用 Rumex hymenosepalus 作为抗菌剂合成的 Au@Ag Core@Shell 纳米颗粒
摘要
在这项工作中,我们使用顺序合成方法合成具有核@壳结构的金银双金属纳米粒子(Au@AgNPs)。 Rumex hymenosepalus 含有高含量儿茶素和芪的根提取物 (Rh) 被用作纳米颗粒合成中的还原剂。通过透射电子显微镜 (TEM) 获得的尺寸分布,Au@AgNPs 的平均直径为 36 ± 11 nm,金纳米粒子 (AuNPs) 的平均直径为 24 ± 4 nm,银纳米粒子 (AgNPs) 的平均直径为 13 ± 3 nm。 NPs 的几何形状主要是准球形。 AuNP 上的银壳厚度约为 6 纳米,并被表面上的活性生物分子覆盖。纳米颗粒表征包括用扫描透射电子显微镜 (STEM)、能量色散 X 射线光谱 (EDS)、X 射线衍射 (XRD)、紫外-可见光谱、Zeta 电位记录的高角度环形暗场图像 (HAADF)、和动态光散射 (DLS)。傅里叶变换红外光谱仪 (FTIR) 和 X 射线光电子光谱仪 (XPS) 表明纳米颗粒被提取物分子稳定。使用 Gompertz 模型对暴露于纳米材料的微生物进行生长动力学研究。结果表明AgNPs和Au@AgNPs影响大肠杆菌的滞后期和生长速度 和白色念珠菌 呈剂量依赖性,对 Au@AgNPs 反应更好
介绍
在过去的 25 年中,已经研究了几种用于纳米材料合成的化学方法;然而,这些方法大多使用对环境不友好的物质,并使用高温或昂贵的设备。在这项工作中,我们使用绿色合成方法合成了金银纳米结构。这种方法从一开始就最大限度地减少了污染。在开发不会对健康或环境构成威胁的“清洁”纳米材料时,使用“清洁”工艺,避免大部分废物和有害污染物的使用。
金属纳米粒子的绿色合成寻求与生物系统相互作用的积极影响,这意味着纳米粒子及其与多酚分子的自我功能化产生与细胞和大分子等系统兼容的生物相互作用。一般来说,这些生物相互作用被用作治疗癌症、糖尿病和神经退行性疾病等疾病的纳米药物。银(壳)和 AuNps 作为核壳系统的合成具有诸如光学诊断传感器、分子传感器 [1, 2]、光热疗法、抗菌剂和改进催化过程 [3,4,5,6] 等应用。与单金属纳米颗粒。
特别是在不同反应器中的顺序或同时方法、化学和物理方法 [7] 用于双金属合成:超声喷雾热解 [8]、声化学方法 [5]、微流控芯片 [9]、顺序纳米流体纳米沉淀 [ 10, 11] 微乳[12]、脂质体[13]、还原剂为化学或绿色化学类型。
干材料在纳米粒子合成中用作金属氧化物 [14]、碳纳米管 [15、16],其他用途是印刷软电子设备 [17]。用于生物系统 [18,19,20] 药物载体 [21, 22]、抗菌剂 [23, 24]、传感应用 [25] 和计算纳米技术的湿纳米颗粒是用于定义用途的现代分类 [26]。
Khatami 等人回顾了使用植物核@壳型纳米粒子的绿色合成,特别是使用了Antigonon leptopus , 柿子椒 , 印楝 , Potamogeton pectinatus , Anacadium oceidentale 用于合成尺寸在 5 到 500 nm 之间的 Au@Ag 纳米粒子 (a),针对不同应用(非抗菌)和总状芦笋进行了测试 根提取物用于合成 Au-Ag 合金纳米颗粒,在 大肠杆菌 中测试的 MIC 为 480 µg/mL , 枯草芽孢杆菌 , 肺炎克雷伯菌 , 铜绿假单胞菌 , 和金黄色葡萄球菌 [27]。通过化学合成获得的 Au@Ag NPs 具有抗菌作用,据报道,银含量(壳)的 MIC 约为 2.5 µg/mL [28],Lu 等。阿尔。报道了化学合成 Au/AgNPs@Van 是使用 NaBH4 制备的,并添加万古霉素,对于在革兰氏阳性和阴性细菌中测试的纳米颗粒双金属,MIC 为 60 nmol/mL [29]。
双金属纳米材料的抗菌性能 [30,31,32,33,34] 作为 Ag 浓度的函数而提高。相反,金浓度的增加会降低抗菌性能,但会降低细胞毒性作用,即双金属材料变得更具生物相容性 [35]。比较 Au 和 Ag 单金属材料与双金属材料的效果 [36,37,38,39,40],已经证明双金属材料之间发生协同效应 [41],产生双功能效应 [28, 42]。
纳米材料的功能化特别令人感兴趣,因为系统周围的化学环境 [43,44,45](pH、硫的存在、生物相容性分子等)会对与细胞或微生物的相互作用产生影响;因此,强调利用绿色化学制备生物材料[46,47,48,49,50,51,52,53,54,55]。
由于单金属纳米结构的协同作用[60],双金属颗粒的化学成分[56,57,58]将成为其光学性能的决定因素[41, 59]。
在这项工作中,使用 Rumex hymenosepalus 作为还原剂合成了金和银纳米粒子 提取物,这是一种植物,含有二苯乙烯和儿茶素分子,可作为强大的抗氧化剂,减少金属离子。以金纳米粒子为核,通过顺序合成方法获得双金属纳米粒子核@壳型Au@Ag。纳米材料的表征涉及HAADF-STEM、TEM和EDS y HRTEM技术。
使用不同类型的合成纳米颗粒,对细菌E 的生长动力学进行了比较研究。大肠杆菌 和S。金黄色葡萄球菌 和酵母白色念珠菌。
实验部分
材料
Rumex hymenosepalus 的根 是在当地的一家商业机构中收购的。用于纳米颗粒合成的前体 HAuCl4 和 AgNO3 均购自 Sigma-Aldrich,纯度为 99%。用于微生物检测的脑心浸液 (BHI) 肉汤和马铃薯葡萄糖肉汤 (PDB) 购自 Sigma-Aldrich。清洗纳米颗粒工艺所用乙醇(纯度99%)购自Fermont,实验使用超纯水(milli-Q)。
Rumex hymenosepalus Extract
对于提取物制备,将 150 g 切成薄片并预先脱水的根浸渍在 1000 mL 的 70/30 V/V 乙醇/水混合物中。浸渍在室温下在带有密封塞并避光的玻璃容器中进行。监测所得溶液的吸光度 21 天,直到其值没有变化。此时,浸渍过程被认为完成。获得的提取物依次使用孔径为 8 µm 和 2 µm 的 Whatman 纸过滤器进行过滤,最后使用 0.22 µm 的 acrodisc 过滤器进行过滤。通过旋转蒸发除去乙醇,并将含水提取物浓缩物在 -80°C 下冷冻用于冻干(Labconco,FreeZone 1L)。所得粉剂保存于无菌容器中,室温避光保存备用。
AuNPs 的合成
首先,Rumex hymenosepalus 由冻干提取物制备 10 mg/mL 的水溶液。后来 16 毫升 Rumex 将溶液与 32 mL 超纯水混合,保持 1000 rpm 搅拌,然后缓慢加入 16 mL HAuCl4 (0.01 M)。反应在实验室光照条件和室温下保持一小时。表面等离子体共振强度(λ SPR =540 nm) 是通过 UV-Vis 光谱随时间评估的;如果没有观察到变化,则认为合成完成。所得产物以 12,000 rpm 离心,上清液更换为超纯水,超声处理 1 小时以重新分散纳米颗粒。该过程再重复三遍。前两次使用水作为溶剂,最后使用乙醇作为溶剂。最后,将乙醇分散液离心,沉淀物在对流烘箱中在 40°C 下干燥,以制备 2300 µg/mL 的 AuNPs 水分散液。
Au@AgNPs 的合成
对于 Au@AgNPs 合成,2 mL AuNPs 水分散体 (2300 µg/mL)、0.8 mL AgNO3 (0.1 M) 和 0.8 mL Rumex hymenosepalus 溶液 (10 mg/mL) 沉积在无菌玻璃培养管中。将混合物在超声波清洁器浴(Branson,2510 型)中超声处理 3 小时。之后,内容物以 12,000 rpm 离心 1 小时,所得固体经超声处理再分散于超纯水中,浓度为 1000 µg/mL。
AgNPs 的合成
为了生产 AgNP,将 16 mL 提取液 (10 mg/mL) 与 64 mL 超纯水和 8 mL AgNO3 0.1 M 混合。在实验室照明条件下,在 25 °C 下进行反应一小时,然后表面等离子体共振 (\(\lambda_{{{\text{SPR}}}}^{{{\text{Ag}}}}\) =440 nm) 通过紫外-可见光谱随时间监测以评估形成。产物以 12,000 rpm 离心,上清液更换为超纯水,超声处理 1 小时。该过程再重复三遍。前两次使用水作为溶剂,最后使用乙醇作为溶剂。将乙醇分散体离心并将沉淀物在对流烘箱中在 40°C 下干燥。最后,通过超声将获得的 AgNPs 粉尘重新分散在超纯水中,以产生 2000 µg/mL 浓度的胶体分散体。
特征
UV-Vis 吸收光谱是在双光束 PerkinElmer Lambda 45 光谱仪上获得的。采用 0.5 nm 的狭缝,以 480 nm/min 的速度记录 200 至 900 nm 范围内的光谱。对于纳米颗粒,使用 50 µL 样品,仅使用 5 µL 提取物。使用超纯水作为溶剂在石英池中定容至 3 mL。
Zeta 电位 (ζ ) 的 AuNP、AgNP 和 Au@AgNP 使用 Zetasizer-Nano ZS(英国马尔文仪器公司)进行测量。每个样品在室温 (25 °C) 下重复测量三次,并作为每个样品浓度为 1、10、50 和 100 µg/mL 的函数。
使用配备 633 nm He-Ne 激光器的 Zetasizer-Nano ZS(英国马尔文仪器公司)测量 AuNP、AgNP 和 Au@AgNP 的 DLS。每个样品在室温 (25°C) 下重复测量三次,并作为每个样品浓度为 1、10、50 和 100 µg/mL 的函数。多分散指数 (PDI) 由 DLS 实验使用 Malvern 软件通过定义确定 \({\text{PDI}} =\left( {\frac{\sigma }{{\overline{D}}}} \right)^ {2}\),其中 D 是平均直径,\(\sigma\) 是\(D\) 的标准偏差。 PDI值小于等于0.10被认为是高度单分散的[62]。
光学带隙E 计算 AgNPs、AuNPs、Ag@AuNPs 的 g,使用 Tauc 方程通过以下关系确定材料中的带隙:
$$\alpha =\frac{c}{h\upsilon }\left( {h\upsilon - E_{{\text{g}}} } \right)^{1/n}$$ (1)其中\(\alpha\)是材料的吸收系数(\(\alpha\) =2.303 A /d 其中 A 是吸光度,d 是细胞的宽度),其中 E g 是光能带隙,hʋ 是通过在 (αhʋ ) n 和光子能量hʋ .对于样本中允许的直接带间转换,指数 n 取为 2 [63],并且可以很容易地用线性拟合图进行评估 [64]。取决于转换的类型,其值 1/2、2、3/2 和 3 分别对应于允许的直接、允许的间接、禁止的直接和禁止的间接转换 [65]。
使用 FTIR(PerkinElmer, Inc., Waltham, Spectrum Two)通过红外吸收分析 AgNPs、AuNPs、Ag@AuNPs 和 Rh 样品。采集参数为:4 cm −1 分辨率,16 次扫描,在 4000 到 900 厘米之间 −1 波长范围。
X 射线光电子能谱分析在 PerkinElmer 型号 PHI 5100 上进行,该型号包含 Mg/Al 双源,300 W,15 kV。能量为 1253.6 eV 的 Mg Kα 发射线用于 AgNPs、AuNPs、Ag@AuNPs 和 Rh。所有实验均在2 × 10 –9 的真空条件下进行 托。 [66]。使用 Multipak 软件分析数据。对于 XPS 表征,不同的纳米颗粒分散体和 Rumex hymenosepalus 提取溶液如下沉积在干净的盖玻片上。将 30 µL 样品添加到盖玻片中,使其完全干燥以备下一次沉积。该过程至少重复5次,直到形成薄膜,然后通过XPS表征。
高角度环形暗场扫描透射电子显微镜(HAADF-STEM)可以被认为是电子显微镜中一种强大的操作模式,它提供了大量的补充信息来阐明纳米材料的结构。像差校正的 HAADF-STEM 可以用原子分辨率确定不同化学性质的原子的位置。这是由于像差校正的 HAADF-STEM,以其化学敏感性和高空间分辨率而闻名 [67]。在这种操作模式下,非相干图像占主导地位,衍射对比度的贡献可以忽略不计[68]。因此,HAADF-STEM像差校正中的原子序数对比(Z对比)使我们能够非常精确地确定纳米结构的结构细节。
对于 STEM 分析,在 JEOL-JEMARM200 电子显微镜中对样品进行分析,该显微镜在 200 kV 下运行,聚光镜配有 CEOS 校正器。 Z-Contrast STEM 图像在 BF 和 HAADF 模式下同时记录。使用 40 微米聚光镜孔径(32-36 mrad 会聚角)和 9 pA 光斑尺寸记录图像。
对于电子显微镜分析,将一滴 (10 µL) Au@AgNPs 悬浮液沉积在 300 目厚的碳网格上,干燥至室温,然后放置在真空室中 24 小时。
在 Jeol 2010F 装置(1.9 埃分辨率)中通过 TEM 在 200 kV 下分析纳米颗粒。 EDS 分析是使用带有 XFlash 4010 检测器的 QUANTAX 200-TEM X 射线光谱仪 (Bruker) 实现的。对于 HRTEM 分析,在大于 100,000X 的放大倍数下记录 TEM 显微照片。晶面的晶面间距通过数字显微照片分析(3.0 Gatan 版)确定。样品制备与上述 STEM 分析相似。
使用配备多层反射镜单色器和 Cu Kα 微聚焦密封管 (λ) 的 Bruker D8 QUEST 衍射仪系统收集数据 =1.54178 Å)。在 T 收集帧 =300 K 通过 ω /φ - 扫描,然后处理以获得强度与 2Theta 的衍射图。采用High Score Plus软件对原始数据进行处理,利用软件关联的ICSD粉末衍射数据库进行搜索匹配相识别分析。
抗菌活性测定
测试的微生物是细菌大肠杆菌 (ATCC 25922), 金黄色葡萄球菌 (ATCC 5538P) 和酵母白色念珠菌 从一名成年男性尿路感染患者收集的受感染尿液中分离出来(我们的研究遵循赫尔辛基宣言的原则)。脑心浸液(BHI)和马铃薯葡萄糖肉汤(PDB)分别用于制备细菌和酵母菌的接种物。培养物在 37 ºC 下孵育过夜。每毫升悬浮液中的菌落形成单位 (CFU/mL) 浓度是通过在 540 nm 处测量细菌的 UV-Vis 光密度来确定的,酵母在 600 nm 处测量。含有4 × 10 8 的悬浮液 , 7.8 × 10 8 , 和 2.5 × 10 6 CFU/mL用于E。大肠杆菌 ,S。金黄色葡萄球菌 , 和 C.白化病 , 分别。抗菌活性在 96 孔板中进行测试,在 37 ºC 下使用添加了纳米颗粒和微生物的液体培养基。所有测试均一式三份进行。使用 Gen 5 软件在多模式读板机 Synergy HTX Biotek 中测量吸光度。所有微生物生长曲线均使用 Origin Lab 8.0 软件进行。作为第一步,将 70 µL 的新鲜肉汤培养基(BHI 或 PDB,根据所研究的微生物)与所需浓度的纳米颗粒混合到孔中。随后,将 30 µL 微生物悬浮液加入孔中并用培养基匀浆。每个孔的最终体积为 100 µL。分配接种物后,在上述分光光度计中读取 96 孔板。将板在 37°C 下保持 24 小时,在每次读数前采用圆形振动模式,每次读数为 15 分钟。在上述波长下通过光密度测量(OD)测定微生物的生长速度。
Gompertz 模型分析的曲线增长
在文献中众所周知,Gompertz 生长模型很好地描述了微生物种群的生长。该模型使我们能够理解描述微生物种群生长的两个关键参数:适应期(滞后期)和种群增长率。特别是,研究滞后期在微生物抑制处理中的行为是相关的,因为它提供了微生物对处理的适应性反应的信息,甚至可以指示微生物对评估处理的抗性的发展[69 ].
Zwietering 等人已经描述了改进的 Gompertz 模型。 [70] 并由 Li 等人改编。 [69] 和其他作者 [71,72,73,74,75,76,77,78] 作为调整生长曲线和
$$y =A\exp \left\{ { - \exp \left[ {\frac{\mu e}{A}\left( {\lambda - t} \right) + 1} \right]} \right \}$$ (2)其中 A 是表示为 OD540 (金黄色葡萄球菌 和E。大肠杆菌 ) 和 OD600 (白色念珠菌 ), μ 是指数阶段的增长率,e 是指数 e 1 , \(\lambda\) 是滞后阶段。我们已经使用软件起源 9.1 调整了我们的增长动力,以分析对 S 的影响。金黄色葡萄球菌 ,E。大肠杆菌, 和C。白化病 AuNPs、AgNPs 和 Au@AgNPs 三种试剂。
纳米粒子对 E. 的影响。大肠杆菌, ,S。金黄色葡萄球菌 , 和 C.白化病 成长
大肠杆菌 和金黄色葡萄球菌 在BHI培养基中接种,并且C.白化病 接种于 PD 肉汤中。所有培养物在 36°C 下孵育过夜。孵育后,将三种培养物分别调整为吸光度为 1、0.7 和 1(λ =540 纳米)。 AuNPs、AgNPs 和 Au@AgNPs 被调整到 50 µg/mL 的浓度。在 96 微孔板中,调整后的培养物以 7:3 的比例与纳米颗粒接触,每个微孔的最终体积达到 200 µL。此外,在相同的先前描述的条件下,将调整后的培养物暴露于无菌水作为对照条件。用于该实验的其他对照是新鲜培养基和不含微生物的每种纳米颗粒溶液。 大肠杆菌、金黄色葡萄球菌 , 和 白色念珠菌 分别暴露于 AuNPs、AgNPs 和 Au@AgNPs。微孔板在 36°C 下孵育,并在 1、7、13 和 19 小时获得处理和对照条件下的每种微生物的样品。收集的样品在连续的 1:10 盐水溶液中稀释并铺在 Müeller-Hinton 板表面。接种的板在 36°C 下孵育 24 小时。培养后直接计数法计算CFU/mL。
最低杀菌浓度 (MBC) 测定
金黄色葡萄球菌 , 大肠杆菌 , 和 白色念珠菌 将每个接种到 Müeller-Hinton 肉汤中,在 36°C 下孵育过夜并调整至 0.5 McFarland Nephelometer。一旦针对最低抑菌浓度 (MIC) 测定调整接种物,就进行微量稀释测试。简而言之,为此目的使用了 96 孔板。将 160 µL 每种微生物的调整培养物倒入 5 个孔中。制备了 1000 µg/mL 的 AuNP、AgNP 和 Au@AgNP 溶液。以前的溶液用于制备 750、500 和 250 µg/mL 溶液。使用纯无菌水作为 0 µg/mL 的纳米颗粒,并将 40 µL 的每种先前溶液添加到 160 µL 的调整培养物中。以这种方式,每种培养物都暴露于终浓度为 200、150、100、50 和 0 µg/mL 的每种测试纳米颗粒。微孔板在 36°C 下孵育 24 小时。之后,将每个孔的样品接种在 Müeller-Hinton 的表面上,并在与先前描述的相同条件下孵育。孵育后,观察 Müeller-Hinton 板寻找任何生长信号。未观察到生长信号的浓度记录为MBC值。
结果和讨论
特征化
图 1a 显示了合成纳米材料的 UV-vis 吸收光谱。吸光度已针对对应于每个纳米粒子系统的最大局域表面等离子体共振(LSPR)进行归一化。
<图片>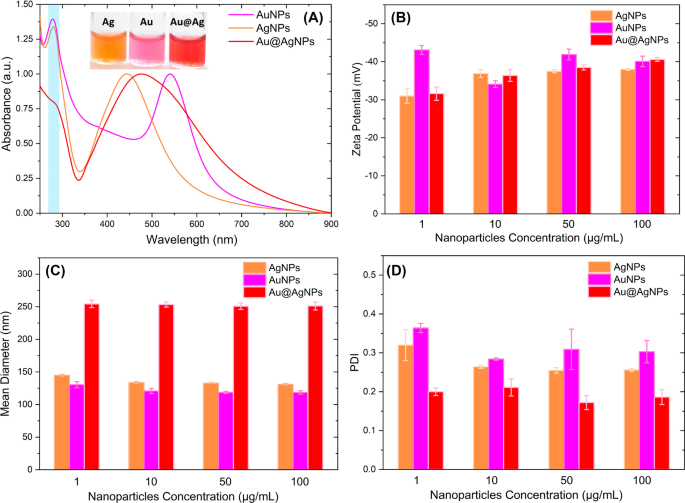
纳米粒子 UV-Vis 光谱 (a ), Z-电位 (b ), DLS (c ) 和 PDI (d ) 的 Au、Ag 和 Au@AgNPs
图>图 1a 中的 Au@AgNps 吸收光谱有一个以 474 nm 为中心的单波段,它位于 AgNPs LSPR(445 nm)和 AuNPs LSPR(544 nm)之间。 Au@AgNPs 上没有类似金的吸收峰表明通过顺序合成获得的纳米材料是核@壳结构。不可能检测到任何与 Au 相关的吸收带属于原子核。一些作者假设对于核@壳系统,吸收光谱由与每种金属相关的两个带组成,壳厚度在 3 到 4 纳米之间。与金属核相关的吸收随着厚度的增加而消失,获得单个吸收带,其中最大位置取决于双金属颗粒的厚度/核尺寸比[79, 80]。
萨马尔等人。 [81] 通过控制核尺寸和添加不同厚度的壳,合成了核@壳纳米粒子(Au@Ag)。特别是,我们对 Au@AgNPs 的 UV-vis 结果与 Samal 等人报道的结果一致。对于 32 nm 金核和大于 15 nm 的银厚度,其中光谱的特征在于单个吸收带(~ 450 nm),并且观察到 Au 表面等离子体的抑制。
此外,在图 1a 中,可以观察到以 280 nm 为中心的吸收(蓝色突出显示的区域),对应于来自 Rumex hymenosepalus 的分子 提取物在我们的纳米颗粒合成中用作还原剂。附加文件 1:图 S1 对应于 Rumex hymenosepalus 水溶液吸收光谱。观察到以 278 nm 为中心的特征带,与与多酚化合物的羰基共轭的芳环的电子跃迁有关 [82]。纳米粒子 UV-Vis 光谱中的这个吸收带表明最终产品含有残留在其中的提取物分子,Rivero-Cruz 等。阿尔。已经报道了从 R 中分离出的四种芪类、两种黄烷-3-醇和三种蒽醌。处女膜 [83] 和 Rodríguez-León 等。阿尔。已进行核磁共振研究以确定 Rumex hymenosepalus 含有重要分子如芪糖苷和表儿茶素没食子酸酯和表没食子儿茶素没食子酸酯 [61]。这些分子作为还原剂参与纳米颗粒合成。该过程涉及酚环的一些-OH基团的去质子化以形成 =CO基团。多酚分子被氧化,释放的电子转移到Ag + 和 Ag 3+ 离子形成 Au 0 和 Ag 0 .
图 1b 显示了与浓度为 1、10、50 和 100 µg/mL 的 AuNP、AgNP 和 Au@AgNP 对应的 Zeta 电位值。纳米颗粒分散在超纯水中,在25℃下重复三次测量。一般而言,在整个浓度范围内,纳米粒子的 zeta 电位值比 -30 mV 更负,AuNPs 和 Au@AgNPs 在浓度为 100 µg/mL 时达到 -40 mV,AgNPs 达到 -38 mV。这些高度负的 Zeta 电位值表明纳米粒子之间会发生排斥相互作用,从而阻止它们聚集并允许金属胶体长期稳定 [84,85,86]。通过将 UV-Vis 光谱结果与获得的 Zeta 电位值相关联,我们可以确定高度负值可能是由于提取物的多酚分子复合到纳米颗粒表面 [87]。
对于生物应用,获得具有单分散尺寸的纳米粒子群是有价值的 [88],因此图 1c 和 d 显示了对应于 AuNP、AgNP 和 Au@AgNP 浓度的平均直径和多分散指数 (PDI) 的 DLS 值1、10、50 和 100 µg/mL。 Au@AgNPs 的平均直径约为 250 nm,并且作为浓度的函数保持恒定,对于单金属纳米粒子,AuNPs 和 AgNPs 的平均直径分别约为 122 和 135 nm,以类似的方式。在同一情况下,观察到单金属纳米粒子的 PDI 值约为 0.3,Au@AgNPs 的值约为 0.2。这些结果表明,不同的纳米颗粒体系具有良好的稳定性,大小的多分散性适中(0.3 ≥ PDI ≥ 0.2)。
计算了 Tauc 图的光学带隙(附加文件 1:图 S2)。半导体材料的导带值E g <3 eV,作为参考锗有 E g <0.7 eV,硅是 E g =1.1 eV [89]。在我们的案例中,Au@AgNPs、AuNPs 和 AgNPs 的带隙分别为 1.93 eV、2.03 eV 和 2.33 eV。这意味着这些材料被认为是半导体,而这一特性是通过产生增加能隙的量子限制效应获得的,因此纳米材料可以用作传感器、电池和光电器件[64]。
为了确定纳米颗粒中是否存在有机化合物,这些纳米材料通过 XPS 和 FTIR 进行了表征。图 2a 显示了植物提取物和纳米颗粒的调查光谱。可以看出,所有纳米颗粒系统都呈现出与来自 Rumex hymenosepalus 的元素碳(C 1 s,284.6 eV)和氧(O 1 s,532.2 eV)相关的特征信号 提炼。该结果证实了来自提取物的有机分子存在于获得的纳米材料中。此外,还获得了高分辨率 XPS 光谱以分析纳米颗粒中银和金的氧化态(附加文件 1:图 S3)。对于 AgNPs(附加文件 1:图 S3A),对应于 3d3/2 和 3d5/2 谱线的 373.76 eV 和 367.76 eV(ΔBE =6.0 eV)的信号可以与 Ag 0 相关联 (金属银)。对于 AuNP(附加文件 1:图 S3B),与 4f5/2 自旋轨道耦合相关的峰值位于结合能 87.65 eV。对于 4f7/2,自旋轨道耦合峰位于 83.98 eV。强度比 (I4f7/2> I4f5/2)、位置和峰之间的间隔 (ΔBE =3.67 eV) 证实金离子 (Au 3+ ) 完全还原为金属金 Au 0 [90]。对于 Au@AgNPs,对应于银和金的高分辨率 XPS 光谱分别显示在附加文件 1:图 S3C 和图 S3-D 中。这些光谱显示出与其单金属对应物相同的行为。这表明在 core@shell 演示中,银和金都是零价的。
<图片>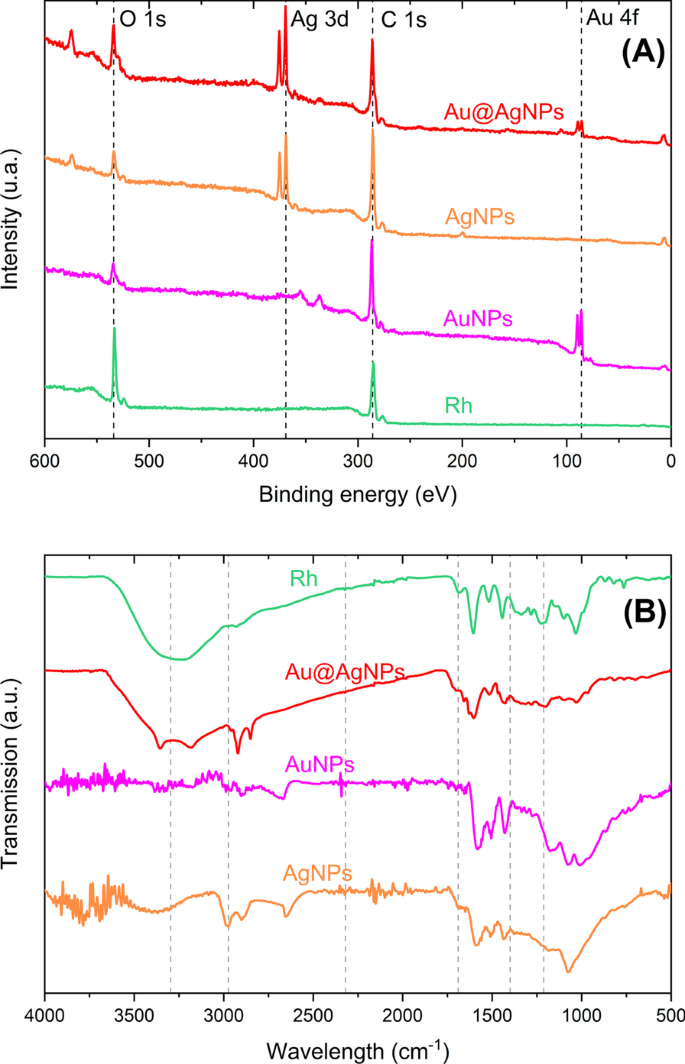
XPS 调查光谱 (a ) 和 FTIR (b ) 对于 Rh、Ag、Au 和 Au@AgNPs
图>图 2e 中的 FTIR 显示在 3296 cm -1 羟基,2974 cm -1 (C–H) 芳香环的结合,1689 cm -1 与羰基拉伸 (C=O) 和 1689–1400 cm -1 相关 由于羧酸盐键 (C-O) 和拉伸碳-碳键和 1212 cm -1 与 AuNPs 共轭的苯酚 C-O 拉伸相关,1049 cm -1 儿茶素酯基分子的 C-O 拉伸模式 [88,89,90] 和 799 cm -1 苯酚中平面的弯曲,C-H 弯曲报告为 964.4 和 829.39 cm -1 白藜芦醇的特征 [91] 在 Rh 提取物中转移到 1031 和 867 cm −1 ,AuNPs、AgNPs和Au@AgNPs也发生位移,表明Rh提取物的分子多酚与纳米颗粒发生络合。
图 3a 对应于 Au@AgNPs 系统在低放大倍数(100 nm 比例尺)下的代表性明场 STEM 显微照片。可以看到一组没有团聚并具有大部分准球形几何形状的纳米粒子。 The same region is shown in dark field (HAADF) in Fig. 3b, and the core@shell structure can be observed, where can we distinguish Au-core looks more intense than Ag-shell, due to the difference in atomic number. Figure 3c and d corresponds to STEM higher magnification micrograph (scale bare 20 nm) of a nanoparticles group of system core@shell in a bright and dark field, respectively. Can be appreciated with clarity brilliant Au core and Ag shell lightly contrasted. These images show that the thickness of the Ag-shell varies between 3 and 5 nm. Additional file 1:Figure S4 corresponds to an individual images gallery where can be observed uniformity of Ag-shell.
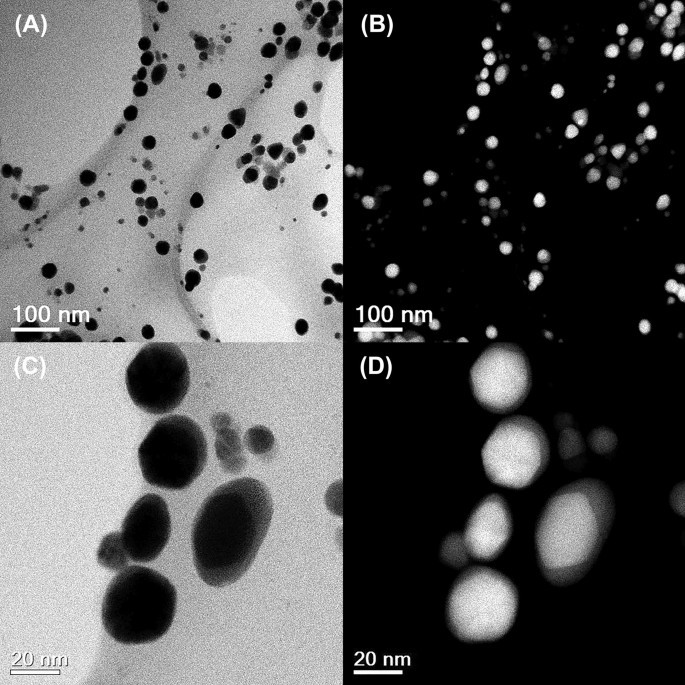
Scanning Transmission Electron Microscopy at low magnification (scale bar 100 nm) of Au@AgNPs in Bright Field (a ) and HAADF (b )。 A Small group of Au@AgNPs at higher magnification (scale bar 20 nm) in Bright Field (c ) and HAADF (d )
图>Figure 4a, c, and e corresponds to micrograph TEM of representative nanoparticles systems AuNPs, AgNPs, and Au@AgNPs, respectively. In all cases, nanoparticles have sphere-like morphology and are shown well separated from each other. This can be explained by the extract molecules onto nanoparticle surfaces, acting as spacers between them. Figure 4b, d, and f shows histograms corresponding to size distribution obtained by TEM and performed with 500 nanoparticles collected from 15 to 20 micrographs for each nanomaterial. The histogram presents Gaussian distribution with a mean size of 24 ± 4 nm (AuNPs), 13 ± 3 nm (AgNPs), and 36 ± 11 nm (Au@AgNPs). The discrepancy in values between DLS and TEM measurements with size distribution graph is due to conditions micro-environmental around the nanoparticles, while DLS shows a diameter for a system that includes metal, hydrated coating, and solvent by comparison in TEM measurements is a dry system where measurement is over metal only, in particular, in DLS the molecules used for complexation of nanoparticles (reducing agents and stabilizers) are dispersants that induce errors in sizing measurement and shifts it results to higher values [91].
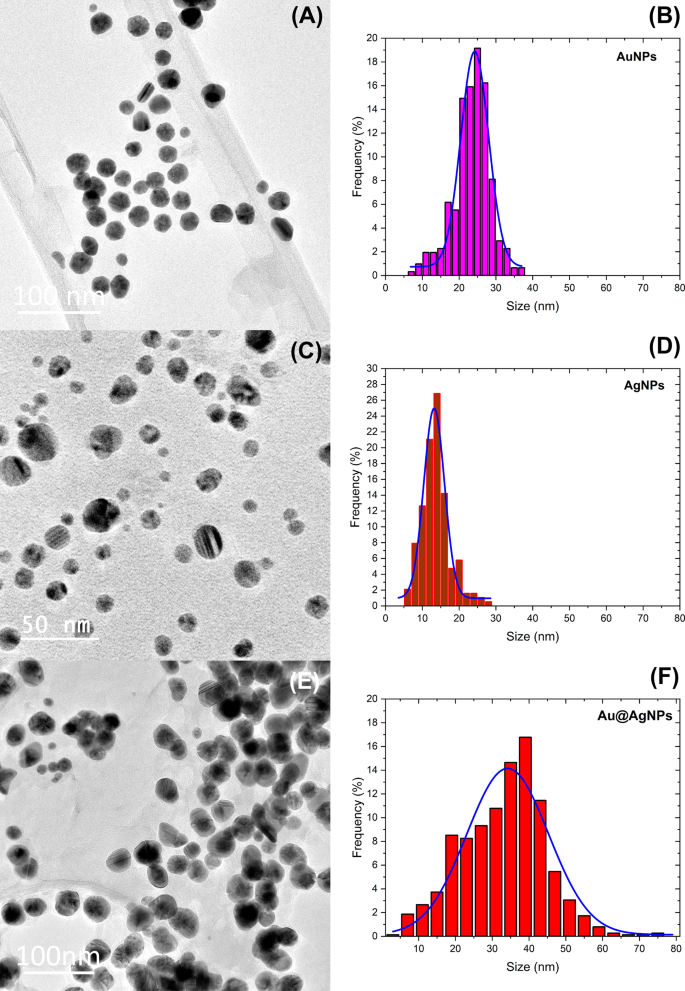
TEM and size distribution of AuNPs in (a ) 和 (b ), AgNPs in (c ) 和 (d ), and Au@AgNPs in (e ) 和 (f )
图>Figure 5 corresponds to the Au@AgNPs HAADF-STEM micrographics. A single nanoparticle is shown in Fig. 5a with a gold nucleus and silver cover perfectly delimited, the atomic number (Z) changes through the interface Au@Ag, intensity variations can be quantified by HAADF-STEM [67].
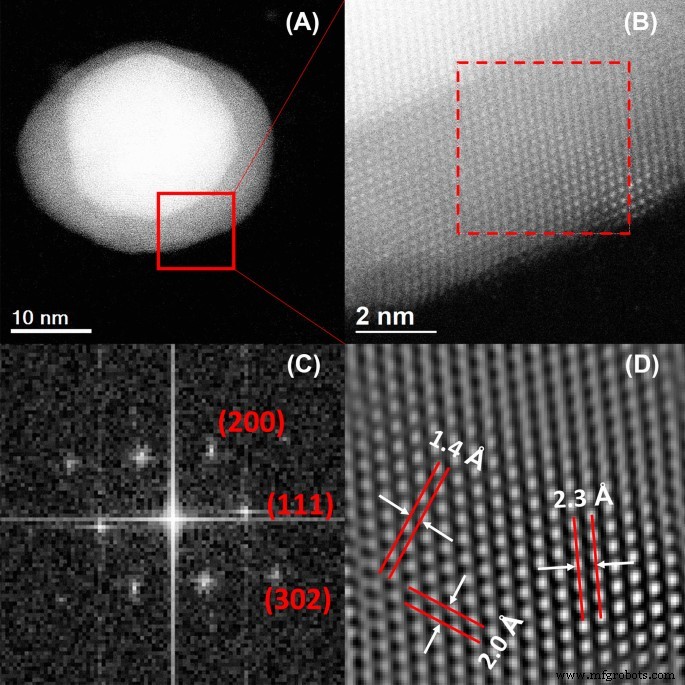
Au@AgNPs HRTEM (a )。 Magnification from (a ) of interface core–shell (b )。 FFT plot with Miller index (c ) and integrated image from FFT (Inverse) with interplanar distance (d )
图>The red square region is amplified to obtain an HRTEM micrography of the shell portion (Fig. 5b), and then to verify the crystalline shell structure, the nanoparticle periphery region was analyzed (discontinued square) with the Digital Micrograph 3.0 software (Gatan). Fast Fourier Transform (FFT) image of the selected area was obtained (Fig. 5c). Using the Inverse Fast Fourier Transform was possible to estimate interplanar distances of 2.3 Å, 2.0 Å, and 1.4 Å in Fig. 5d. These distances can be assigned, respectively, to the crystalline planes (111), (200), and (220) of face-centered cubic (fcc) silver according to Inorganic Crystal Structure Database (ICSD) at the FIZ Karlsruhe–Leibniz Institute for Information Infrastructure, Germany or the electron crystallography software Jems (V 4-5430, JEMS-SAAS, Switzerland) [92]. A similar analysis of crystal structure by HRTEM was carried out for monometallic nanoparticles as illustrated in Additional file 1:Figures S5 (for AuNPs) and S6 (AgNPs). In both cases, crystal structure corresponds to face-centered cubic (fcc).
EDS chemical analysis shows the presence of both metals for a group of bimetallic Au@AgNPs observed by TEM (Fig. 6a) in proportions of the atomic weight percent 77% of Ag (shell) and 23% of Au (cores) (Fig. 6b). In comparison, a single bimetallic (Fig. 6c) Au@AgNPs has proportions around 80% of Ag (shell) and 20% of Au (core) (Fig. 6d). To estimate the gold and silver atomic percentage on core@shell nanoparticles (Au@Ag), a quasi-spherical geometry approximation of nanoparticles morphology was considered. The AuNPs average diameter obtained from TEM size distribution (\(\overline{D}_{{{\text{Au}}}} =24\;{\text{nm}}\)) was used for core volume estimation (V Au), and Au@AgNPs average diameter \(\left( {\overline{D}_{{{\text{Au}}@{\text{Ag}}}} =36\,{\text{nm}}} \right)\) for core@shell volume estimation \((V_{{{\text{Au}}@{\text{Ag}}}} )\). So, shell volume is determined as \(V_{{{\text{Ag}}}} =V_{{{\text{Au}}@{\text{Ag}}}} - V_{{{\text{Au}}}}\). Total atomic content of Au and Ag was calculated considering an fcc crystalline structure (4 atoms per unit cell) for booth metals where the Au and Ag lattice parameters are 4.0783 Å and 4.0862 Å, respectively [93]. Atomic content estimation by this procedure is 70% for Ag (Shell) and 30% Au (Core), which differs by 10% concerning the measurement obtained by EDS. Figure 7 shows a theoretical estimation of silver and gold content and how varies as the thickness of the shell increases and the size of the core is kept constant (\(\overline{D}_{{{\text{Au}}}} =24\;{\text{nm}}\)). It is observed that for Au@AgNPs with a diameter greater than 30.2 nm, the atomic content of silver exceeds the content of gold. In Supplementary Material, a detailed description of calculation is carried out to obtain atomic contents percentage. Similar estimations of atomic percentages were carried out considering other Au-core diameters and varying Au@AgNPs diameter (Additional file 1:Figure S7A-B).
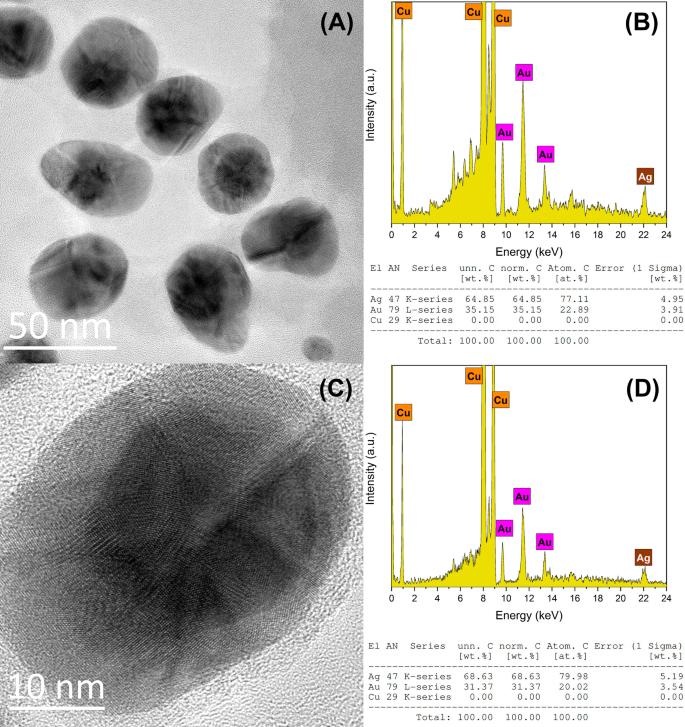
TEM and EDS of an Au@AgNPs group (a , b ) with Au 23 and Ag 77% at. and single Au@AgNPs (c , d ) with Au 20 and Ag 80% at
图>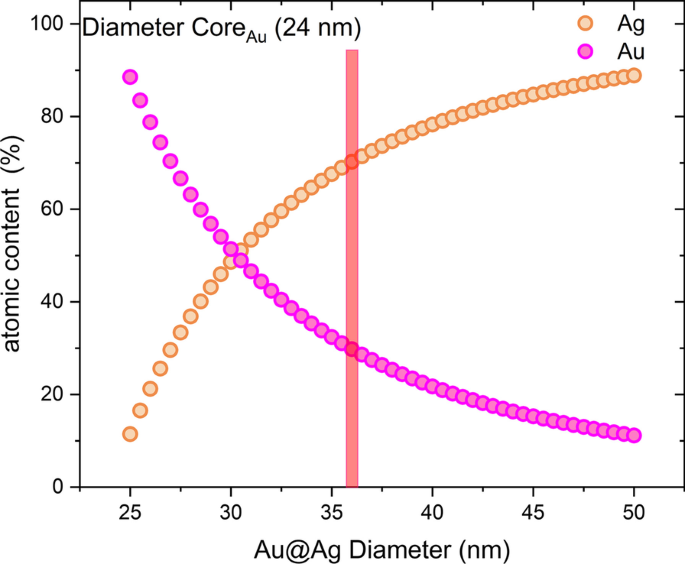
Variations of atomic content percentage versus increase thickness shell, where diameter AuNPs (24 nm) was kept constant
图>Figure 8 corresponds to XRD patterns for AuNPs and AgNPs as well as bimetallic Au@AgNPs. All the synthesized products have fcc crystalline structure as previously reported in the characterization by electron microscopy. Peaks for Au@AgNPs are located at 2θ diffraction angles of 38.25°, 44.4°, 64.9°, 77.85°, and 81.25°. As can be appreciated in the figure, the AgNPs and AuNPs diffraction peaks are found in the same positions mentioned with a difference of ± 0.5°. This is because Au and Ag have very similar lattice constants, so their diffraction patterns for fcc crystal structure are almost identical [94,95,96]. In this way, the diffraction peaks in Fig. 8 are assigned, respectively, to the crystalline planes (111), (200), (220), (311), and (222) of the gold and silver fcc structure by JCPDS:4-0783 and 4-0784 [97].
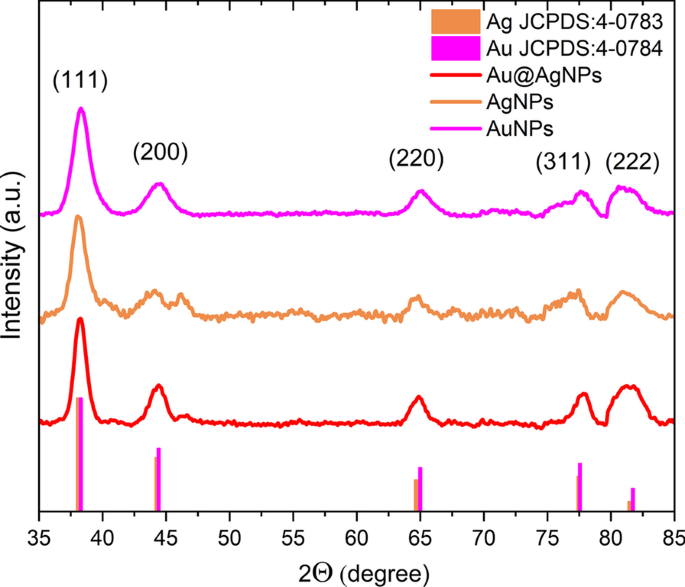
X-ray Diffraction AuNPs (pink), AgNPs (brown), and Au@AgNPs (red)
图>Antimicrobial Activity
Monometallic (AgNPs, AuNPs) and bimetallic (Au@AgNPs) materials were tested at four different concentrations:1, 10, 50, and 100 μg/mL. Selected microorganisms to evaluate antimicrobial activity were yeast Candida albicans , Gram-positive bacteria S.aureus , and Gram-negative bacteria E.大肠杆菌 . Growth kinetics curves in a time-lapse of 24 h are shown in Fig. 9.
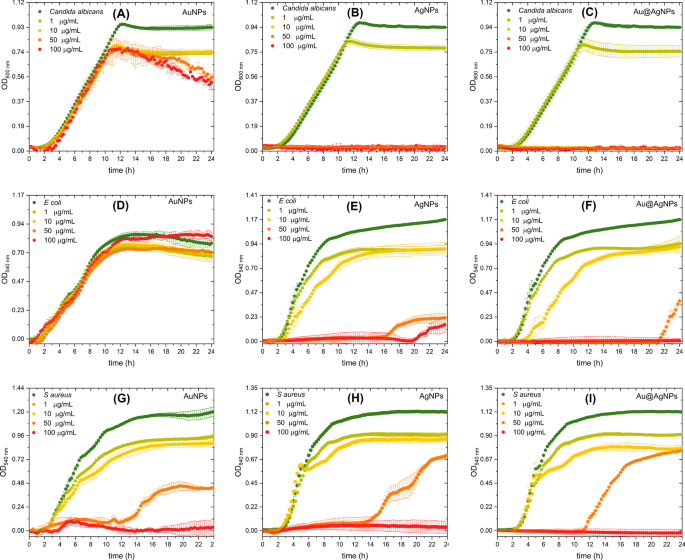
The growth curves using AuNPs, AgNPs, and Au@AgNPs like inhibitory treatments on Candida albicans (a –c ), Escherichia coli (d –f ), and Staphylococcus aureus (g –我 )
图>Candida albicans
AuNPs show no effect on growth kinetics until 10 h (Fig. 9a), varying in a dose-dependent manner the absorbance reached at 24 h. Interestingly, with 50 µg/mL or more, the growth kinetic shows a steep negative slope from 10 h until reaching a 45% reduction at 24 h, which suggests an antifungal effect of these materials. This can be attributed to the ability of gold nanoparticles to interact with relevant proteins present in fungus such as H + -ATPase, affecting proton pump activity. This atrophying the ability of yeast to incorporate nutrients causing its death [61]. In Fig. 9b and c was observed that AgNPs and Au@AgNPs inhibit the growth of the yeast Candida albicans from 10 µg/mL. The determination of the MIC50 concentration for both materials was estimated from the dose–response curve shown in Additional file 1:Figure S8. MIC50 is defined as the concentration of nanoparticles that produces a 50% decrease in absorbance concerning the control (yeast without treatment). For AgNPs and Au@AgNPs, MIC50 were 2.21 µg/mL and 2.37 µg/mL, respectively.
However, according to the EDS results (Fig. 9b), the silver content in Au@AgNPs is 64.85% mass. Thus, the concentration of silver in Au@AgNPs for MIC50 is 1.53 µg/mL, 30% lower than in the case of AgNPs. Padmos et.阿尔。 have demonstrated that silver nanoparticles possess important antibacterial features, but are cytotoxic by mammalian cells this effect has reduced using bimetallic nanoparticles especially using gold in the core of bimetallic nanoparticles [35].
Escherichia coli
In Fig. 9d, AuNPs do not show significant inhibition (< 15%) or affect the growth kinetics of E.大肠杆菌 . For AgNPs (Fig. 9e) at low concentrations, the Lag phase remains unchanged, but there is a marked decrease in growth ratio indicated by the slope decrement. At 50 µg/mL Lag phase lasts up to 16 h and viability reaches a maximum of 20% at 24 h. For 100 µg/mL, an apparent detachment of the growth phase of the microorganism is not observed. In Au@AgNPs (Fig. 9f), the first two concentrations do not show changes in their growth phase, but a phase delay of up to 2 h is observed compared to the control. It is interesting to note that the lag phase lasts up to 21 h for the 50 µg/mL concentration, finally there is no explicit growth behavior for the 100 µg/mL concentration.
Staphylococcus aureus
A comparative analysis of lag phase regrowth occurred after 12 h for Au (Fig. 9g), Ag (Fig. 9h), and Au@AgNPs (Fig. 9i) in the case of S.金黄色葡萄球菌 at 50 µg/mL. For the highest concentration at 100 µg/mL, there is no growth of the bacteria. Additionally, we observe changes in the slope of respect control for Au, Ag, and Au@AgNPs at 1 and 10 µg/mL.
AuNPs interaction with these Gram-positive bacteria could be due to the charged surface that causes an electrostatic interaction, destabilizing membrane structure. Similar results, but with higher NPs concentrations, are reported for AuNPs synthesized using Ananas comosus fruit extract as reducing agent [98] and blue-green alga Spirulina platensis protein [99].杨等人。 show MIC > 500 µg/mL for S.金黄色葡萄球菌 (CMCC(B)26003), our AuNPs has shown inhibition with 10 times less concentration; in this case, a critical synergy exists with polyphenols molecules on coating and stabilizing the surface of nanoparticle [100]. ROS is generated of less to higher intensity [101] by AuNPs, polyphenols (plant extracts), and AgNPs, so AuNPs in synergy with resveratrol and epigallocatechin gallate (EGCG) promote antibacterial response over S.金黄色葡萄球菌 [102] had the most feasible mechanism in this case. Penders et al. reported 250 and 500 µg/mL of AuNPs-like antibacterial agents over S.金黄色葡萄球菌 increases in bacterial growth lag time and antibacterial effect [61, 98, 99].
We believe that inhibition is caused by AgNPs [103] accumulation and diffusion on bacteria related to NPs surface charges that promote electrostatic interactions [104] with the bacteria's membrane leading to higher penetration and damage. We think this is a similar mechanism described for interactions between E.大肠杆菌 biofilms and AgNps [105].
For Au@AgNPs, the obtained results are comparable to those reported by other workgroups [100, 101]; however, different authors suggest that the inhibition of the growth of the microorganisms is directly related to the thickness of the shell [100, 101]. Core–shell NPs showed low cytotoxicity when tested in NIH-3T3 fibroblasts cells (normal mammalian cells) [35]. A lower proportion of silver in the shell of the Au@AgNPs shows similar results to AgNPs [100], and Au core potentializes antibacterial effect, and minimizes the cytotoxicity.
Curves Growth Analyzed by the Modified Gompertz Model
To know how the growth ratios (µ ) and Lag phase (λ ) are quantitatively modified, the growth curves of microorganisms exposed to different concentrations of nanomaterials (Fig. 10) were adjusted by the Gompertz model (Eq. 2).
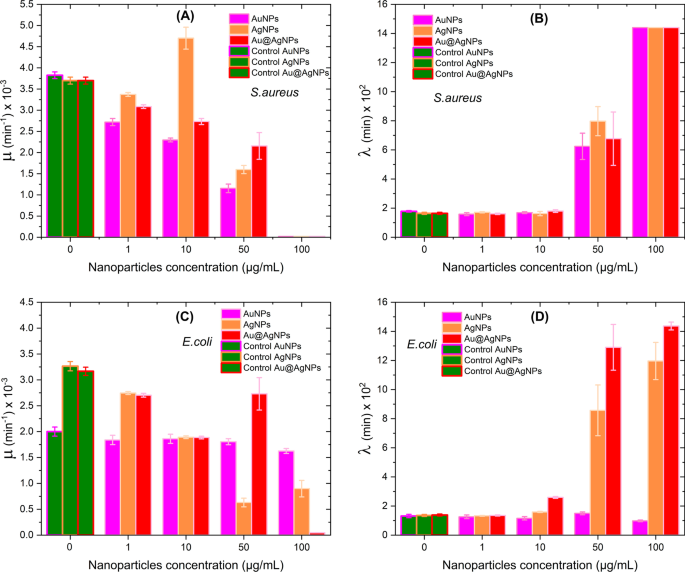
Kinetic parameters were obtained by the Gompertz model. Growth Rate µ and Time Lag Phase \(\lambda\) for S.金黄色葡萄球菌 (a , b ) and E.大肠杆菌 (c , d )
图>In Fig. 10a, it can be seen that all nanomaterials produce a decrease in the replication rate of S.金黄色葡萄球菌 populations when the concentration of nanoparticles increases. This effect results in slightly higher sensitivity for AuNPs. At a concentration of 50 µg/mL, the growth ratio is only 30% concerning control (Additional file 1:Tables S1-S24); at a concentration of 100 µg/mL, all materials inhibit the growth of the S.金黄色葡萄球菌 人口。 The behavior of the adaptive phase for S.金黄色葡萄球菌 with the different treatments is shown in Fig. 10b. It is observed that there are no significant differences in the material used, and at 50 µg/mL, the Lag phase has increased by almost 5 times compared to the adaptive phase of S.金黄色葡萄球菌 (Additional file 1:Tables S1-S24). In general, we can establish that the different nanomaterials evaluated in S.金黄色葡萄球菌 reduce the replication rate and postpone the adaptive phase in a dose-dependent manner until its inhibition at 100 µg/mL.
Figure 10c clearly shows that AuNPs do not affect the growth ratio µ E。大肠杆菌 细菌。 Meanwhile, AgNPs produce a decrease over µ , reaching a minimum value corresponding to 19% to the control (µ for E.coli without treatment) for 50 µg/mL (see Additional file 1:Table S52). In contrast, Au@AgNPs completely inhibit the E.大肠杆菌 growth at 100 µg/mL. Analysis of the behavior of E.大肠杆菌 Lag phase exposed to different materials is shown in Fig. 10d. In this case, unlike Fig. 10b, each material has a characteristic response. Thus, AuNPs do not generate any modification in the adaptive phase of E.大肠杆菌 , while AgNPs and Au@AgNPs have a dose-dependent effect on the Lag phase, the latter material standing out. Thus, we can establish that AuNPs have no appreciable effect on E.大肠杆菌 bacteria, and Au@AgNPs can inhibit replication and, therefore, indefinitely postpone the Lag phase of E.大肠杆菌 . Interestingly, this effect is not achieved for AgNPs even though the net silver content is higher than in Au@AgNPs. This suggests that the core@shell presentation of both metals produces a synergy that favors antimicrobial activity. Feng et.阿尔。 have reported an electron compensation phenomena from Au to Ag in core–shell and alloy structures, which derive in enhance the cytotoxicity of nanoparticles but kept it the antibacterial properties, that means, a synergy between Au and Ag are assumed, due to observed differences between the monometallic and bimetallic materials [106], but more research is necessary.
Figure 11 shows the results of the direct count study of colonies of microorganisms exposed to nanomaterials. In general, the behavior of the microorganism populations reproduces the results obtained from the growth kinetics study (Fig. 7a, e, i). For example, in Fig. 11a, the population of microorganisms (represented logarithmically) decreases significantly only at 19 h where AuNPs have killed 92% of C. albicans . Interestingly, for nanoparticles containing silver (Fig. 11b, c) a more pronounced population decline is observed. Au@AgNPs system at 50 µg/mL, almost entirely inhibits S.金黄色葡萄球菌 at 7 h (99.5% of bacteria killed) although microorganism reactivates its growth for later times. MBC determination was not possible to obtain for tested concentrations (Additional file 1:Figure S9-11), according to experimental observation higher concentrations are required to show this effect. However, for C. albicans , AgNPs showed an MBC value of 50 µg/mL (Additional file 1:Table S55).
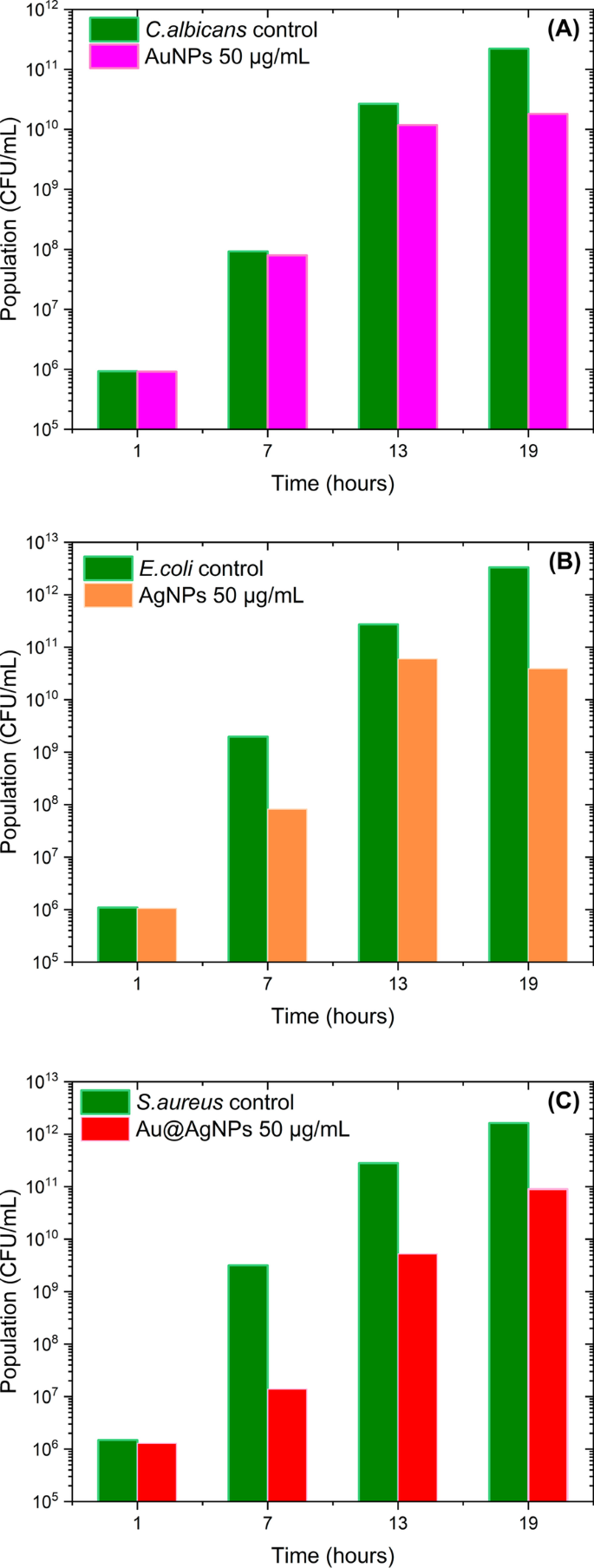
Effect of nanoparticles over microorganisms growth C. albicans exposed to AuNPs (a ), E.大肠杆菌 exposed to AgNPs (b ), and S.金黄色葡萄球菌 exposed to Au@AgNPs (c )。 All microorganisms were exposed at 50 µg/mL nanoparticles concentration
图>Conclusions
For the first time, the production of gold nanoparticles and core@shell (Au@Ag) is reported using a Rumex hymenosepalus root extract as a reducing agent. To obtain Au@AgNPs is proposed a two-step sequential method that produces particles with moderate polydispersity and homogeneous silver shell. Determination of the growth curves and their parameters obtained through the Gompertz model indicate different effects of the nanomaterials on evaluated microorganisms. Inhibitory effects of AuNPs over S.金黄色葡萄球菌 are reached at a concentration of 5 times less to report for other AuNPs synthesized by different processes. This reveals the importance of the synthesis process followed and the environment on the surface of the nanoparticles. On the other hand, AgNPs and Au@AgNPs produce a great growth of the lag phase (> 12 h). However, bacteria can adapt and initiate their growth at these sub-inhibitory concentrations with the consequent risk of generating resistance to these nanomaterials. This highlights the importance of conducting growth kinetic studies that cover an appropriate period to discard a delayed growth. Interestingly, Gompertz's analysis indicates that Au@AgNPs present a higher effect on the growth kinetic of microorganisms than shown by monometallic nanoparticles, which can be attributed to a synergistic effect of both metals on the core@shell structure. Bactericidal effects are only achieved in C. albicans exposed to AgNPs. More experiments must be carried out on higher concentration ranges of these nanomaterials (> 200 µg/mL) to determine their MBC on the studied microorganisms.
数据和材料的可用性
All data generated or analyzed during this study are included in this article and its supplementary information file.
缩写
- AuNPs:
-
金纳米粒子
- AgNPs:
-
银纳米粒子
- Au@AgNPs:
-
Gold–silver bimetallic nanoparticles with core@shell structure
- DLS:
-
动态光散射
- EDS:
-
能量色散X射线光谱
- EGCG:
-
Epigallocatechin gallate
- FTIR:
-
傅里叶变换红外光谱仪
- HAADF:
-
High angle annular dark field images
- MBC:
-
Minimal bactericidal concentration
- MIC:
-
Minimum inhibitory concentration
- Rh:
-
Rumex hymenosepalus Root extract
- TEM:
-
透射电子显微镜
- STEM:
-
Scanning transmission electron microscope
- 紫外可见光:
-
Spectroscopy ultraviolet–visible spectroscopy
- XPS:
-
X射线光电子能谱
- XRD:
-
X射线衍射
纳米材料
- 氧化铜纳米颗粒对大肠杆菌的生物合成、表征和抗菌潜力评估
- 小型硒纳米晶体和纳米棒的简便合成和光学特性
- 用贵金属纳米粒子装饰的电纺聚合物纳米纤维用于化学传感
- 涂有 CuS 纳米粒子的有色导电 CuSCN 复合材料的简便合成
- 使用多功能 GaN/Fe 纳米颗粒靶向内皮细胞
- 合成单分散二元 FePt-Fe3O4 纳米粒子的后处理方法
- Ag 纳米颗粒/BiV1-xMoxO4 与增强的光催化活性的协同效应
- La1 − xSr x MnO3 锰酸盐纳米颗粒的合成方法对其性能的影响
- PEG 包覆的 CoFe2O4 纳米颗粒的毒性与姜黄素的处理效果
- 用银纳米粒子作为抗菌剂装饰的基于氧化石墨烯的纳米复合材料
- 配体掺杂的氧代氢氧化铜纳米粒子是有效的抗菌剂
- 评估由食用菌根真菌 Tricholoma crassum 合成的蛋白质包覆金纳米粒子的抗菌、凋亡和癌细胞基因传递特性