧化态 源在 150 W 下运行。结合能使用 C 1s 校准 峰值位于 284.6 eV。在 PX200 设备上进行程序升温还原 (H2-TPR) 以测量 H2 消耗量。在 H2-TPR 分析之前,样品在 He 流中在 300°C 下预处理 1 小时。冷却至 50°C 后,通过以 10°C/min 的速率加热至 900°C,用 10 vol% H2/Ar 气流还原催化剂。
催化活性测试
催化活性评估在内径为 8 毫米的固定床流动毫反应器中进行。在反应之前,将 200 毫克催化剂用 17.6 克化学惰性石英砂稀释。随后,将与空气平衡的 10% CO 混合物以 36.3 mL min -1 的总流速引入反应器 .在反应温度下保持 30 分钟后,气态产物通过 COx 在线分析 分析仪(GC-508A 气相色谱仪)。 CO转化率根据下式计算:
$$ \mathrm{CO}\kern0.5em \mathrm{conversion}=\frac{\left[\mathrm{CO}\ 2\right]}{\left[\mathrm{CO}\right]+\left[ \mathrm{CO}\ 2\right]}\times 100\% $$其中 [CO] 和 [CO2] 分别代表出口 CO 和 CO2 浓度。样品催化活性的温度依赖性记录在 30-200°C 的范围内,升温速率为 10°C min -1 .
结果与讨论
Au/Ce-La 纳米棒催化剂的表征
ICP
Au/Ce-La 纳米棒催化剂中存在的金量由 ICP-AES 确定。表 1 中显示的结果表明,所有催化剂中的实际金含量都低于标称值。根据制备程序,金在沉积-沉淀过程中应有损失。
图>下注
N2 吸收测量用于测量CeO2 纳米棒和Ce-La 纳米复合材料的BET 表面积和平均直径。如图 1 所示,Ce-La 纳米棒的吸附等温线为 IV 型,表现出 H3 磁滞回线的特征。所有样品在相对压力大于 0.85 时都表现出非常强烈的 N2 吸附体积增加,这是存在大量介孔 [2, 22] 的特征,表明 Ce-La 纳米复合材料由聚集体(松散组合) 形成狭缝状孔隙。随着 La 的掺杂,磁滞回线移动到约 0.95 的相对压力,这意味着孔隙尺寸会变小,对应于 Ce-La 复合材料的减少。如表 2 所示,CeO2 纳米棒的比表面积为 99.7 m 2 /g,减少到 74.1 m 2 /g 当以 3:1 的 Ce/La 摩尔比掺杂 La 时。随着La含量的增加,Ce-La纳米复合材料的表面积不断减小。这主要是由于 La 的含量,它不会嵌入 CeO2 的晶格中,而是作为孤立的 LaO x 存在 导致 Ce-La 纳米复合材料的形态差异很小。可以观察到,所有纳米棒的表面积都相似,为 80–100 m 2 /G。 Ce0.75-La0.25 纳米棒的孔体积为 0.23 cm 3 /g,与 Ce 纳米棒相似,但比其他 Ce-La 纳米棒大。来自 BJH 分析的估计孔径证实了 Ce-La 纳米复合材料的介孔性质。这可能是催化CO氧化的优势。
<图片>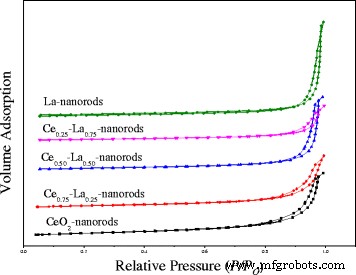
不同La含量Ce-La纳米棒的氮吸附-解吸等温线
图> 图>XRD
对合成的样品进行粉末 X 射线衍射分析,随后分析了它们的结构属性。在 2θ =28.6°、33.1°、47.6° 和 56.3° 处观察到氧化铈的结晶峰(图 2a),对应于(111)、(200)、(220)和(311)衍射平面和证实了 CeO2 晶体的立方萤石结构(JCPDS 编号 34-0394)。当 La 含量为 0.25 at.% 时,La-Ce 复合材料的衍射峰变宽。以 2θ =30.0°、46.0°、52.0° 和 53.6° 为中心的峰对应于孤立的 La2O3 的衍射平面。没有检测到指定给 La(OH)3 的峰。但由于含量低且衍射位置近似,不易鉴别LaOx的存在 .随着 La 含量的增加,纳米复合材料中的 La2O3 或 La(OH)3 出现了一些突出的峰。 La2O3 的主要衍射峰位于 2θ =30.0° (101)、39.6° (220)、46.2° (110) 和 66.8° (112),可归为六方相(JCPDS 卡 05-0602 )。 La(OH)3 的主要衍射峰出现在 2θ =15.7° (100)、27.3° (110)、27.9° (101) 和 39.4° (201),可以归为六方相 (JCPDS)卡 36-1481)。结果表明,La 可以作为孤立的 La2O3 或 La(OH)3 存在于复合材料中。金沉积后,没有衍射峰可以指示金的纯面心晶体结构(图 2b)。这可能是由于金纳米粒子含量低和/或粒径小所致。
<图片>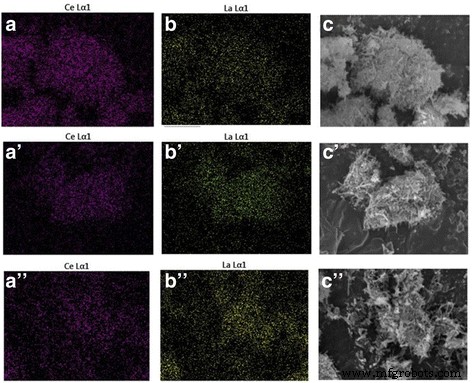
具有不同 La 含量 (0–100 at.%) 的 1% Au/Ce-La 纳米棒的 XRD 图谱 (a ) 和具有不同 Au 负载量的 Au/Ce0.75-La0.25 纳米棒在 300°C 下煅烧 2 小时 (b )
图>SEM 和 TEM
图 3a-e 为不同浓度 La 3+ 得到的 CeO2 和 Ce-La 纳米复合材料的 SEM 照片 离子。可以看出,所有的 Ce-La 纳米复合材料都表现出棒状结构。显然,许多棒堆叠成 Ce-La 束,导致形成不同大小的狭缝状孔。结果与 N2 吸附-解吸等温线一致。如图 2a 所示,产品主要由直径为 5-10 nm、长度为 100-300 nm 的纳米棒组成。在图 3e 中,可以清楚地看到大量直径约为 12.5 nm 的纳米棒,还有少量平均直径约为 8.0 nm 的短纳米棒。在图 3b-d 中,随着 La 3+ 掺杂浓度的增加 ,样品总是呈现纳米棒形态。然而,虽然掺杂浓度为 25 mol%,但所获得的样品在所有样品中显示出最均匀的纳米棒,直径为 5-20 nm,长度为 100-300 nm。图 3f 显示了获得的单个 Ce-La 纳米棒的 TEM 图像。从氮吸附-解吸等温线可以看出,载体中有许多孔隙。 Ce-La 纳米棒的 HRTEM 图像显示它们在结构上是均匀的,并且本质上是单晶的。图 3f 中的晶格条纹插图说明了两个面间距值,即 0.31 和 0.34 nm,它们分别与 CeO2 和 La2O3 的(111)、(110)面一致 [3, 15, 43]。它揭示了 La 3+ 离子已有效地生成为 La2O3,这与 XRD 谱一致。
<图片>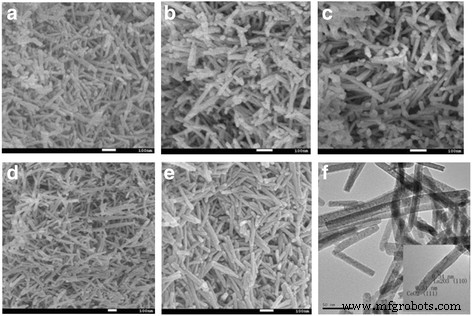
不同La含量的Ce-La纳米棒的SEM图像:0 (a ), 25 at.% (b ), 50 at.% (c ), 75 at.% (d ), 100 at.% (e ); Ce0.50-La0.50 纳米棒的 TEM 图像 (f );插图显示了相应的 HRTEM 图像
图>采用元素映射和 EDS 分析来确定 Ce-La 样品的化学成分(图 4 和表 3)。结果显示均匀的 La/Ce 摩尔比与合成的预期值非常一致。在 300°C(图 5a)和 400°C(图 5c)下煅烧的 Au/Ce0.75-La0.25 样品的 TEM 图像清楚地表明,添加金后 Ce-La 纳米晶体的形状基本不变.通过 TEM 在 Ce-La 纳米棒上没有观察到金颗粒。高度分散的金簇的存在 (d <1 nm) 已通过元素映射和 EDX 分析得到证实(图 5b、e 和 f 中的插图)。一致地,对该样品进行的 XRD 分析(图 2)没有显示任何与金相关的峰,因为金颗粒太小而无法检测到。这表明 Ce-La 纳米棒表面可以将金原子分散和稳定为亚纳米簇(TEM 不可见)。这与文献 [2, 44,45,46] 是一致的。然而,一些大的金颗粒团块(平均 d ~ 7 nm)已在 400°C 煅烧的 Au/Ce0.75-La0.25 纳米棒中观察到,因为间距为 0.236 nm 的条纹被分配到金属 Au 的 (1 1 1) 平面(图 5c)其中显示了金颗粒的团聚体)。可以看出,随着煅烧温度的升高,金颗粒明显长大,相应地导致催化活性下降。
<图片>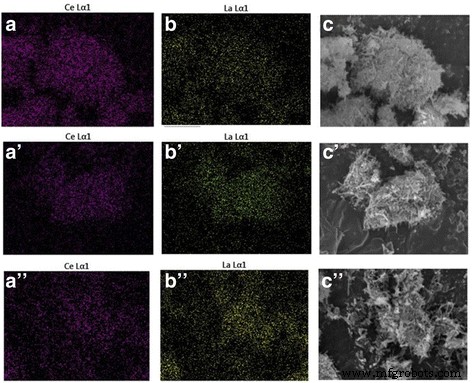
Ce和La的元素映射图像,La含量为25原子%的Ce-La纳米棒混合样品的SEM图像(a –c ), 50 at.% (a ′–c ′), 75 at.% (a ′′–c ″)
图> 图> <图片>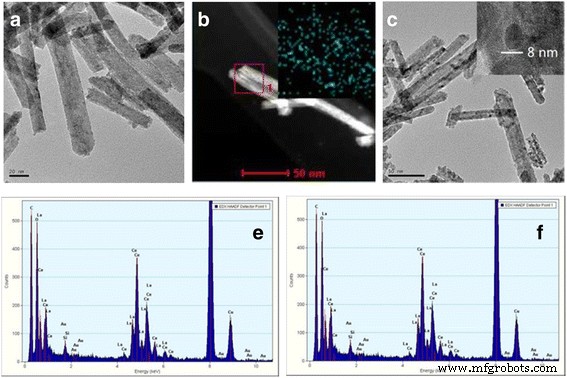
在 300°C 下煅烧的 0.5% Au/Ce0.75-La0.25 纳米棒的 TEM 和 STEM 图像 (a –b ) 和 400°C (c ), EDX 分析 (e –f ) (a 的图像 –b ) 表示存在金信号
图>XPS
执行图 6 中的 XPS 光谱以研究在 300°C 下煅烧 2 小时的 1%Au/Ce0.75-La0.25 纳米棒样品中的化学复合物和状态。 Ce 3d的XPS光谱 显示了 3d 的明显峰值 3/2 自旋轨道态和 3d 图 6a 中的 5/2 自旋轨道状态。众所周知,这些峰位于结合能约为 899、903 和 916 eV 处,通常用作光谱标记来检测 Ce 4+ 状态。在我们的例子中,Ce 3d 核心能级显示三个自旋轨道双峰,它们是 Ce 4+ 四价态的特征峰 .位于 882.8、888.1 和 898.4 eV 附近的峰归属于 Ce 3d 5/2,以及那些在 901.3、907.0 和 916.7 eV 附近的被分配给 Ce 3d 3/2,对应于 Ce (IV) 化合物的自旋轨道分裂双峰。观察结果与报道的文献大体相符 [19, 28, 29, 32]。很明显,样品处于Ce 4+ 状态 不含任何Ce 3+ 杂质 状态。图 6b 显示了 La 3d 的 XPS 光谱 1%Au/Ce0.75-La0.25 纳米棒样品的区域。自旋轨道分裂 3d 5/2 和 3d 3/2 水平显示双峰结构。 3d 之间的自旋轨道分裂 3/2 和 3d 5/2 能级约为 17.0 eV,卫星峰与主峰之间的间隔为 4.1 eV,与 La 3+ 的报告值一致 化合物 [11, 47]。正如预期的那样,La 以 + 3 氧化态存在,可能对催化活性有重要影响。 O 1s XPS 光谱(图 6c)是不对称的,分别解卷积为 529.3、531.6 和 527.6 eV。 529.3 eV 处的峰归属于晶格氧,531.9 eV 附近的峰归属于载体表面的羟基 [27, 28, 32]。 527.6 eV 的小肩峰归因于 La-O,这也可以揭示 LaO x 的存在 在催化剂中 [11, 48]。很明显,根据高峰强度,载体表面存在大量羟基。 Au 4f 中的 XPS 光谱 在 300 和 400°C 下煅烧的催化剂区域如图 6d 所示。在图 6d 中,在 300°C 下煅烧的催化剂显示出 Au 4f 7/2 结合能信号为 84.6 eV。信号是阳离子 Au + 的特征 物种 [14, 15, 31]。相比之下,催化剂在 400°C 下煅烧后,Au 4f 7/2 峰位于结合能 83.6 eV,Au 4f 5/2 位于结合能 87.7 eV。金属Au 0 的存在 被清楚地观察到。还检测到位于 85.0 和 88.2 eV 附近的小峰,对应于氧化的金物质。很明显,在 300°C 下煅烧的催化剂实际上主要显示出阳离子 Au + 种(> 90% Au + 物种)。相比之下,在 400 °C 下煅烧的样品具有 90% 的 Au 0 和 10% 的 Au δ+ .从金属 Au 到载体的电子密度转移导致 Au 的部分氧化和金和载体之间的强相互作用。 Au δ+ 的存在 负责支撑表面的部分减少。因此,Au δ+ 被认为比 Au 0 更活跃 用于 CO 氧化 [11, 21]。在我们的案例中,在 300 °C 下煅烧的催化剂具有更多的 Au δ+ 比在 400°C 煅烧的催化剂的活性高,因此不难推断出在 300°C 煅烧的催化剂比在 400°C 煅烧的催化剂活性更高,这与活性结果一致。
<图片>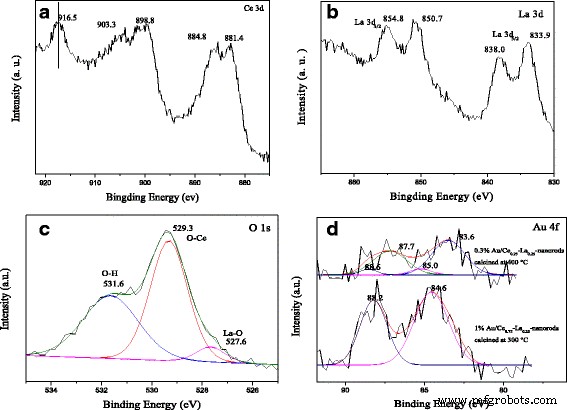
在 300°C 下煅烧 2 小时的 1%Au/Ce0.75-La0.25 纳米棒的 XPS 光谱:Ce 3d 峰值 (a ), La 3d 峰值 (b ), 和 O 1s 峰值 (c )。 Au 4f 峰值 (d ) 的 Au/Ce0.75-La0.25 纳米棒在 300 和 400°C 下煅烧 2 小时
图>紫外可见
在不同温度下煅烧的 Ce0.75-La0.25 纳米棒和 0.5% Au/Ce0.75-La0.25 纳米棒的 UV-vis 漫反射光谱如图 7 所示。在载体的光谱中,在不同温度下煅烧的催化剂的光谱在 500 和 600 nm 之间表现出弱而宽的吸收带,这是金属金纳米粒子的表面等离子体共振 (SPR) 的特征 [21, 24, 49]。 SPR 可以归因于电子响应于光激发的集体振荡,这将导致在紫外可见区域吸收光。表面等离子体共振的位置受分散的金颗粒尺寸、颗粒形状和周围材料的介电特性的影响。在本研究中,煅烧预处理导致吸收带发生较大的红移,随着煅烧温度的升高,吸收带的位置(500-600 nm)发生红移。变化等级如下:80°C→200°C→300°C。随着煅烧温度进一步升高至 400°C,吸收带向短波长移动。关于移动峰位置的解释有几篇报道 [24, 50,51,52,53]。虽然金颗粒的直径 <2 nm,但扩大的位移峰位置主要是由金属介电函数的尺寸相关阻尼引起的。由于与周围金属氧化物的化学相互作用,金颗粒中的电子密度也降低,这可以解释导致进一步红移的机制[52]。金颗粒尺寸的增加会导致吸收峰蓝移(平均直径小于 25 nm),对于大颗粒(平均直径大于 25 nm),观察到相反的效果 [53]。根据 TEM 数据,对于在 300°C 下煅烧的催化剂,催化剂中金颗粒的尺寸 <1 nm。然而,随着煅烧温度进一步升高至 400°C,金颗粒开始生长,平均尺寸约为 7 nm。如前所述,等离子体带的位置在很大程度上取决于金颗粒的形状和大小。在目前的情况下,这种大的变化可能是由于金颗粒大小的不同而造成的。数据与催化活性试验结果一致。这也表明金纳米粒子在载体表面分散良好。
<图片>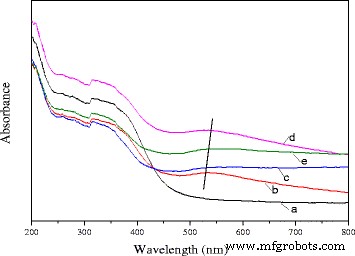
在 80°C (b)、200°C (c)、300°C 下煅烧的纯 Ce0.75-La0.25 纳米棒载体 (a) 和 0.3% Au/Ce0.75-La0.25 纳米棒的 UV-vis DRS (d) 和 400°C (e)
图>H2-TPR
图 8a 显示了纯氧化物和混合氧化物样品的 TPR 曲线。对于纯 CeO2 纳米棒,以低温 (410°C) 和高温 (620°C) 为中心的还原峰分别归因于 CeO2 的表面和本体氧物种的还原 [1, 32]。对于纯 La 纳米棒,可以在 ~ 700°C 处检测到明显的还原峰,这归因于本体 La2O3 的还原。有趣的是,Ce-La 纳米棒在~ 500°C 处出现了还原峰。具有 25%、50% 和 75% at.% La 掺杂的三个样品的还原峰显示在 La 掺杂后向更高温度移动约 20°C。当 La 含量为 25 原子%时,观察到 520°C 的强烈还原峰温度。这是一个新的还原温度,与纯 CeO2 纳米棒的还原温度显着相关。与参考文献相比,由于 La-O 和 Ce-O 之间的协同相互作用,Ce-La 纳米棒的还原温度高于纯 CeO2 [31, 54]。可以发现二元氧化物应该有独立的CeO2和LaOx .如图 8b 所示,金沉积后,Au/CeO2 和 Au/La-Ce 纳米棒在极低温度(100-200°C)下出现新的还原峰。这里,由于 XPS 结果,催化剂在 300°C 下煅烧后,Au 主要是 Au δ+ ,因此在 ~ 200°C 处的还原峰归因于高价金物质的还原 [21]。以~ 350°C 为中心的小峰可能与 Ce-La 纳米棒的还原有关。此外,对于 1% Au/Ce0.75-La0.25 纳米棒,230°C 附近的另一个还原峰可归因于金促进 CeO2 的还原。百分之一的 Au/Ce0.75-La0.25 纳米棒在催化剂中具有最低的还原温度,这可能有助于它成为最活跃的 CO 氧化催化剂。这与活动结果一致。由于所有氧化物载体的表面还原峰在金沉积后显着降低,这表明大多数可用氧在此较低温度下减少,并表明金上的 H2 离解和溢出到相邻氧化物表面上更有可能是原因为强烈的低温还原峰[31]。 TEM 和 XPS 数据表明小尺寸的阳离子金颗粒高度分散在载体表面。 LaO x 的存在 还可以帮助稳定阳离子 Au。这有利于金-载体相互作用的强度[11]。金和载体之间的强相互作用促进了 Au/Ce-La 纳米棒向低温转移的还原。结果表明,金沉积对Ce-La纳米棒的还原性影响很大。
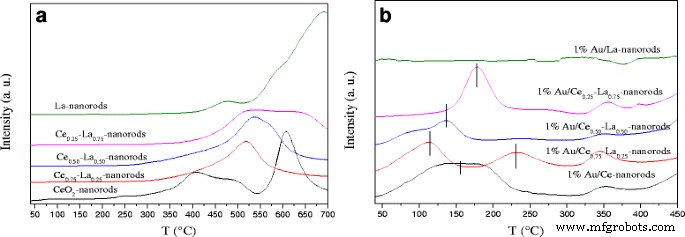
H2-TPR profiles of a La-Ce nanorods with different La content (0–100 at.%) and b 1% Au/La-Ce nanorods with different La content calcined at 300 °C
图>Catalytic Activity
Effect of La Content
As shown in Fig. 9, catalytic activity results for Au/Ce-La nanorod samples ranging from pure CeO2 to 100 at.% La-content nanorod supports. The most striking feature in the figure is the high activity of the Au/Ce0.75-La0.25 nanorod catalyst with the 100% conversion at temperature as low as 30 °C. In contrast, the other Au/La-Ce catalysts showed lower activity compared to Au/Ce0.75-La0.25 nanorods catalysts under the same reaction conditions. The results indicated that La doping has very much impact on this high CO conversion activity with a La content of 25 at.%, while a further increase results in a significant drop in activity. This again closely mirrors the trends seen in the reducibility of the samples, where an increase of La content from 25 at.% results in a strong loss of reducibility.
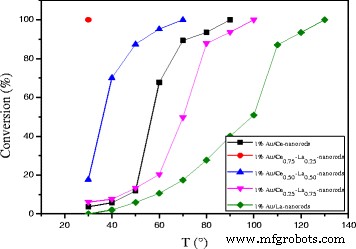
Catalytic activities of 1% Au/ La-Ce nanorods with different La content calcined at 300 °C for 2 h
图>In consideration of the preparation methods, gold loadings, gold particle size and distribution on different Ce-La nanorods supports, XRD, TEM and XPS data showed that all the catalysts should have the same number and type of active Au sites. So this high activity of the Au/Ce0.75-La0.25 nanorods catalysts correlates well with the reducibility data discussed above. H2-TPR results indicated that Au/Ce0.75-La0.25 nanorods has the lowest reducibility temperature and highest reducibility in the region of 50–400 °C, especially in the region of 50–150 °C, which could exactly approach the region of reaction temperature. In the process of reaction, the Ce0.75-La0.25 nanorod support served as oxygen carrier. The reducibility of Ce0.75-La0.25 nanorods could promote the formation of active oxygen. That is to say high reducibility of the catalyst, good activity the catalyst has. Au/Ce0.75-La0.25 nanorod catalyst subsequently has the best activity.
Effect of Gold Content
The catalytic activities for CO oxidation were measured from low conversion to 100% conversion for the Au/Ce0.75-La0.25 nanorod catalysts calcined at 300 °C for 2 h with a series of low gold contents. As shown in Fig. 10, all of the catalysts showed high catalytic activities. The CO conversion increased greatly with increasing gold content. The complete CO conversion could be attained at 50 °C over 0.5% Au/Ce0.75-La0.25 nanorod catalyst. The size and chemical states of gold nanoparticles are generally thought to be vital for the performance of supported gold catalysts. It has been reported that its gold nanoparticles with the diameter of < 5 nm would be suitable for the supported gold catalysts in the catalytic CO oxidation [27, 28]. The XPS data proved that gold in Au/Ce0.75-La0.25 nanorod catalyst exists in the form of cationic Au + . TEM images of the samples were also shown to investigate the diameter of gold nanoparticles in the catalysts. Consequently, the gold particles of Au/Ce0.75-La0.25 nanorods were detected as sub-nanometer. Taking into account the particle size, mass content, and chemical states of the gold nanoparticles, gold particles with small diameter highly dispersed on the surface of Ce0.75-La0.25 nanorods and interacted strongly with the support [17, 21, 23]. The strong interaction between gold particles and the support would help improve CO adsorption and accelerate active oxygen spillover to gold particles from the support, so 0.5% Au/Ce0.75-La0.25 nanorods which had relatively high content of gold should exhibit the best CO oxidation activity. In fact, 0.5% Au/Ce0.75-La0.25 nanorods indeed present high performance. The results demonstrated the activity of supported gold catalysts is strongly dependent on the gold nanoparticle size, chemical states, and the quantity of the active species, an increase of which implied an increase of the catalytic activity. In the case of Au/Ce0.75-La0.25 nanorod catalyst, catalysts with low gold content could also exhibits high activity at low temperature, which would promote the progress of supported gold catalyst. The results indicated that supported gold catalysts prepared by deposition-precipitation with pH value of 6–10 for HAuCl4 solution could have high catalytic activity due to small diameter of gold nanoparticles, corresponding with the references [8,9,10].
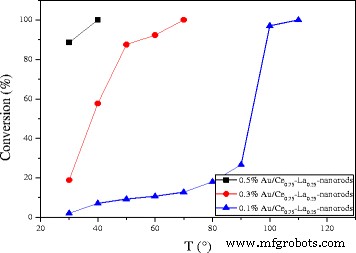
Catalytic activities of Au/Ce0.75-La0.25 nanorods with different gold goadings calcined at 300 °C for 2 h
图>Effect of Calcination Temperature
The effect of calcination temperature on the catalytic activity of 0.5%Au/Ce0.75-La0.25 nanorods is also shown in Fig. 11. The results indicated an increase in the activity of catalyst with the calcination temperature from 80 to 300 °C. The 0.5% Au/Ce0.75-La0.25 nanorod catalyst calcined at 200 °C could convert CO to CO2 completely at 80 °C. While for 0.5% Au/Ce0.75-La0.25 nanorod catalyst calcined at 80 °C, the temperature increased to 100 °C. The results showed that CO conversion increased with increasing calcinations temperature. Then, for the sample calcined at 400 °C, about 90% CO can be converted to CO2 at 100 °C and CO could be converted to CO2 completely at 120 °C. The sample calcined at 300 °C possessed the best catalytic activity. The catalytic performance of supported gold catalysts strongly depends on gold nanoparticle size and metal-support interaction due to “synergic effect” at the gold-support interface [10, 13, 15, 18]. The gold-support interaction largely depended on calcination temperature of catalysts. The electron could transfer from gold to the support [10]. Thus, with increasing calcination temperature, the charges on gold particles became increasingly positive, which is good for the enhancement of catalytic activity for CO oxidation. Here, as shown in the Fig. 5, size of gold particles in the catalysts calcined at 300 °C was small. The XPS data also indicated that gold was main Au δ+ after calcination at 300 °C. Thus, the stronger metal-support interaction could account for the relative good catalytic performance for catalysts calcined at 300 °C. From 80 to 300 °C, the higher the calcination temperature is, the stronger interaction exists between gold particles and support. As a consequence, from 80 to 300 °C, the activity of catalysts increased. However, after the 0.5% Au/Ce0.75-La0.25 nanorod catalyst calcined at 400 °C, complete conversion temperature increased. The main reason might be that the high-temperature treatment led to increased mobility and growth of gold nanoparticles, which correspondingly led to the loss of catalytic activity. The XPS also suggested that the catalysts calcined at 400 °C, Au was mainly Au 0 . It could be concluded that the activities of supported gold nanoparticles were influenced by both the oxidation state and the size of gold nanoparticles, and the appropriate calcination temperature was 300 °C.
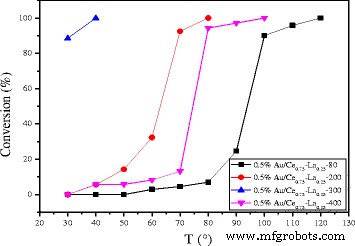
Catalytic activities of 0.5% Au/Ce0.75-La0.25 nanorods calcined at different temperatures
图>Stability Observations
The stability of the 0.3% Au/Ce0.75-La0.25 nanorod catalyst during CO oxidation at different reaction temperatures was measured, as shown in Fig. 12a. When the reaction was carried out at 70 °C, the initial CO conversion over 0.3% Au/Ce-La catalyst can reach 100% and has almost no change with continuously increasing reaction time. 0.3% Au/Ce-La catalyst with 60% CO conversion rate at 40 °C is also attained even after 10-h running period, and no obvious decline in CO conversion is observed. Although the catalytic activity of 0.3% Au/Ce0.75-La0.25 nanorod catalyst at 40 °C was lower than that of 0.3% Au/Ce0.75-La0.25 nanorod catalyst at 70 °C, the conversion of CO over the catalysts at both temperatures still seemed to be stable over 10 h on stream. It is thought that the catalyst was of good durability. It was clear that the activity over 0.3% Au/Ce0.75-La0.25 nanorod catalyst did not strongly depend on the reaction temperature. As the reaction temperature decrease the activation rate barely becomes little slower and then finally reaching a steady state in which the CO conversion was still around 90%. For comparison, the stability of 0.5% Au/Ce0.75-La0.25 nanorod catalyst at the reaction temperature of 40 °C with initial conversion of 100% was also provided in Fig. 12b. It was obvious that in 10 h, no decrease of CO conversion for 0.5% Au/Ce0.75-La0.25 nanorods was detected. The results depicted that with the change of gold content, Au/Ce0.75-La0.25 nanorods could still perform good stability.
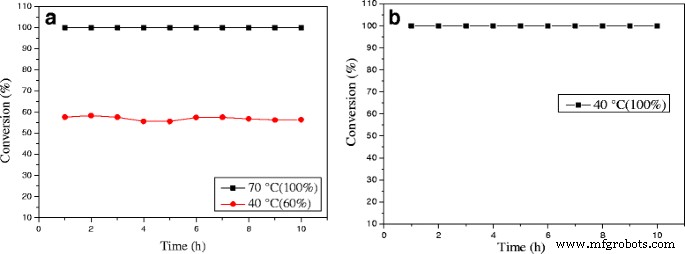
The stability of 0.3% Au/Ce0.75-La0.25 nanorods, reaction temperature:40 and 70 °C (a ) and 0.5% Au/Ce0.75-La0.25 nanorods, reaction temperature:40 °C (b ) for the CO oxidation
图>As engine efficiency increases and automotive exhaust temperatures decrease, traditional supported gold catalysts would be insufficient to meet emission regulations. And there are also a number of industrial catalytic processes which (e. g., the catalytic oxidation of CO in automotive exhaust gas) are sometimes carried out at high temperatures. Thus, the development of new catalysts that are active at lower temperature, yet still stable at periodic high temperatures, will be vital. In the two regards, catalysts with good activity at low temperature that are stable at high reaction temperatures are desirable. It is necessary to investigate their catalytic performance for CO oxidation at a certain high temperature which is a very stringent test for the stability of gold nanocatalysts against sintering. In the present work, the stability of 0.3% Au/Ce0.75-La0.25 nanorod catalyst was also measured at 200 °C (100%) for high-temperature treatment. As shown in Fig. 13, no decline of catalytic activity was observed within 50 h indicates that the catalyst keeps good stability within 50 h. Remarkably, very few serious gold sintering occurred during the reaction. It indicated that 0.3% Au/Ce-La catalyst can exhibit good catalytic stability at both low and high reaction temperatures.
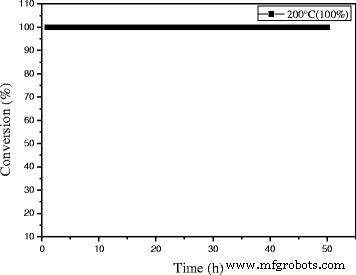
The stability of 0.3% Au/Ce0.75-La0.25 nanorods at the reaction temperature of 200 °C for CO oxidation
图>Reaction Mechanism Speculate
Combined with H2-TPR and XPS experiments, it suggested that CO oxidation over LaO x -doped CeO2-supported Au catalysts might follow the Langmuir–Hinshelwood + Redox mechanism [1, 20, 26, 30, 32]. The XPS results suggest that there are Ce 3+ and Ce 4+ on the surface of the catalyst. H2-TPR data also proved that reducibility of this binary Ce-La nanorod oxides could be promoted by Au deposition. The reducibility of Au/Ce-La nanorods was much higher than pure Au/CeO2 or Au/LaO x catalysts. This would help the produce of oxygen vacancies. The oxygen vacancy is a very lively activity center. The active center can promote the activation of O2. Thus, the CO oxidation reaction could become more easily. There are also amount of adsorbed oxygen species on the surface of catalyst. Usually adsorbed oxygen species play an important role in the oxidation of CO. The O2 of the reaction will form the chemisorbed oxygen, and the oxygen vacancy would be replenished by O2 of reaction gas to form new active lattice oxygen. XPS data also proved that gold in the catalysts was mainly Au δ+ species, which would accelerate the adsorption of CO. The possible reaction mechanisms of Au/Ce-La nanorod catalyst could be described as follows. Firstly, CO and O2 were chemisorbed on the surface of the catalysts. Then, the chemisorbed oxygen directly reacts with CO, or the active lattice oxygen of the catalyst reacts with CO, and the catalyst produced the oxygen vacancy with oxygen from gas-phase O2. At last, CO was oxidized into CO2 (shown in Fig. 14).
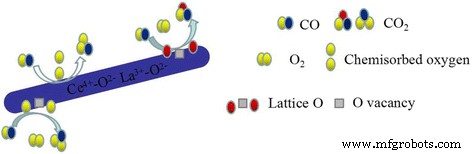
Proposed CO reaction pathways over the catalysts, Au/Ce-La nanorods
图>Conclusions
In summary, a series of mixed Ce-La nanorods with various amounts of La was prepared via a simple hydrothermal reaction at high concentration of NaOH and without surfactant. Gold was loaded by deposition-precipitation. After La doping, the composite could retain the initial rod morphology. As a result, Ce-La nanorods with 25 at.% La maintained the optimal nanorods with the length of 0.6 um and the diameter of 3–5 nm. Gold particles were dispersed well on the support. The reducibility of Ce-La nanorods could be affected significantly by LaO x doping. The deposition of gold had important influence on the reducibility of catalyst. Thus, the CO oxidation activity of Au/Ce-La nanorods was essentially changed in comparison with pure Au/CeO2 and Au/La nanorods. One percent Au/Ce0.75-La0.25 nanorods could convert CO to CO2 completely at 30 °C. Further increase in La content results in decreased activity due to the decrease in reducible oxygen sites. The Au/Ce0.75-La0.25 nanorod catalyst with low gold concentration also showed high activity. With the increase of gold content, there is an increase in the activity. The stability test of 0.3% Au/Ce0.75-La0.25 nanorods indicated that the catalyst not only kept 100% conversion after continuous operation for 10 h under 70 °C but also showed no deactivation after 10 h on stream at 40 °C. As expected, the activity of 0.3% Au/Ce0.75-La0.25 nanorods also retained a 100% CO conversion during 50 h at 200 °C. The results revealed that LaO x as the dopant could grow together with CeO2 in one rod. The 1D binary mixed Ce-La nanorods could be a good support for precious metal group catalysts with low content of gold.
纳米材料
- 用于合成和生物医学应用的荧光纳米材料的进展和挑战
- 氧化铜纳米颗粒对大肠杆菌的生物合成、表征和抗菌潜力评估
- 通过激光脉冲和溅射技术合成导电二氧化硅纳米纤维/金纳米粒子复合材料
- 石墨烯和氧化石墨烯的体外和体内生物安全和抗菌能力
- ZnO 纳米晶体的合成及其在倒置聚合物太阳能电池中的应用
- 聚(3,4-亚乙基二氧噻吩)/金/石墨烯复合材料的固态加热合成及其在安培法测定亚硝酸盐和碘酸盐中的应用
- 通过蒸发诱导自组装和增强的气敏特性简便合成虫孔状介孔氧化锡
- Cu2ZnSnSe4 纳米片的一锅法合成及其可见光驱动的光催化活性
- 桔梗皂苷(桔梗)用于金和银纳米颗粒的绿色合成
- 金属和金属氧化物纳米粒子的绿色合成及其对单细胞藻类莱茵衣藻的影响
- 氧化锌纳米粒子的特性及其对微生物的活性
- 无配体铱纳米粒子的简便合成及其体外生物相容性