用于增强药物递送的喉癌细胞和免疫细胞内聚合物纳米颗粒的细胞内化的定量评估
摘要
基于聚(乳酸-乙醇酸共聚物)(PLGA)的纳米药物的临床转化受到限制,部分原因是吞噬细胞的非特异性吞噬作用导致递送效率低下。了解癌细胞和免疫细胞之间的纳米颗粒相互作用在很大程度上仍然难以捉摸。在这项研究中,首先对荧光 PLGA 颗粒(100 nm、500 nm 和 1 µm)在单培养或共培养系统中对有或没有单核细胞/巨噬细胞的喉癌细胞的细胞内化进行了定量研究。浓度为 5–20 µg/mL 的 PLGA 颗粒显示出优异的生物相容性,但 20 µg/mL 的 500 nm 和 1 µm PLGA 颗粒会略微降低细胞活力。显微镜观察发现,癌细胞和巨噬细胞都能有效摄取所有三种尺寸的颗粒;然而,定量荧光检查表明,与单一培养中的相比,共培养中所有 PLGA 粒子的癌细胞摄取指数(每个癌细胞的平均细胞内粒子荧光标准化为每个巨噬细胞的平均细胞内粒子荧光)显着下降(1.35-1.05, 1.50–0.59 和 1.4–0.47(对于 100 nm、500 nm 和 1 µm 颗粒)。使用流式细胞术的定量分析进一步证实了共培养物中癌细胞的吸收指数降低,但每个巨噬细胞的颗粒计数更高。还发现在 PLGA 治疗后,通过巨噬细胞融合形成的多核巨细胞增加,这可以进一步作为一种潜在的肿瘤药物递送方法加以利用。总的来说,这些发现为纳米颗粒-免疫-癌细胞的相互作用提供了新的见解,可能有助于基于PLGA的纳米载体在喉癌治疗中的应用。
介绍
癌症是全球主要的死亡原因之一,2018 年报告的新死亡病例约为 1000 万[1]。喉癌(癌细胞起源于喉部)是头颈部鳞状细胞癌 (HNSCC) 中第二常见的恶性肿瘤,2018 年约有 180,000 例新发病例和 95,000 例死亡 [2]。目前,靶向药物被开发为一种可选治疗方法,以应对传统疗法(如手术、放疗和化疗)带来的挑战 [3]。例如,通过开发新型药物纳米载体(即 、微针)、个性化抗癌药物和治疗性抗体靶向递送系统 [4]。
纳米载体已被广泛用于负载抗癌药物,如顺铂、紫杉醇和多西他赛,以提高其水溶性、生物利用度和稳定性,从而改善药物递送和疗效 [3, 4]。一般来说,基于纳米颗粒 (NP) 的药物递送允许递送范围广泛的其他物质(例如 、蛋白质、抗体、疫苗和核酸)到动物模型和患者身体的特定区域 [4, 5]。然而,所谓的增强渗透性和保留 (EPR) 效应导致不同类型癌症的靶向效率差异很大 [6]。最近的证据表明,纳米药物载体(这里是金 NPs)在肿瘤上的沉积优先取决于转胞吞作用 [7],这是一种生物跨细胞转运,其中物质/NPs 从一侧跨细胞转移到另一个包括内吞作用、囊泡转移和胞吐作用的过程。然而,应该指出的是,关于靶向药物递送机制的矛盾结果强调了利用从体外细胞培养到离体组织培养的多种实验策略来理解细胞与纳米药物或纳米颗粒相互作用的基础至关重要和体内动物研究。
许多纳米载体,如脂质体、白蛋白 NPs (NPs)、二氧化硅 NPs 和聚(乳酸-乙醇酸共聚物)(PLGA)已在临床上用于治疗包括喉癌在内的不同类型的癌症 [4]。基于聚合物的纳米粒子如胶束和 PLGA 在生物医学应用中具有巨大的潜力,因为它们通过简单的混合或共价结合形成了多功能的纳米药物制剂、优异的自组装能力、高载药能力和生物相容性 [8, 9 ]。例如,负载有阿霉素和吲哚菁绿的聚乙二醇 (PEG) 包被的 PLGA 纳米载体可以实现乳腺癌的协同化学光热治疗 [10]。此外,体外和体内研究表明,载有顺铂的胶束对 HNSCC 原位肿瘤(i.e. , SAS-L1 和 HSC-2) [11]。尽管几种基于聚合物的纳米药物载体如 PLGA 和胶束已被批准用于临床或正在临床试验中进行评估 [4, 6],但更多的聚合物纳米药物正在针对不同的头颈癌细胞进行临床前研究动物系和异种移植肿瘤模型[11,12,13,14]。
科学证据强调了宿主对纳米药物载体的免疫反应的重要性,因为一旦这些纳米颗粒进入人体,它们就不可避免地被免疫系统识别。巨噬细胞被认为是细胞宿主防御的第一道防线,专门用于中和和清除过敏原、微生物和外来颗粒(例如 ,纳米载体)通过吞噬作用和随后引发的免疫反应。由于 NPs 在单核吞噬细胞系统 (MPS) 中的有效积累,例如肝脏中的库普弗细胞和脾脏中的红髓巨噬细胞,大多数新型设计的纳米载体未能靶向递送到体内特定的病变区域或肿瘤[15]。因此,了解单核细胞和巨噬细胞等免疫细胞对纳米颗粒的细胞摄取机制至关重要,因为它决定了纳米载体在相关组织和生物体液中的寿命。因此,新兴的体外细胞共培养系统逐渐引起纳米医学和毒理学领域越来越多的关注,因为越来越需要获得更有意义的结果,以更好地反映体内状况[16]。事实上,共培养系统已被证明在模拟健康和患病组织状态方面表现出真实的情况 [17],并已可靠地用于 NP 细胞摄取和药物吸收研究 [18,19,20,21]。利用共培养癌细胞和免疫细胞模型通常为探索这些纳米材料进入细胞的吸收途径和机制提供了合适的平台,这可能有助于设计更好地靶向癌细胞同时减少 NP 吞噬作用的纳米载体。因此,在免疫细胞存在的情况下确定癌细胞中纳米颗粒的吸收效率和命运至关重要。大多数纳米载体的直径设计为 50-200 nm,以利用 EPR 效应并延长血液循环,而据报道,较大的纳米颗粒 (> 500 nm) 可被 MPS 有效清除 [6]。 PLGA 是一种 FDA 批准的纳米载体,具有不同的尺寸(100、500 和 1000 nm),因此被选择来研究 UM-SCC-17A(一种经典的喉鳞状细胞癌细胞系)[22] 和 THP- 1(人类急性单核细胞系)细胞。多项体外研究应用 PLGA 纳米载体递送药物杀死单培养的 HNSCC 癌细胞 [12, 13, 23],这是第一项探讨免疫细胞和喉癌细胞同步摄取 NP 的效率和机制的研究。通过采用不同大小的PLGA建立共培养模型,这可能为开发用于HNSCC治疗的新型安全设计纳米药物提供基础。
材料和方法
材料
本研究使用了三种不同尺寸的商业化 PLGA 颗粒 (Sigma-Aldrich),包括 100 nm、500 nm 和 1000 nm。所有颗粒都装有绿色荧光团,其光学激发和发射 (ex/em) 波长为 460 nm 和 500 nm。所有颗粒均以粉末形式接收并悬浮在终浓度为 10 mg/mL 的蒸馏水中以供进一步使用。三个粒子的流体动力学直径和 zeta 电位通过动态光散射 (DLS) 进行,使用 Malvern Zeta Sizer Nano 仪器(Malvern Instruments Ltd.,Malvern,UK)进行。每个颗粒的悬浮液在 80 µl 蒸馏水中 1:100 稀释,用于 DLS 测量。根据制造商的指示,对每个颗粒进行了 3 次重复测量。
UM-SCC-17A 和 THP-1 的细胞培养和颗粒暴露
人喉癌细胞系UM-SCC-17A和人单核/巨噬细胞系THP-1分别购自美国Sigma-Aldrich和上海恒亚生物技术公司,用于构建体外模型。这项研究。 UM-SCC-17A 细胞和 THP-1 细胞在补充有 10% FBS(Gibco,德国)和 1% 青霉素-链霉素溶液(Gibco,德国)的 DMEM [22] 或 RPMI-1640 细胞培养基中培养,温度为 37 °C,含 5% CO2。在细胞接种以分化为巨噬细胞之前,THP-1 细胞暴露于 100 nM 佛波醇 12-肉豆蔻酸酯 13-乙酸 (PMA)(Sigma,美国)溶液 72 小时。两个细胞每3天用0.5%胰蛋白酶-EDTA传代,每天检查细胞形态以确保细胞健康。
将细胞以0.1 × 10 6 的密度接种于24孔板中 细胞/孔用于 WST-1 和 LDH 测定的单培养细胞,或用于荧光显微镜检查的 24 孔板中的无菌玻璃盖玻片。对于共培养模型,首先将 UM-SCC-17A 细胞以 50,000 个/孔的密度接种在 24 孔板中过夜,然后加入 50,000 个/孔的 THP-1 细胞。将颗粒悬浮在 500 µl 单独培养的 UM-SCC-17A 和 THP-1 细胞的各自细胞培养基中或用于共培养细胞的 1:1 混合细胞培养基中,并暴露于细胞样品 24 小时,最后WST-1 和 LDH 测定的浓度为 5、10 和 20 µg/mL,荧光显微镜的浓度为 10 µg/mL。
对于 FACS 测量,以与之前所述相同的方式将细胞以 250,000 个细胞/孔的密度或每个 125,000 个细胞/孔的密度接种到 12 孔板中,以用于共培养模型。细胞在 1 mL 细胞培养基中过夜生长,并以 10 µg/mL 的终浓度暴露于 PLGA 颗粒 24 小时。
细胞活力测定
根据制造商的说明,通过细胞增殖试剂 WST-1 试剂盒(Roche,德国)测定细胞活力。简而言之,WST-1 溶液在用于单培养 UM-SCC-17A 或 THP-1 的相应细胞培养基或用于共培养细胞的 1:1 混合细胞培养基中以 1:10 稀释。暴露后排出上清液,将细胞与 500 µl WST-1 检测工作溶液在 37°C 下孵育 30 分钟。收集样品并以 14,000 rpm 离心 10 分钟以去除颗粒。使用 Infinite ® 在 450 nm 波长下测量溶液的吸光度(OD 值) F200(Tecan,美国)。通过减去仅含有WST-1工作溶液的空白样品的值校正吸光度值,并将相对细胞活力与未处理的对照样品进行比较。
细胞膜渗漏检测
使用商业细胞毒性检测试剂盒 (LDH) (Roche, Germany) 测量乳酸脱氢酶 (LDH) 的释放,以确定 PLGA 颗粒的细胞毒性。暴露后 24 小时收集细胞上清液,以 14,000 rpm 离心 10 分钟,然后在 200 µl 完全细胞培养基中按 1:10 稀释。阳性对照定义为通过将细胞与 0.2% Triton X-100 在 37°C 下孵育 15 分钟,并在 200 μl 完全细胞培养基中稀释 1:50 来释放 LDH 的总释放量。在室温下将样品与 100 份 LDH 检测工作溶液孵育 30 分钟,然后使用 50 µl 1% HCl 停止反应。使用Infinite®F200(Tecan,USA)测量492 nm波长处的吸光度,并根据下式计算相对LDH浓度
$${\text{Relative}}\;{\text{LDH}}\;{\text{concentration}} =\left( {{\text{sample}}\;{\text{OD}}{- }{\text{blank}}\;{\text{OD}}} \right)/\left( {{\text{positive}}\;{\text{control}}\;{\text{OD} }{-}{\text{blank}}\;{\text{OD}}} \right) \times 5 \times 100\% .$$荧光显微镜
在荧光显微镜下通过 Hoechst 染色观察细胞以定位颗粒。在颗粒暴露 24 小时后,将细胞用 PBS 洗涤 3 次,并在室温下与 4% 甲醛一起孵育 10 分钟。用甲醛固定后,将细胞与含有 1:1000 稀释 Hoechst 的 200 µl 染色溶液一起孵育 和 1% BSA 的 PBS 溶液 30 分钟。然后,将盖玻片倒置在载玻片上,并用一滴 DAKO 荧光抗褪色剂保持以进行可视化。荧光显微镜设置了四个光学通道,包括细胞形态的亮场、细胞核的 DAPI 和粒子的 GFP。记录每个荧光图片的粒子通道的曝光时间并用于不同粒子的荧光强度的均质化,并使用 ImageJ (https://imagej.nih.gov/ij) 随机选择的区域通过荧光强度计算细胞内粒子/)。在单培养或共培养的 UM-SCC-17A 细胞之间比较了不同颗粒的摄取指数。简而言之,计算内化颗粒的平均荧光强度 (MFI),例如每种细胞类型的 50 个细胞,其被确定为总荧光强度 (I 总)特定区域和自发荧光(I auto) 无粒子区域方程 [24, 25] 中相同大小的区域。癌细胞的摄取指数由 UM-SCC-17A 的 MFI 确定,该 MFI 标准化为单一培养或共培养模型中的 THP-1 细胞的 MFI。计算如下:
$${\text{uptake}}\; {\text{index}} =\frac{{I_{{{\text{total1}}}} - I_{{{\text{auto1}}}} \left( {{\text{MFI}}}_{ {{\text{UM}} - {\text{SCC}} - 17A}} } \right)}}{{I_{{{\text{total2}}}} - I_{{{\text{auto2} }}} \left( {{\text{MFI}}_{{{\text{THP}} - 1}} } \right)}}$$荧光激活细胞分选 (FACS)
进行荧光激活细胞分选 (FACS) 以测量三种粒子的吸收能力。暴露后 24 小时,将细胞用 PBS 洗涤 3 次以去除细胞培养基和解离颗粒,并与 200 μl 0.5% 胰蛋白酶-EDTA(Gibco,德国)在 37°C 下孵育 4 分钟以从板中去除细胞.然后,通过加入2ml完全细胞培养基终止反应,将细胞悬液移至玻璃管中进行FACS测量并在300 × G,4℃下离心5分钟。轻轻排出上清液,将细胞悬浮在 200 µl PBS 中并保存在冰上。首先使用前向 (FSC-A) 侧 (SSC-A) 散射从碎片和死细胞中对活细胞进行门控。然后,使用 APC 通道与 FSC-A 分析共培养的细胞,以根据细胞的大小将 UM-SCC-17A 细胞与巨噬细胞分开。每个样品共分析了 30,000 个细胞,计算了每个细胞的平均荧光强度,并对不同颗粒进行了标准化。同时,在颗粒与细胞孵育 24 h 后,收集细胞培养上清液、洗涤缓冲液(洗涤 3 次以去除附着在细胞表面的残留颗粒)和细胞(胰蛋白酶消化),使用微孔板测量荧光强度读卡器(Infinite®F200、Tecan)。通过这种方法,可以确定每组细胞摄入颗粒的百分比,例如,在 12 孔/板(总共 250,000 个细胞)中,以 5 µg 100 nm 的剂量暴露于单个细胞中的约 30,000 个颗粒PLGA 颗粒,导致平均 13,000 个颗粒被单个细胞内化,约占递送到细胞的应用剂量的 43%。该传递百分比可进一步用于计算 FACS 中每种类型细胞在共培养系统中的粒子数。
细胞融合的量化
巨噬细胞与多核巨细胞 (MGC) 融合被定义为在正常细胞质内形态学上含有两个或多个细胞核的巨细胞,如文献中所记录的,在明场图像和染色后的荧光图像中可以清楚、同步地识别[26] .细胞融合的百分比是通过归一化到总细胞计数的 MGC 核数(手动计数)计算的,这是用 ImageJ 中的自动方法确定的(https://imagej.nih.gov/ij/)。>
统计分析
GraphPad V8.0 软件(GraphPad Software Inc., San Diego, CA, USA)用于统计分析和结果可视化。在单向 ANONA 之后,分别进行 Holm-Sidak 方法或 t 检验以比较多组结果或两组结果。所有实验均以独立的三次重复进行,数据表示为平均值 ± 标准偏差(STD)。 p 的结果 值 <0.05 (*) 和 p <0.01 (**) 被认为是显着的。
结果与讨论
聚(乳酸-乙醇酸共聚物)颗粒的表征
PLGA 颗粒的荧光显微照片(图 1a、b、c)表现出强大的荧光强度,并且在制备后 14 天的过程中荧光信号没有显着降低(附加文件 1:S1),表明相对均匀的尺寸分布和高荧光稳定。正如供应商所指出的,100、500 和 1000 nm PLGA 颗粒的尺寸分别为约 80.6 ± 19.3 nm、542.6 ± 128.3 nm 和 951.9 ± 237.5 nm,(图 e.f)、e.f.根据 zeta 电位测量,发现粒子上的平均表面电荷为 - 20.6 ± 5.3、-17 ± 4.6 和 - 16.5 ± 3.5,0 和 1 的多分散指数分别为 0.057、0.056 和 10,062 nm 颗粒,后者表明它们的高度单分散标准。涡旋所有颗粒,然后在水浴中超声处理 5 分钟以在很大程度上消除颗粒聚集。然而,由于不可避免的颗粒聚集(约 3-4%),我们观察到了对应于 100 和 1000 纳米颗粒的小峰,直径约为 4-6 微米。
<图片>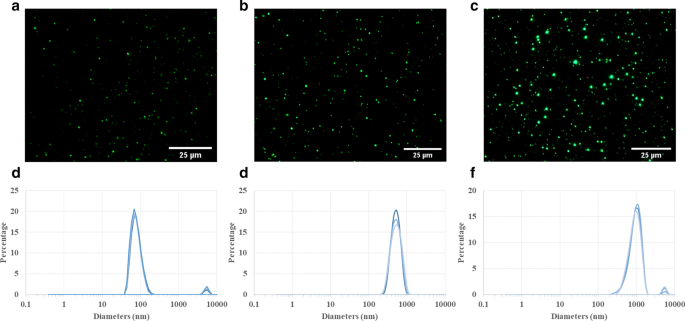
PLGA 颗粒的表征。荧光显微照片显示了不同的尺寸 (a 100 纳米,b 500 纳米和 c 1 µm) 的 PLGA 颗粒。这些颗粒可以在奥林巴斯光学显微镜下检测到,在水悬浮液中表现出相对均匀的粒度分布。动态光散射 (DLS) 测量显示按体积加权的 100 纳米 (d ), 500 (e ), 1 µm (f ) PLGA 颗粒。尽管在 100 nm 和 1 µm PLGA 悬浮液中有一小部分颗粒聚集(较小的峰:3-4%),但由于主峰较窄,因此总体粒径分布非常均匀
图>细胞活力和细胞毒性评估
由于其卓越的生物相容性和生物降解性,PLGA 颗粒已被 FDA 和欧洲医药管理局批准用于生物医学应用 [27, 28]。因此,PLGA 颗粒目前已用于临床并广泛应用于临床前研究,作为将药物输送到特定病变区域或肿瘤的纳米载体。然而,PLGA 颗粒的靶向效率和治疗功效至少部分受到免疫系统的阻碍。例如,肝脏中库普弗细胞的高粒子吞噬作用极大地限制了纳米药物载体进入肿瘤部位[15]。因此,建立先进的体外模型来研究癌细胞、免疫细胞和颗粒之间的相互作用,以更好地模拟药物递送的体内情况至关重要。 UM-SCC-17A 是一种独特的喉鳞状癌细胞系,从原发性喉癌标本中分离出来 [29]。然而,关于其在共培养系统中的体外细胞摄取效率的信息(e.g. , 巨噬细胞和癌细胞的共孵育) 仍然不足,这是一个需要解决的问题,以提高体内反应的预测能力。此外,已经证明颗粒大小和表面涂层在动物模型和细胞培养物中向实体瘤、病变部位和癌细胞的递送能力方面起着重要作用 [30,31,32,33]。在这里,我们因此将三种尺寸的 PLGA 应用于 UM-SCC-17A 癌细胞,以研究粒径对单培养和共培养系统中细胞摄取和细胞内分布的影响。
本研究使用 WST-1(4-[3-(4-碘苯基)-2-(4-硝基苯基)-2H-5-四唑]-1,3-苯二磺酸盐)方法测定单培养 THP-1 的细胞活力和 UM-SCC-17A 细胞,以及两种细胞的共培养。尽管在所有测试浓度下用 100 和 500 nm PLGA 处理的组中没有发生明显的细胞死亡,但最高浓度 (20 µg/mL) 的 500 nm 和 1 µm PLGA 颗粒显着降低了单培养 UM-SCC-17A 中的细胞活力细胞(图 2a)。正如预期的那样,与浓度为 5–20 µg/mL 的所有三种类型的 PLGA 孵育 24 小时后,THP-1 细胞活力没有受到显着影响(与未处理细胞相比,其活力≥ 95%,其活力被视为 100% ,图 2b)。与单一培养 UM-SCC-17A 细胞的结果相同,共培养系统中的细胞活力并未因此处使用的三种剂量的 100 和 500 nm PLGA 颗粒的存在而改变,而在用 20 µg/mL 1 µm PLGA(图 2c)。
<图片>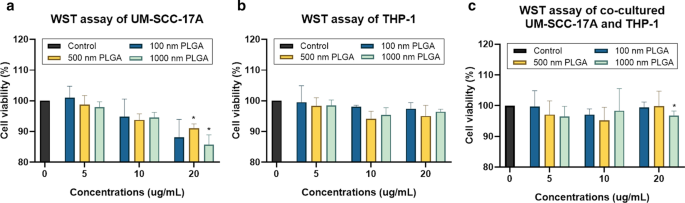
使用 WST 测定法测定单培养和共培养细胞中的细胞活力。 UM-SCC-17A (a ) 和 THP-1 细胞 (b ),以及共培养的 UM-SCC-17A 和 THP-1 细胞 (c ) 用三种尺寸的不同浓度 (5-20 µg/mL) PLGA 颗粒处理
图>利用 LDH 测定法评估颗粒细胞毒性是通过测量细胞外 LDH 的量来确定细胞膜的渗漏 [9]。这种细胞质酶释放到细胞培养上清液中是细胞膜损伤的特征,导致不可逆的细胞死亡。尽管在用不同剂量的 1 µm PLGA 处理的单培养 UM-SCC-17A 细胞中,LDH 水平随着更高剂量的轻微增加,即使在 20 µg/mL 的最高浓度下也没有观察到对细胞的明显细胞毒性(图 3a) .毫不奇怪,这里使用的所有三种尺寸的 PLGA 在不同剂量下都不能诱导大量 LDH 释放到上清液中,表明对单培养 THP-1 细胞的毒性作用微不足道,这也与上述细胞活力结果高度一致(图. 3b)在共培养实验中,就释放的 LDH 水平而言,所有不同浓度的颗粒对两种细胞都显示出优异的生物相容性(图 3c)。一般来说,这里使用的从 100 nm 到 1 µm 的广泛粒径范围涵盖了纳米载体的典型尺寸(50-200 nm),例如脂质体、胶束、树枝状聚合物、聚合物和微型细胞。该范围还包括亚微米级颗粒(500 纳米颗粒仍可被视为纳米颗粒,例如 尺寸约为 500 nm 的颗粒在肺中具有与 10-100 nm [34] 和微米级 1 µm 纳米颗粒相同的清除途径。除了最高浓度的 500 nm 和 1 µm PLGA 颗粒对 UM-SCC- 17A和共培养细胞(但85%以上的细胞存活),表明它们有利于在药物递送系统中的应用。
<图片>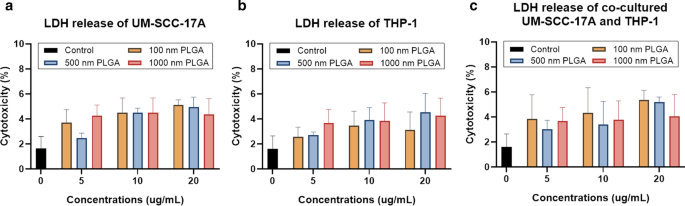
使用 LDH 测定法测定对单一培养和共培养细胞的细胞毒性。 UM-SCC-17A (a ) 和 THP-1 细胞 (b ) 和共培养细胞 (c ) 用 PLGA 颗粒处理。在单培养和共培养系统中培养颗粒(此处使用不同大小和浓度)和细胞 24 小时后,未观察到显着的细胞死亡
图>PLGA 在单培养和共培养系统中的细胞摄取能力
图 4 显示了单一培养和共培养中的细胞形态(图 4a、d、g)、细胞核和 100 nm 纳米颗粒(图 4b、e、h)以及合并的放大图像(图 4c、f、i)文化系统。在对照组的未处理细胞中未观察到颗粒(附加文件 1:S2)。在巨噬细胞单培养细胞的细胞质中观察到大量的大尺寸 100 nm PLGA NPs 团块(图 4c)。同时,单一培养的 UM-SCC-17A 表现出优异的 100 nm PLGA 吸收能力,这由细胞膜内观察到的亮绿色荧光信号证明(图 4f)。为了更好地说明 PLGA 颗粒在 THP-1 或 UM-SCC-17A 细胞中的细胞内积累以及共培养物中的细胞外颗粒,应用了明场图像与荧光图像的叠加,如附加文件 1:S3)。在共培养系统中,两种细胞类型在明场图像中在形态和细胞大小方面是可区分的,其中巨噬细胞呈现圆形和小尺寸,而癌细胞呈现大尺寸和细长形状。然而,由于共培养系统中巨噬细胞对 100 nm PLGA 的高吸收,癌细胞显示出细胞摄取不足。这是意料之中的,因为巨噬细胞被认为是一种有效且特定类型的免疫细胞,可以执行清除外来微生物、过敏原和颗粒的吞噬功能。类似地,图 5 和图 6 分别显示了单细胞或混合细胞培养物中 500 nm 和 1 µm PLGA 颗粒的细胞摄取能力的定性分析。值得注意的是,在用此处使用的 10 mg/mL 浓度的不同大小的 PLGA 颗粒处理后,两种细胞形态都没有改变(图 4a、d、g、图 5a、d、g 和图 6a, d,g)。 500 nm 和 1 µm PLGA 颗粒在单独的 UM-SCC-17A 细胞(图 5e、f 和图 6e、f)中显示出比单独的 THP-1 细胞(图 5b、c 和图 5b、c 和图 5e、f)更强的荧光强度. 6b,c)。在共培养孵育中(图 5h,i 和图 6h,i),癌细胞中有明显的信号降低,其中发现粒子荧光强度低于巨噬细胞的荧光强度。巨噬细胞快速有效地吞噬所有类型的颗粒,因此癌细胞无法在共培养系统中摄取足够的颗粒。为了量化吞噬作用对癌细胞对不同尺寸 PLGA 颗粒的吸收能力的影响,进行了半定量光学分析。简而言之,基于例如 50 个单独的载有粒子的细胞计算粒子荧光强度以获得两种细胞类型的平均值,并且通过平均每个癌症的细胞内粒子荧光强度来确定癌细胞的摄取指数细胞标准化为巨噬细胞(图 7)。在单一培养中,发现 100 nm PLGA 的癌细胞摄取指数约为 1.34 ± 0.19,这表明癌细胞摄取的颗粒多于单细胞培养中巨噬细胞摄取的颗粒(图 7)。这并不奇怪,因为 PLGA 颗粒的高粘度和更大尺寸的喉癌细胞。类似地,在单一培养中,500 nm 和 1 µm PLGA 也被癌细胞大量内化,吸收指数分别约为 1.5 ± 0.25 和 1.4 ± 0.31。在共培养系统中存在巨噬细胞时,大颗粒的吸收指数显着下降(0.59 ± 0.12 和 0.47 ± 0.1)。同时,在混合细胞培养物中孵育 100 nm PLGA 颗粒 24 小时后,两种细胞类型的细胞内化能力相同。 Overall, the results accumulated here suggested that the ingestion of particles by cancer cells and macrophages might depend on different routes of uptake pathway in single-cell culture and co-culture environment. Moreover, the presence of macrophages reduced the cellular internalization of PLGA particles by cancer cells particularly for large ones, a biological event that has been observed in in vivo nanomedicine drug delivery studies [3, 15].
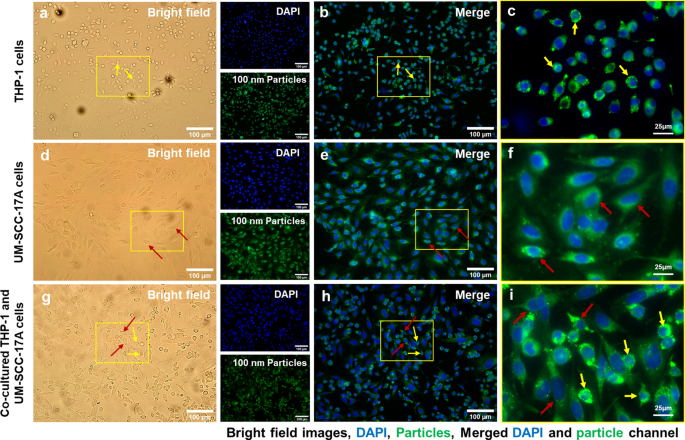
Microscopic examination of cellular internalization of 100 nm PLGA nanoparticles in monoculture and co-culture cells. Single type of cells or mixed cells was treated with 100 nm PLGA nanoparticles for 24 h at 37 °C at a concentration of 10 µg/mL. Cells were observed under bright-field (a , d , g ) and fluorescent channels (b, e, and h) with particles observed at the green channel and cell nuclei in the blue channel after stained with Hoechst. Massive cellular uptake of 100 nm PLGA nanoparticles can be visualized in magnified images (c , f , i ) for monoculture THP-1 macrophages (yellow arrows) and laryngeal cancer cells UM-SCC-17A (red arrows). In the co-culture system, 100 nm PLGA nanoparticles were still highly ingested by macrophages but less efficient for cancer cells compared to single cultured cells
图>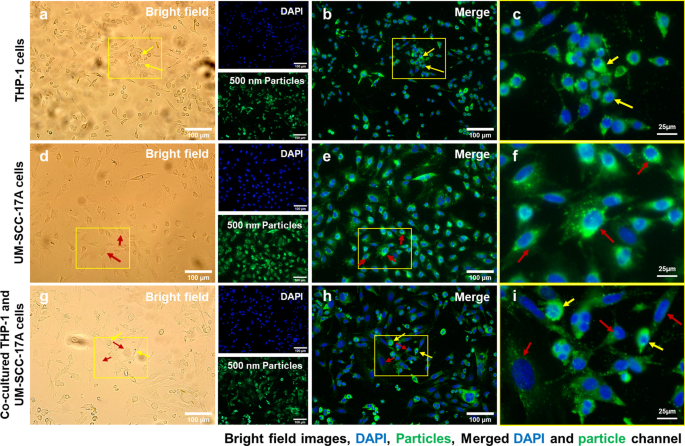
Microscopic examination of cellular internalization of 500 nm PLGA nanoparticles in monoculture and co-cultured cells. Single type of cells or mixed cells was treated with 500 nm PLGA nanoparticles for 24 h at 37 °C at a concentration of 10 µg/mL. Cells were observed under bright-field (a , d , g ) and fluorescent channels (b , e , h ) with particles observed at the green channel and cell nuclei in the blue channel after stained with Hoechst. Massive cellular ingestion of 500 nm PLGA nanoparticles can be visualized in magnified images (c , f , i ) for monoculture THP-1 macrophages (yellow arrows) and laryngeal cancer cells UM-SCC-17A cells (red arrows). In the co-culture system, 500 nm PLGA nanoparticles were still highly uptake by macrophages, while cancer cells had inadequate particle internalization
图>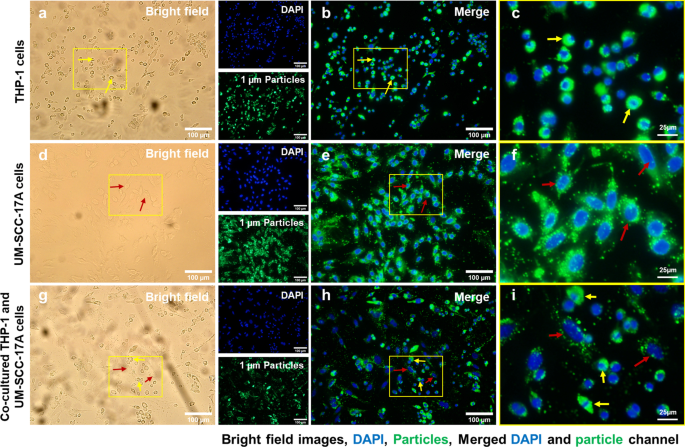
Microscopic examination of cellular internalization of 1 µm PLGA particles in monoculture and co-culture cells. Single type of cells or mixed cells was treated with 1 µm PLGA particles for 24 h at a concentration of 10 µg/mL. Cells were observed under bright-field (a , d , g ) and fluorescent channels (b , e , h ) with particles observed at the green channel and cell nuclei in the blue channel after stained with Hoechst. A large amount of particle uptake and tremendous accumulation of 1 µm PLGA particles can be visualized in magnified images (c , f , i ) for monoculture THP-1 (yellow arrows) and UM-SCC-17A laryngeal cancer cells (red arrows). In the co-culture system, 1 µm PLGA particles were still efficiently uptake by macrophages, while cancer cells had insufficient particle ingestion
图>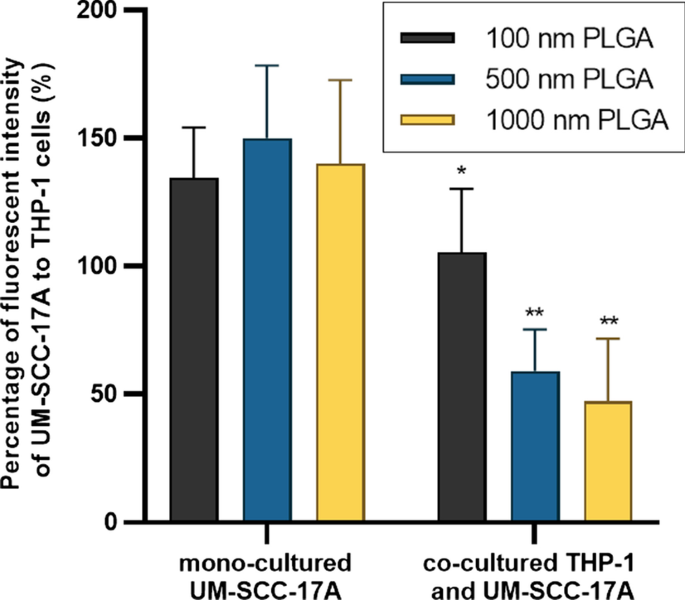
Quantitative analysis of PLGA particle internalization in UM-SCC-17A cells in monoculture and co-culture systems. The percentage of particle fluorescent intensity in UM-SCC-17A cells normalized to that in THP-1 cells for solely and mixed cultured cells
图>Quantification of cellular uptake by flow cytometry
Flow cytometry is widely used to determine of particle-cell interplay in a quantitative manner, for example, the size-dependent uptake of polystyrene particles with sizes ranging from 20 to 1000 nm by dendritic cells in vivo [35]. FACS was thus utilized for analysis of the percentage of NP-positive cells and particle number in those NP-positive cells in monoculture and co-culture systems. Then, average NP counts in a single cell were calculated by determining the total fluorescence intensity in particle-laden cells normalized to the fluorescence of total applied dose. For example, about 70% of 500 nm PLGA was deposited in the cancer cells, whereas the residual was kept in the supernatant of cell cultures (Additional file 1:S4). This fluorescence intensity measured by a Tecan reader was then compared to the average/total FACS signals (FITC signal values of P5 and P6 in Fig. 8,) to estimate the particle number in the co-culture system. As seeded in the co-cultured cells, about half of the cells were recognized as macrophages and the rest were considered as cancer cells. It was observed that approximately 13,000 of single 100 nm PLGA particles accumulated in cancer cells and 9700 particles in macrophages (Fig. 8i) in the monoculture, consistent with the above microscopic examinations. A much lower particle number (164 ± 30 and 45 ± 15) was ingested by monocultured cancer cells for 500 nm and 1 µm PLGA, with a slightly lower particle count in the respective macrophages (Fig. 8i). The number of particles ingested by single cells is in great agreement with the literature records [24]; for instance, an average 2500 of gold NPs coated with cetyltrimethylammonium bromide were deposited in epithelial cells, while PEG-modified gold NPs only had a few tens per cell [36]. In co-culture systems, unexpectedly, there is a slight increase of 100 nm particle number in cancer cells, despite a higher enhancement of NP internalization by macrophage. Comparing to the uptake index in the single-cell to mixed cell culture, it also reduces about 25% for 100 nm PLGA particles. The uptake indices were found to significantly decline with around 2.5 and 3 folds reduction in co-cultured system for 500 nm and 1 µm PLGA, respectively (Fig. 8i). Generally, it was found that the existence of macrophages largely affects the uptake ability of cancer cells especially for large particles, which is also in excellent agreement with the above observations.
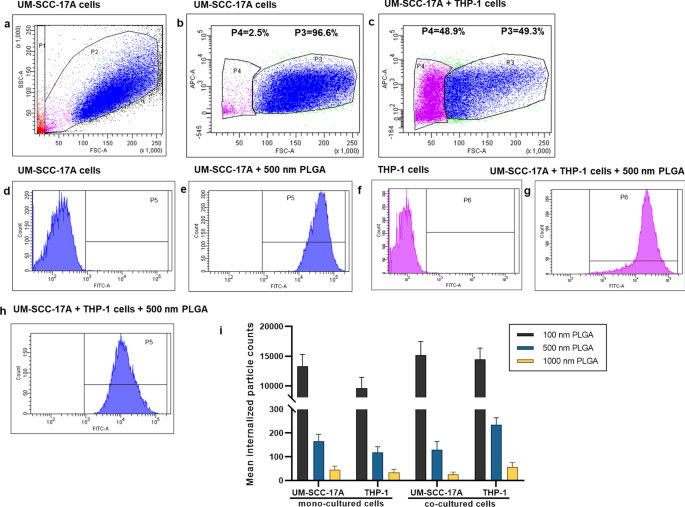
Particle uptake quantification in monoculture UM-SCC-17A cells and co-culture with THP-1 cells by flow cytometry. Grating strategy to identify respective cell populations in mixed cell culture (a –h )。 Gating is showing one representative experiment of cells exposure to 500 nm PLGA particles. With initial live gating in the y-axis with a side scatter (SSC-A) and x-axis with a forward scattering (FSC-A), P2 a were further gated with FSC-A versus APC-A to differentiate the THP-1 cells in P4 c from UM-SCC-17A cell population in P3 (monoculture cells (b ) and mixed cells (c )). The cell population of P3 further displayed as counts versus FITC plots (P5) in non-exposed cells (d ), monoculture cells (e ), and co-cultured cells (h )。 Also, P6 is the counts versus FITC-A plot originated from P4 population in non-exposed cells (g ) and co-cultured cells (f )。 Both types of cells efficiently ingested the 500 nm PLGA indicated by the solid histogram completed shifted to the right side of the x -axis, indicating particles are taken up by all exposed cells. Quantification of particle-laden numbers (i ) in both types of cells for mono-culture and co-culture systems
图>Currently, different uptake pathways by cancer cells in ingesting particles with different sizes and surface modifications like clathrin-mediated endocytosis, caveolae-mediated endocytosis, clathrin- and caveolae-independent endocytosis, micropinocytosis, and macropinocytosis have been claimed in the literature [37]. For instance, it has been proved that iron oxide aggregates with a size of < 200 nm are taken up by MCF-7 cells through the clathrin-mediated endocytosis, whereas larger aggregates tend to be ingested via macropinocytosis [38]. Another study has demonstrated that 100 nm plain polystyrene particles tended to be taken up mainly through macropinocytosis, whereas the internalization of carboxylated polystyrene particles prefer to occur via the clathrin-mediated endocytic route [25]. Phagocytosis is a classical uptake pathway for immune cells such as neutrophils, dendritic cells, and most importantly monocytes/macrophages. The uptake pathways tightly depend on various parameters of drug delivery vesicles, e.g. , the particle size, chemical composition, surface modification, proteins in the culture environment, as well as cell type. Usually, multiple uptake pathways can be involved in particle ingestion such as the caveolae-mediated endocytosis, clathrin-mediated endocytosis, and macropinocytosis, which has been found to participate in cellular internalization of 300–400 nm chitosan NPs in human HeLa cells [39]. It has also been observed that 63 nm cholesterol-modified pullulan NPs enter into the human hepatocellular carcinoma (HepG2) cells via macropinocytosis and clathrin-mediated endocytosis [40]. Several previous studies showed that PLGA particle cell uptake involves different endocytic pathways, in which clathrin-independent endocytosis is the main route responsible for the internalization of PLGA in in vitro models [23, 24, 37]. Nevertheless, once they entered into the cells, PLGA particles applied here were highly potentially entrapped by the endo-lysosomal system consisting of early endosomes, recycling endosomes, late endosomes, and lysosomes [41]. It should be noted, however, that there is a lack of studies showing the cellular uptake mechanisms in co- or tri-cultured systems in vitro, a notion that has to be probe in the future.
Induction of cell fusion by PLGA NPs
Extensive scientific evidence has shown that the fusion of monocytes/macrophages into multinucleated giant cells (MGCs) occurred in a broad range of biological processes [42, 43]. Generally, implantation of biomaterials into the body causes a foreign body response characterized by the fusion of macrophages into MGCs and fibrotic encapsulation [44]. A wide type of human and murine primary cells like alveolar macrophages, splenic macrophages, microglia, bone-marrow-derived macrophages, and blood monocytes, as well as cell lines such as RAW264.7 and UG3 were also frequently observed to form MGCs in vitro [26, 45]. It is well-known that the macrophages form a fusogenic phenotype when they are unable to ingest foreign materials via phagocytosis because of the large size of particles or implants. So far, it is unclear whether in vitro macrophage cell fusion relates to the particle size in the range 100–1000 nm, which can be easily phagocytized. Here, the cell fusions were observed in all groups by microscopic examination (Fig. 9). The cellular fusion was confirmed only when multiple nuclei were found to share the same cytoplasm in both fluorescence images and bright-field images. Spontaneous formation of giant cells by THP-1 cells was occurred in the control group without particle treatment (Fig. 9a), with a fusion percentage of about 10% of the total cells (Fig. 9h), as reported in the literature [46]. Size-dependent cellular fusion was found for 100 and 500 nm PLGA particles, whereas 1 µm PLGA-induced insignificant enhancement of fusions in monoculture. This may be due to the small number of 1 µm PLGA particles exposed to cells (approximately 60 particles exposed to each cell at a concentration of 10 µg/mL). When the macrophages were co-cultured with cancer cells, the percentages of MGC formation were increased in all groups in comparison with the corresponding monoculture groups. In particular, there is a significant increment of cellular fusion for 1 µm PLGA (19%) in the mixed cell culture. The most prominent increase in fusion occurred in co-cultured 500 nm PLGA particles, which might be attributed to the large size of particles and sufficient particle numbers ingested per cell.
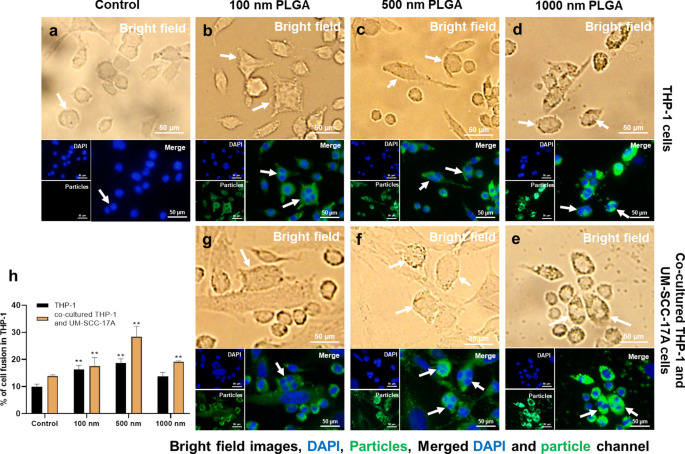
Visualization and quantification of THP-1 cell fusion in monoculture and co-culture systems. Cell fusion occurred in all groups including the control group without particle treatment for THP-1 cells (a )。 Monocultured (b , c ) 100 nm and 500 nm NP-induced significant cell fusion compared to 1 µm PLGA group (d ), which has a slightly higher level than that of the control group. In co-cultured systems, 100 nm (g ) and 1 µm (e ) PLGA had an identical percentage of cellular fusion, which is apparently smaller than that of 500 nm group (f )。 Quantification of cell fusion for all groups after 24 h incubation was displayed in h
图>The molecular machinery involved in macrophage fusion has been widely probed, achieving substantial progress [43]. For example, the formation of macrophage fusion receptors CD47and CD44 together allowed mediating the process of macrophage fusion, and subsequent the differentiation of giant cells [42] and miR-223 delivery by a NP vesicle permitted attenuating it [47]. Hence, further study should focus on the determination of key molecules in regulating particle-induced macrophage fusion and the dominant receptors expressed in the giant cell membrane. Another important concern is the fate of MGCs. It is believed that MGCs fused with particles or stimuli might subsequently experience the process of apoptosis or necrosis [48]. This may lead to the release of undigested particles or other giant materials to the other cells in vitro or biological tissues in vivo, further inducing the long-term inflammatory response or granulomas. On the other hand, the macrophage fusion itself may be benefit for NP drug delivery, for example, in tumorous tissues, the re-release of drugs from macrophages to the cancer cells might represent an enhanced tumor killing ability. More importantly, future nanomedicine study should address how to exploit the application of macrophage fusion for nanocarrier drug delivery to improved disease diagnosis and therapy. This is because not only macrophages an abundant type of immune cells in the body with the excellent uptake ability of nano-drugs, but also they may be used in targeted gene delivery for repair of injured tissues.
结论
To the best of our knowledge, this study first reported the competitive cellular uptake of PLGA particles with sizes ranging from 100 nm to 1 µm in co-cultured UM-SCC-17A laryngeal cancer cells and THP-1 monocytes/macrophages. The data collected here proved that immune cells may alter/lower the particle internalization by cancer cells in vitro, which is similar to the previous findings in in vivo nanocarrier drug delivery studies. Size-dependent and cell culture-related macrophage cellular fusion caused by PLGA particles has also been demonstrated here. Future studies should probe the uptake mechanism in co-cultured systems and design novel approaches to achieve a higher uptake index for laryngeal cancer cells in the presence of phagocytes. Moreover, elaborate evaluation of intracellular trafficking and fate of nano-drug-carriers in co- or tri-cultured systems in 3D, which better mimic the in vivo conditions, is needed prior to the utilization of animal studies in vivo and even in clinical trials.
数据和材料的可用性
本研究期间生成或分析的所有数据均包含在这篇已发表的文章 [及其补充信息文件] 中。
缩写
- PLGA:
-
Poly(lactic-co-glycolic acid)
- HNSCC:
-
Head and neck squamous cell carcinoma
- EPR:
-
增强渗透性和滞留性
- NP:
-
纳米粒子
- ICG:
-
Indocyanine green
- MPS:
-
Mononuclear phagocyte system
- LDH:
-
乳酸脱氢酶
- FACS:
-
Fluorescence-activated cell sorting
- MGC:
-
Multinucleated giant cells
- DLS:
-
动态光散射
纳米材料
- 用于增强药物递送的纳米纤维和细丝
- 用于灵敏快速检测卵巢癌细胞的柔性石墨烯生物传感器的演示
- 用于癌症治疗的纳米粒子:当前的进展和挑战
- 生物相容性 FePO4 纳米颗粒:药物递送、RNA 稳定和功能活性
- 用于癌症应用的基于细胞的药物递送
- 131I 追踪的 PLGA-脂质纳米颗粒作为靶向化疗治疗黑色素瘤的药物递送载体
- 以叶酸受体为靶点的生物类黄酮染料木素负载壳聚糖纳米颗粒可增强对宫颈癌的抗癌作用
- 基于叶酸和 gH625 肽的 Fe3O4 磁性纳米颗粒功能化增强细胞内化的比较
- 基于紫杉醇的靶向脂质纳米颗粒的抗增殖和细胞凋亡触发潜力,通过转铁蛋白受体增强细胞内化——白血病细胞研究
- Mg 掺杂对用于增强光催化评估和抗菌分析的 ZnO 纳米颗粒的影响
- 具有疏水修饰的普鲁兰多糖纳米颗粒的米托蒽醌新型递送抑制膀胱癌细胞以及纳米药物大小对抑制效率的影响
- 用于提高溶解度的蝌蚪形和球形血红素纳米颗粒的制备和表征