评估由食用菌根真菌 Tricholoma crassum 合成的蛋白质包覆金纳米粒子的抗菌、凋亡和癌细胞基因传递特性
摘要
很少报道具有不同几何形状的具有高功能蛋白质涂层的金纳米粒子的生物合成,而无需额外的加帽步骤。本研究首次描述了从食用菌根真菌Tricholoma crassum中绿色合成蛋白质包覆的金纳米粒子 (伯克。)Sacc. 纳米颗粒的尺寸范围为 5-25 nm,并且具有不同的形状。光谱分析表明,在生产过程中,随着反应时间的延长,吸收最大值发生红移,随着pH值的增加,吸收最大值发生蓝移。这些通过光谱学、SEM、TEM、AFM、XRD 和 DLS 进行表征。可以通过改变合成参数来改变粒径。它们对细菌、真菌和多重耐药病原菌具有强效抗菌活性。这些也对细菌的生长动力学和真菌孢子的萌发具有抑制作用。当用彗星试验进行测试时,这些在真核细胞上显示出凋亡特性。此外,这些颗粒被天然的 40 kDa 蛋白质覆盖,该蛋白质被用作基因的附着位点,以便将其传递到肉瘤癌细胞中。目前的工作还尝试使用溶血试验优化这些纳米颗粒的安全剂量,以用于治疗。讨论了纳米颗粒在发酵罐中的大规模生产以及该颗粒的其他可能应用。
背景
随着纳米粒子在农业、医药和家用产品中不可避免的广泛应用,了解其有害影响和减少有害影响的方法的压力越来越大[1]。使用活生物体或其酶进行纳米材料的绿色合成具有生态友好、成本效益高且可安全用于治疗的优点 [2]。在微生物中,丝状真菌具有更大的合成纳米颗粒的能力,因为它们能够分泌更多的酶 [3, 4]。细胞外金纳米粒子 (AuNPs) 已使用多种真菌制备 [5,6,7],但在这些报告中仅提及这些粒子上的天然蛋白质外壳 [8, 9]。
在医学中,随着多重耐药菌的出现,纳米粒子是替代选择,因为它们不会产生耐药性 [10]。 AuNPs 在肿瘤细胞的治疗和诊断以及基于纳米载体的基因传递方面特别有希望 [11,12,13]。在生理条件下,AuNPs 通过细胞膜显示出低渗透性,但在肿瘤细胞中,由于增强的渗透和保留 (EPR) 效应,吸收增强 [14]。当 AuNPs 被蛋白质覆盖时,这种吸收会进一步增强。蛋白质帽有助于将纳米颗粒稳定在胶体状态,并为药物或基因提供对接位点以进行递送 [14]。然而,加盖过程涉及额外的步骤。天然蛋白质帽的提供消除了化学途径的应用,这些途径大多是危险的 [5]。尽管它很重要,但关于具有天然蛋白质涂层的贵金属纳米颗粒的绿色合成的报道有限[15, 16]。
在将 AuNP 应用于治疗之前,需要检测的一个重要方面是纳米材料与细胞膜的生物相容性 [6]。另一方面是细胞毒性,AuNPs 可以聚集红细胞 [13]。因此,需要重点关注危险方面并在安全范围内使用[7, 17]。
我们实验室目前报道了唯一一种可产生胞外银纳米粒子的食用菌根真菌,该真菌为Tricholoma crassum (伯克。)Sacc [2]。在这里,我们描述了从 T 绿色合成 AuNP。克拉苏姆 大小范围为 5-25 nm 且具有不同形状。对它们进行了表征和分析,以确定其对细菌、真菌以及多重耐药 (MDR) 病原菌的抗菌活性。这些对细菌的生长动力学和真菌孢子的效力具有抑制作用。最重要的是,这些颗粒是天然的蛋白质涂层。我们已经测试了这些颗粒作为将基因传递到癌细胞中的载体的功效。进行溶血试验以检查这些颗粒的生物相容性和毒性。 AuNPs 的凋亡特性在真核细胞上用彗星试验进行了测试,以估计具有最小副作用的治疗用途的可行浓度。因此,目前的工作试图在安全范围内优化 AuNPs 在医学和纳米技术界面上的合成和应用,以尽量减少对环境和生物的损害。
方法
真菌、细菌和植物生长条件
鹅口疮 (伯克。)萨克。用于纳米颗粒的生产。对于抗菌测定,E.大肠杆菌 (DH5α), 根癌农杆菌 (LBA4404)、大肠杆菌的多重耐药 (MDR) 菌株。大肠杆菌 (DH5α)和A。根瘤菌 (LBA4404) 被使用。植物病原真菌Magnaporthe oryzae 和黑链格孢 被使用。番茄(品种 Pusa Ruby)和烟草(品种 SR1)幼苗在生长室中的土壤上生长,光照/黑暗光周期为 16:8 h,28 ± 1°C,光强度为 50 μmol m − 2 s − 1 .
AuNPs 的合成
T。克拉苏姆 菌丝体在马铃薯葡萄糖肉汤 (PDB) 中于 28°C 培养 7 天。 1 g 菌丝垫与 10 ml 去离子水在振荡器上以 50 RPM 在 28 °C 下搅拌 24、48 和 72 小时。上清液通过 Whatman 滤纸过滤。 1. 根据我们的报告 [2] 将细胞滤液 (pH 5.2) 与 1 mM 氯金酸盐 (HAuCl4) 水溶液一起孵育,并在 28°C 下在黑暗中搅拌 1 小时,用于 24、48 制备的每种类型的细胞滤液, 和菌丝体孵育 72 小时。
为了在不同 pH 值下生物合成 AuNP,在孵育前使用 1 M HCl 或 1 M NaOH 将无细胞滤液的 pH 值调节到酸性范围 (3.5) 和碱性范围 (7、8 和 9)。还使用不同的反应温度(0、15、28、75 和 100°C)、不同浓度的无细胞滤液(× 0.5、× 1、× 2)和氯金酸盐离子(0.5、1、2 mM ).
紫外可见光谱
使用紫外-可见分光光度计分析与氯金酸盐溶液孵育 1 小时的每个细胞滤液的上清液的吸光度,并在 450 至 750 nm 之间绘制吸光度光谱图。
扫描电子显微镜 (SEM)、透射电子显微镜 (TEM)、原子力显微镜 (AFM) 和 X 射线衍射 (XRD)
这些分析是根据 Chowdhury 等人进行的。 [15] 稍加修改。使用扫描电子显微镜 (SEM)、透射电子显微镜 (TEM)、原子力显微镜 (AFM) 表征使用 24 小时细胞滤液,然后在 28°C (pH 5.5) 下与 1 mM HAuCl4 溶液孵育 1 小时的金纳米粒子, 和 X 射线衍射 (XRD)。将玻璃棒上的 AuNPs 薄膜真空干燥,并使用 FEI Quanta 200(FEI,美国)进行扫描电镜分析。
AuNP 的形状和尺寸由 TEM 确定。将一滴 (10 μl) AuNP 悬浮液置于涂有碳的铜网上,并在装载到样品架之前进行真空干燥。这些纳米颗粒的 TEM 显微照片是使用具有低电压 (100 kV) 结构的 TECNAI G TEM 获得的。
对于 AFM 成像,AuNP 被沉积在新鲜切割的白云母红宝石云母片(红宝石云母有限公司,印度)上,并使用真空干燥器进行干燥。使用 Pico plus 5500 ILM AFM (Agilent Technologies, USA) 进行声交流电 (AAC) 模式 AFM。
对于 XRD 研究,将 AuNP 悬浮液薄膜均匀地铺在载玻片上,并使用真空干燥器进行干燥。在 D8 Advance DAVINCI XRD 系统(Bruker AXS Pvt. Ltd.)中记录 XRD 图案,在 40 kV 电压和 40 mA 电流下使用 CuKα 辐射(λ =1.54060/1.54443 Å),并记录衍射强度35°到80°2θ角。
计算机软件分析
AuNP 的测量和直方图的构建是使用 OLYMPUS 软件 MEASURE IT 工具完成的。根据 Sriram 等人的方法计算纳米颗粒的浓度。 [18] 和我们之前的出版物,Chowdhury 等人。 [15].
细菌转化以产生多重耐药性
A.根瘤菌 菌株 LBA4404 和 E。大肠杆菌 DH5α菌株通过使用我们公开的方案[2, 15]分别使用质粒pCAMBIA2301和pUC19和pZPY112进行转化,使其具有多重耐药性。
抗菌试验和细菌生长试验
这些测定是根据我们公布的方案 [15] 进行的。使用 24 小时细胞滤液并在 28°C (pH 5.5) 下与 1 mM HAuCl4 溶液孵育 1 小时合成的金纳米颗粒用于所有生物测定。对于纸盘检测,使用越来越多的多分散 AuNP(0.249、0.498、0.747、0.996、1.245 μg)。从每个细菌菌株的新鲜过夜培养物中,将 25 μL 等分试样涂在 LB 琼脂平板上。使用浓度为 31.121 mg/L 的 AuNP 溶液和无菌去离子水配制纳米颗粒溶液的稀释系列。将直径为 5 毫米的无菌纸盘放置在细菌平板上并孵育,每个盘中金纳米粒子的量逐渐增加,例如 0.249、0.498、0.747、0.996 和 1.245 μg(总体积为 40 μl)。 A.根瘤菌 板在 28°C 下孵育 48 小时,然后在大肠杆菌中孵育。大肠杆菌 在 37°C 下保持 12 小时。对于 DH5α 和 LBA4404 的生长测定,7.5 ml 细菌培养物补充了 2.5 ml AuNPs。
抗真菌检测
M的水悬浮液。稻草 孢子在 6.1 × 10 5 孢子/毫升与血细胞计数器。将 150 μl 这种悬浮液涂在 MEA 板上。将直径为 5 毫米、多分散 AuNP(0.249、0.498、0.747、0.996 和 1.245 μg)量增加的无菌纸盘放在板上并在 28°C 下孵育。 2 天后测量抑制区。
用 AuNPs 处理的细菌细胞的抗菌试验
在 28°C 下用等体积的 AuNP 悬浮液 (15.56 mg/L) 处理过夜的液体 LBA4404 培养物 12 小时。将 50 μl 悬浮液与等体积的 0.4% 台盼蓝溶液(0.5 g 台盼蓝、500 ml 甘油、450 ml 蒸馏水、50 ml HCL)混合,在复合显微镜(Leica DMLS,德国)下观察。
存在 AuNP 的真菌孢子萌发试验
用 20、40、60、80 和 100% v 制备一系列稀释的纳米颗粒 /v 使用浓度为 15.56 毫克/升的纳米颗粒的原液,用水将最终体积定为 100 微升。将等体积的这些悬浮液加入 50 μl Alternaria solani 孢子悬浮液(4.2 × 10 5 孢子/毫升)并在 28°C 下孵育。在复式显微镜(Leica DMLS,德国)下观察 0、2、4、6 h 的孢子。
彗星分析
AuNPs 的凋亡特性是通过标准彗星测定法 [19] 测量的,几乎没有修改。烟草或番茄叶暴露在浓度不断增加的环境中(0、15、20 和 30% v /v 烟草; 5、10、15 和 20% v/v 番茄)AuNP(15.56 毫克/升储备)24 小时。在 4°C 下以 0.74 V/cm(25 V,300 mA)进行分离核的电泳 30 分钟。用 0.4 M Tris 缓冲液中和载玻片,在甲醇中脱水,用溴化乙锭 (20 μg/ml) 染色,并在具有 515-560 nm 激发滤光片和 590 nm 屏障滤光片的荧光显微镜下观察。数据采用Tritek CometScore软件进行分析。
SDS-PAGE
根据我们的出版物[15]分离蛋白质。为了分析与纳米颗粒结合的蛋白质,将 AuNP 用无菌水洗涤并在 Laemmli 缓冲液中煮沸 10 分钟,然后以 8000 rpm 的速度离心 10 分钟。 SDS 处理和未处理的样品在 12% SDS-PAGE 上运行。
癌细胞系培养
肉瘤 180 细胞系在含有 10% 胎牛血清、200 U/ml 青霉素和 200 μg/ml 链霉素的 RPMI 1640 培养基 [20] 中在 37°C、5% CO2 加湿培养箱中培养。
将质粒 DNA-AuNP 复合物递送到癌细胞中
使用公开的方案 [15] 从含有 pCAMBIA1302 的 DH5α 中分离质粒。含有gfp结构的质粒 使用标准方案将克隆在 pCaMV35s 启动子下的序列递送到肉瘤 180 细胞中 [21]。在荧光显微镜下使用对 GFP 具有特异性的过滤器(激发最大值 =395 nm)(Axioskop-40,Carl Zeiss)观察细胞。保留用裸质粒DNA处理的细胞作为对照。
溶血测定
按照标准方案进行溶血测定[22]。等体积的红细胞(1.6 × 10 9 红细胞/毫升)用不同浓度的金纳米粒子(0.1、0.5、1、5、10 和 20 微升/毫升,来自 15.56 毫克/升的储备)处理 1 小时,并计算不同浓度纳米粒子的溶血活性。
结果与讨论
pH 5.5 条件下 AuNP 的生物合成
在合成过程中,由于表面等离子体共振 (SPR) 的变化,细胞滤液的颜色从浅黄色变为紫色 [23],表明 AuNP 的形成。不含真菌滤液的 1 mM HAuCl4 溶液呈黄绿色(图 1a,“A”)和 T 的细胞滤液。克拉苏姆 呈淡黄色(图 1a,“B”)。孵育后,混合物的颜色在 1 小时内变为紫色(图 1a,“C”),表明形成了 AuNP。
<图片>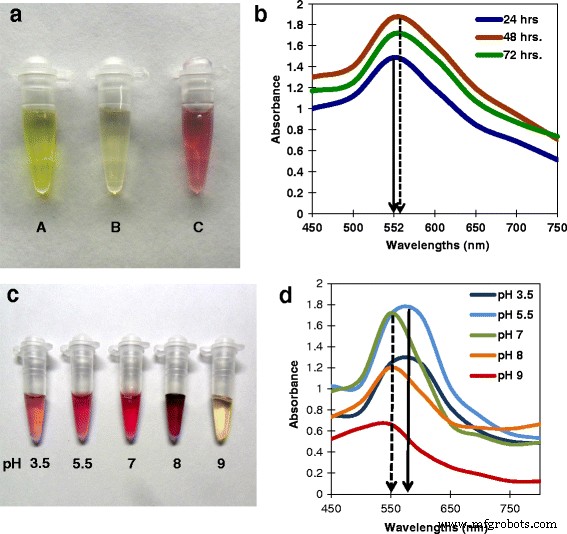
使用苔藓生物合成AuNPs 和光谱分析。 一 反应过程中颜色发生变化。 A 20 mM HAuCl4 溶液。 B 鹅口疮的无菌丝体细胞滤液 . C 1 mM HAuCl4 和 24 小时细胞滤液,1 小时显示紫色,表明 AuNP 的合成。 b 不同孵育时间(24、48、72 小时)合成的 AuNP 的紫外-可见光谱。实线箭头显示 24 小时孵育期在 552 nm 处的吸收峰。虚线箭头表示随着潜伏期的增加吸收最大值的轻微红移。 c 在不同 pH 值下合成的 AuNPs 显示出不同的颜色。 d 紫外可见光谱相同。实线箭头表示 pH 5.5 在 552 nm 处的吸收峰,虚线箭头表示吸收最大值随 pH 增加而发生蓝移
图>从微生物生产纳米粒子的其他方法的一个缺点是这些方法很耗时,并且生物体会产生毒素。以前,Phanerochate chrysosporium 利用无细胞提取物在 90 分钟内生产 AuNP [3]。最近,利用Sporosarcina koreensis生物合成了银和金纳米颗粒 使用延长的 1-2 天反应时间 [24]。在我们的报告中,AuNP 的生产时间已缩短至仅 1 小时。
紫外到可见光谱
金属纳米粒子的光吸收光谱受形状、聚集和 SPR 控制,它们根据粒子大小和形状而变化 [25, 26]。图 1b 显示了使用 24、48 和 72 小时后产生的细胞滤液合成的 AuNP 的紫外可见光谱,然后与 1 mM HAuCl 4 溶液(pH 5.5)孵育 1 小时。此处,24 小时细胞滤液的吸收峰位于 552 nm。首先出现在 552 nm 处的横向等离子体共振带从 24 小时到 48 小时和 72 小时略微向红色移动(如图 1b 中实线与虚线所示),证实了吸光度强度逐渐放大的红移。峰的加宽表明颗粒是多分散的。强 SPR 以大约为中心。 550-560 nm 是典型的胶体金(图 1b)。随着细胞滤液的浓度随着孵育时间(即 24、48、72 小时)的增加而增加,吸光度也成比例地增加。当反应在 28°C 下进行时,它在 1 小时后达到平衡并稳定超过 30 天,没有聚集的迹象,这可能是由于稳定的蛋白质外壳。在之前的研究中,BSA 涂覆的 AuNP 没有显示出聚集 [27]。使用用 24 小时细胞滤液制备的 AuNP 进行了进一步研究。
AuNPs 在不同 pH 和紫外可见光谱下的生物合成
AuNPs 在 3.5、5.5、7、8 和 9 的不同 pH 值下合成,导致粉红色至深紫色(图 1c)。 UV-vis 光谱显示吸光度最大值,SPR 波长从 pH 3.5 增加到 pH 5.5,导致红移。然而,随着 pH 从 7 进一步增加到 pH 9,吸光度最大值和 SPR 波长降低,显示出蓝移(图 1d)。 pH 9 处的峰幅度较小且颜色不变,表明仅形成了少量 AuNP。这是一种酶促生物合成,pH 9 可能会抑制形成 AuNP 所需的酶反应(图 1d)。不同pH下产生的纳米颗粒在室温下1个月后没有聚集。
使用不同温度、底物浓度和前体浓度生产 AuNPs
生物合成过程中物理化学条件的优化对于功能高效的纳米颗粒的产生至关重要 [28]。使用更高浓度滤液的合成表明 AuNP 的产量增加,具有更高的颜色强度和吸光度最大值(图 2a、b)。 × 2 滤液合成时观察到最大局部表面等离子体共振 (LSPR) 的轻微蓝移,表明颗粒间距离增加,簇大小减小 [29, 30]。
<图片>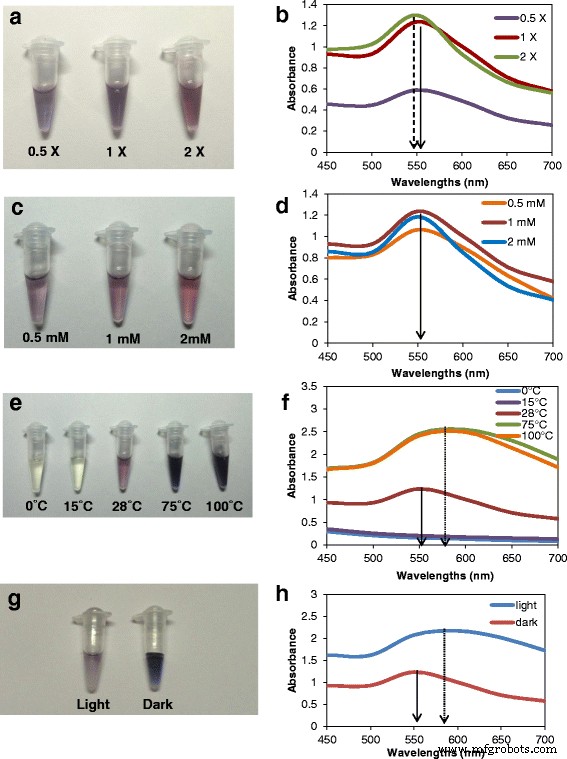
苔藓 AuNPs的生物合成及紫外-可见光谱 使用不同的合成参数。 一 , b 不同浓度的细胞滤液。 c , d 不同浓度的HAuCl4。 e , f 反应温度不同。 g , h 在黑暗和光线下。实线箭头表示 28 °C pH 5.5 时 × 1 细胞滤液和 1 mM HAuCl4 在 552 nm 处的典型吸收峰,虚线箭头表示吸收最大值蓝移,虚线箭头表示 SPR 带红移
图>在测试的三种氯化金浓度(0.5、1 和 2 mM)中,1 mM 的合成最大,吸收最大值为 552 nm。 (图 2c、d)。发现 28°C 是合成的最佳温度。较高的温度(75 和 100°C)虽然介导了 AuNP 的更快合成,但深色和吸收最大值的红移(图 2e、f)表明颗粒比 28°C 时更大。在 0 和 15 °C 下,550-600 nm 内没有显色或吸收峰。在光下合成导致深紫色(图 2g)和吸收最大值的红移,具有表示较大颗粒的宽峰(图 2h)。使用 DLS 测定粒度和数量(图 3a-j)。纳米颗粒大小和聚集的这种变化取决于相关有机物的性质和数量[30],而有机物又随合成条件而变化。
<图片>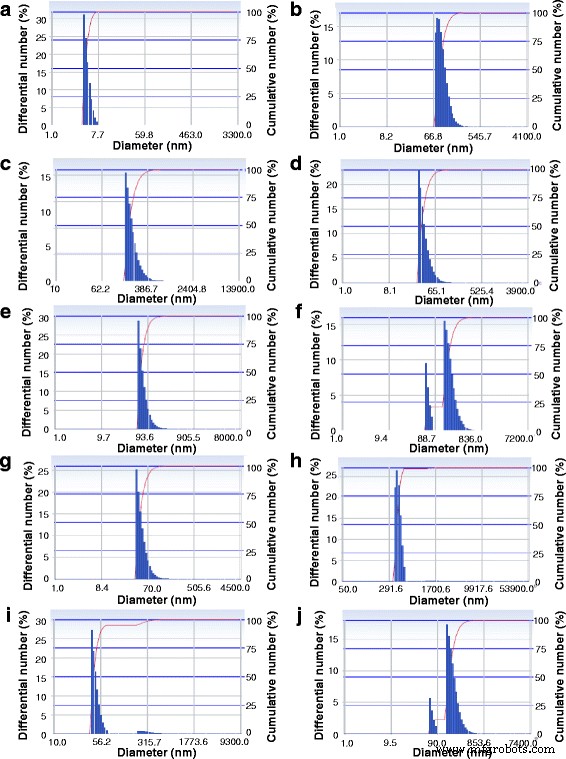
DLS 显示合成的 AuNPs a 的尺寸分布 使用 × 1 无细胞滤液,1 mM HAuCl4,28°C 避光,b 在光线下,c × 0.5 无细胞滤液,d × 2 无细胞滤液,e 在 0 °C,f 在 15°C,g 在 75°C,h 在 100°C,i 含 0.5 mM HAuCl4 和 j 含 2 mM HAuCl4
图>扫描电子显微镜 (SEM) 和透射电子显微镜 (TEM)
SEM 在× 80000 放大倍数下显示出小尺寸和独特几何形状的 AuNP(图 4a)。 TEM 分析清楚地显示了尺寸范围为 2-22 nm 直径的多分散 AuNP(图 4b)。尺寸分布图显示尺寸范围为 5-10 nm 的 AuNP 频率最高,其次是 2-5 nm、10-15 nm、15-20 nm 和 20-22 nm(图 4c)。它们具有不同的几何形状,如直径小于等于 5 纳米的小圆形或菱形、六边形、长方体、等腰三角形和边长从 4.36 到 22.94 纳米不等的近等边三角形(图 4d、e)。
<图片>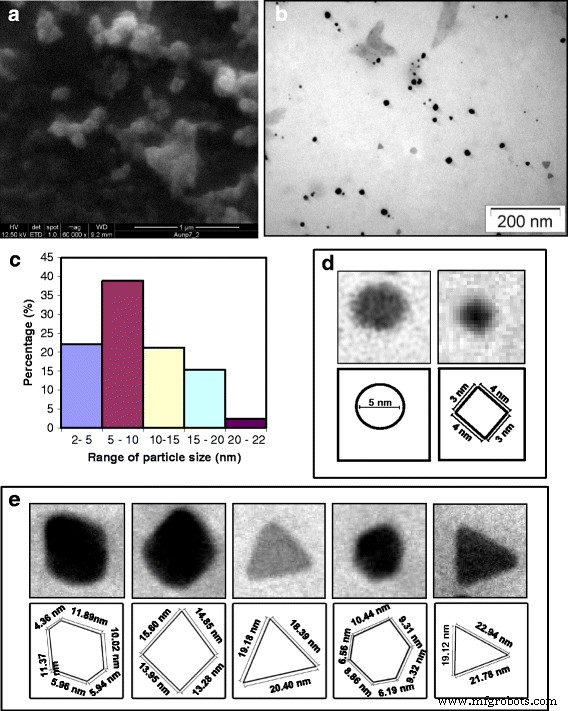
AuNPs的电子显微镜。 一 SEM放大倍数× 80,000。 b TEM 显示在微观领域中不同尺寸的分散颗粒。 c 显示 AuNP 粒径分布的直方图。单个 AuNP 的放大视图,显示不同的几何形状及其尺寸图解。 d 小尺寸范围的球形(左)和菱形(右)。 e 左起:六边形、等边三角形、菱形、多面体、等腰三角形
图>原子力显微镜 (AFM)
图 5a 显示了分散的 AuNP 的 AFM 图像。二维视图(图 5a)显示颗粒具有几乎相似的表面厚度。这些粒子的高度用单表面 3D AFM 可视化(图 5b)。位于随机线性区域(用虚线标记,图 5c)上的 AuNP 的 AFM 2D 图显示的高度范围为 1 到 4 nm。平面表面等离子体带表明颗粒的厚度小于边长。
<图片>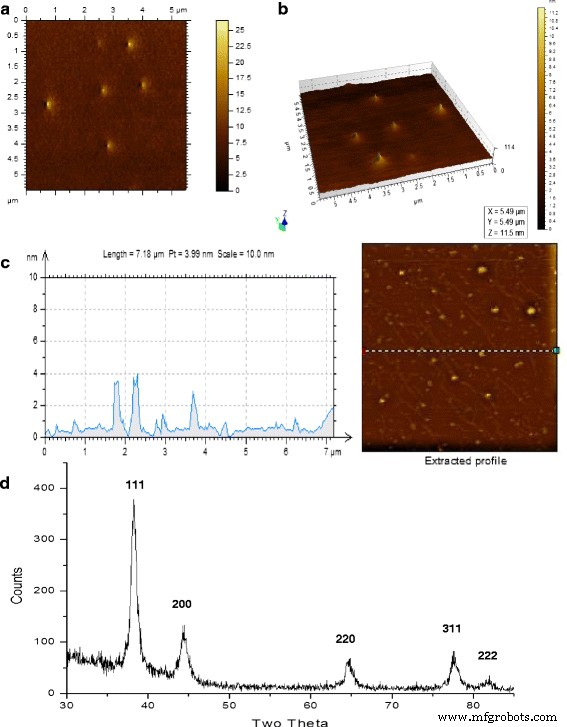
AuNPs的AFM和X射线衍射。 一 AFM 图像:顶视图。 b AFM 图像:三维视图。 c AuNP 的 AFM 图像和位于场中虚线上的纳米粒子高度的图形轮廓。 d 纳米粒子的 X 射线衍射图显示金的典型峰
图>X 射线衍射研究 (XRD)
颗粒薄膜的 XRD 图案显示仅存在 AuNP。在 38° 附近的强衍射峰通常归因于面心立方 (FCC) 结构的 {111} 面 [3]。此处的 XRD 图案显示出 38.23 处的主要衍射峰,这归因于 FCC 结构。其他四个面的衍射峰较弱。在 38.23、44.31、64.60、77.58 和 81.63 处的四个不同的布拉格衍射峰与 AuNPs 的非常匹配(图 5d,附加文件 1:表 S1)。
金纳米粒子浓度计算
发现纳米颗粒 [15] 的浓度为 15.56 毫克/升,用于用 1 mM HAuCl4 孵育 24 小时的细胞滤液产生的颗粒。
使用致病细菌和真菌对 AuNP 进行抗菌分析
AuNPs对人类细菌以及植物病原菌和真菌表现出很强的抗菌活性。使用纸盘测定纳米颗粒的抗微生物活性,其中 AuNP 的量增加,即 0.249、0.498、0.747、0.996 和 1.245 μg。人类细菌E。大肠杆菌 (DH5α),植物病原菌A。根瘤菌 (LBA4404) 和植物病原真菌 M.稻草 被使用。即使在最低浓度下,AuNP 也能抑制所有这些微生物,并且抑制区与颗粒浓度的增加成比例地增加(图 6)。图 6a-c 显示了 E 的抑制区。大肠杆菌 (DH5α),A。根瘤菌 (LBA4404) 和 M.稻草 , 分别。图 6f-h 显示了这三种微生物的抑菌圈与 AuNP 用量的函数关系图。单独的真菌提取物没有任何抑制作用。三种微生物的抑制比较趋势表明,与 LBA4404 和 M 相比,对 DH5α 的抑制作用更大。稻草 (附加文件 2:图 S1a)。
<图片>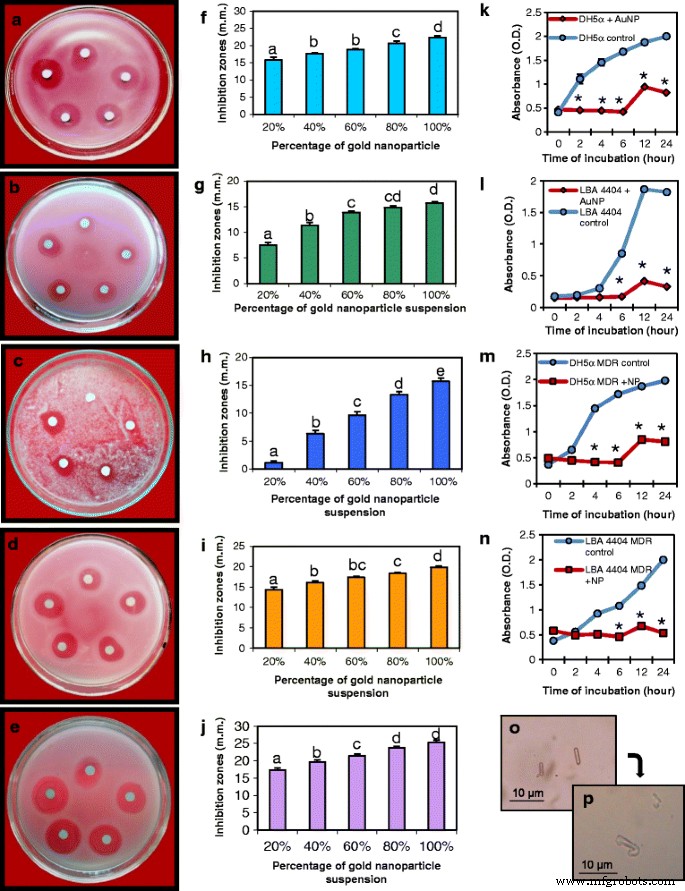
金纳米粒子对病原菌、真菌和多重耐药 (MDR) 细菌的抗菌特性分析。 一 , f 板和相应的图表显示了纳米颗粒的圆盘扩散试验,随着对大肠杆菌的抑制区增加。大肠杆菌 .在与 b 类似的测定中获得的抑制区 , g 根癌农杆菌 . c , h 稻瘟病菌 . d , i 耐多药E。大肠杆菌 . e , j MDR A.根瘤菌 .所有的实验都是在纸盘上增加 AuNPs 的量来完成的。从顶部顺时针方向:0.249 μg (20%)、0.498 μg (40%)、0.747 μg (60%)、0.996 μg (80%) 和 1.245 μg (100%) 的 AuNP。数据是三个重复的平均值 ± SE。不同字母表示样本间差异有统计学意义(P <0.05,Tukey 的 HSD 检验)。 AuNPs对k生长曲线的影响 E。大肠杆菌 , l A.根瘤菌 , 米 耐多药E。大肠杆菌 , 和 n MDR A.根瘤菌 .星号表示控制的显着差异(学生的 t 测试,P <0.05)。 o 对照A的显微镜检查。根瘤菌 细胞。 p A.根瘤菌 AuNPs处理后细胞完整性丧失
图>使用多重耐药的人和植物病原菌测定AuNPs的抗菌活性
制备了多药耐药(MDR)DH5α 和 LBA4404 携带的带有耐药基因的质粒。携带 pUC19 的 MDR DH5α 对 100 μg/ml 氨苄青霉素和 35 μg/ml 氯霉素具有抗性。用 pCAMBIA2301 转化的 LBA4404 对 25 μg/ml 利福平和 50 μg/ml 卡那霉素具有抗性。 AuNP 对 MDR DH5α 和 MDR LBA4404 显示出有效的抑制活性(图 6d、e)。图 6i、j 是显示抑制随着纳米颗粒浓度增加而增加的图。两种 MDR 细菌的抑制比较趋势表明 A 的抑制区域更大。根瘤菌 与大肠杆菌相比 (附加文件 2:图 S1b)。
在 AuNP 存在下一段时间内的细菌生长测定
用 AuNPs 处理的 DH5α 的生长曲线与对照组有显着差异(图 6k,m)。在 DH5α 和 MDRDH5α 的对照组中,生长曲线的对数期在接种后 2 小时内开始,而在 AuNP 处理的 DH5α 和 MDR DH5α 中,在接种后 6 小时内未观察到生长。对照和处理细胞的生长曲线在 12 小时后达到稳定期,但处理细菌的生长显着降低。 LBA4404 生长曲线显示接种后 4 小时在对照组中开始对数期,而在 AuNP 处理组中则为 6 小时。尽管在对照 MDR LBA4404 中,对数期在 2 小时内开始(图 6l,n),但观察到对 MDR LBA4404 生长曲线的类似影响。
AuNPs 对细菌细胞形态和活力的影响
一般来说,大多数纳米粒子可以有效地粘附在细胞膜上,被吸附,然后影响细胞完整性[31]。正常A。根瘤菌 细菌呈杆状,轮廓清晰(图 6o)。与 AuNP 一起孵育的细菌表现出形态扭曲、外膜崩解导致轮廓不规则,以及细胞形状和大小的完整性丧失(图 6p)。这与之前在 E 中观察到的一致。大肠杆菌 用二氧化硅纳米粒子处理的细胞 [32]。我们发现细菌细胞与纳米颗粒的长时间孵育显示细胞完全崩解。
真菌孢子萌发试验
AuNPs是植物病原真菌Alternaria solani毒力的有效抑制剂 .在不同的孵化期用增加剂量的 AuNP 处理真菌分生孢子显示发芽频率和胚管长度逐渐降低(图 7a)。分生孢子的代表性图片(图 7a)和图表显示,从真菌分生孢子中出现的发芽百分比(图 7b)和胚管的平均长度(图 7c)随着颗粒剂量的增加和孵化的增加而降低期间。 Thus, these AuNPs had significant antifungal property which is mediated through the suppression of germination of spores and retardation in the growth of hyphae.
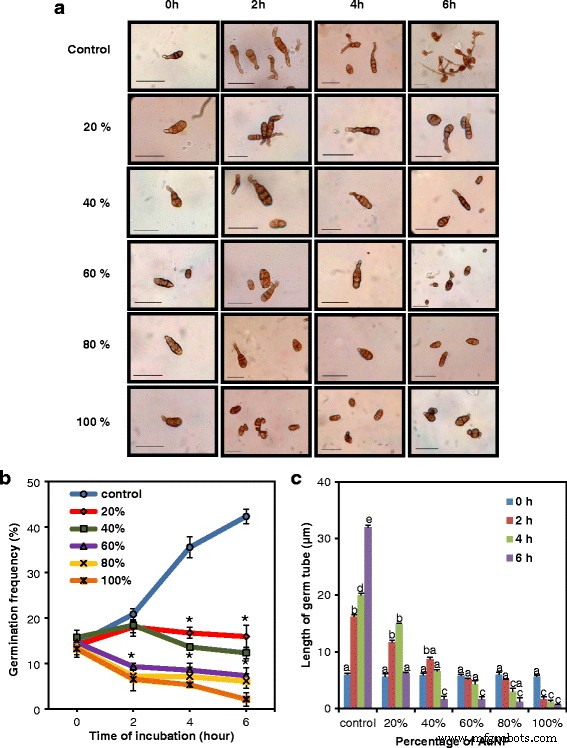
Effect of the AuNPs on the spore germination of plant pathogenic fungus Alternaria solani; 一 Spores after treatment with AuNPs (bar = 30 μm). Rows top to bottom:control spores followed by spores treated with different dilutions of AuNPs (15.56 mg/L stock). Columns left to right:increasing time of incubation with AuNPs showing least germination at 6 h incubation with 100% AuNPs. b Spore germination frequency (%) at different concentrations of AuNPs as a function of time of incubation. Asterisks indicate significant differences to control (Student’s t test, P <0.05)。 c Average germ tube length at different concentrations of AuNPs as a function of time of incubation. Data represents means ± SE of three replicates. Different letters indicate statistically significant differences among the samples (P < 0.05, Duncan’s multiple range test). Spore germination frequency and the average germ tube length decreased with increasing doses of the AuNPs and increasing incubation period
图>Thus, these AuNPs have antimicrobial functions on bacteria as well as fungi, which is rarely reported for AuNPs. These AuNPs being toxic to multi-drug-resistant human bacteria can be utilized in treatment of MDR or extensively drug-resistant (XDR) bacteria-related human diseases which are difficult to treat. Furthermore, the antimicrobial effect against typical phytopathogens like Agrobacterium and fungi, it makes the particles suitable for as bacteriocides and fungicides in the form of nano-agrochemicals. These would enable sustainable management of crop loss through elimination of excessive and indiscriminate use of agrochemicals causing deterioration of soil health, degradation of agro-ecosystem, environmental pollution, and resistance in pathogens [33].
The AuNPs have potent apoptogenic properties
The single cell gel electrophoretic assay or comet assay is a sensitive method to compare apoptoic effects of materials [34, 35]. Treatment of tobacco leaf cells with 7.78 mg/L of AuNPs for a period of 15, 20, and 30 min resulted in gradual increase in apoptosis indicated by increase in percentage of DNA in tail (Fig. 8a, ‘A’, ‘B’, ‘C’, ‘D’). The maximum migration of DNA occurred after 30 min of treatment showing a tail DNA value 28.44 ± 0.74% which was significantly higher than that of untreated control cells (1.7 ± 0.59%). The incubation periods of 15 and 20 min caused less DNA migration with tail DNA values of 7.25 ± 2.56 and 19.19 ± 1.54%, respectively (Fig. 8b). Therefore, these AuNPs have the capacity to induce apoptosis in eukaryotic cells in higher doses. Recently, nanoparticles are being used in novel strategies to target and kill cancer cells. As illustrated by dynamic and quantitative imaging, successful application of nanoparticles as an alternative therapy for cancer depends on the apoptotic properties of the particles [36]. Hence, the present finding shows potent apoptogenic properties of these AuNPs which holds promise in future cancer therapy.
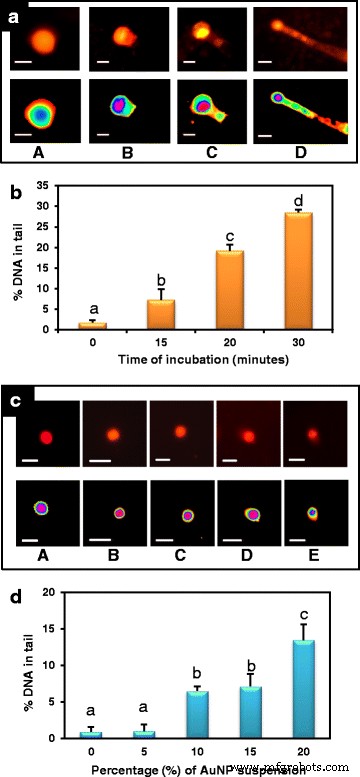
Assay of apoptotic properties of the AuNPs. 一 Comet assay and fluorescent microscopic images of nuclei of tobacco leaves treated with AuNPs for A 0 min. B 15 min, C 20 min, and D 30 min (bar = 5 μm). In the control sets (0 min incubation), most of the DNA is located in the head of the comet while cells subjected to longer treatment show increasing DNA damage and longer comet tails. b Mean of % tail DNA ± SE after different periods of incubation. Data represents means ± SE of three replicates. Different letters indicate statistically significant differences among the samples (P < 0.05, Tukey’s HSD test). c Assay of threshold dosage of AuNPs for apoptosis showing images of nuclei of tomato leaf cells treated with A 0% (control), B 5%, C 10%, D 15%, E 20% of AuNP suspension for 24 h (bar = 10 μm). d Mean % tail DNA ± SE after 24 h treatment with different concentration of AuNPs. Data represents means ± SE of three replicates. Different letters indicate statistically significant differences among the samples (P < 0.05, Tukey’s HSD test). Dosage below 10% show negligible DNA damage while higher than 20% show start of DNA damage
图>These AuNPs are non-apoptogenic at lower doses
Safe application of metal nanoparticles as therapeutic agent needs a pre-determination of biological effect of the particles at the borderline toxicity [37]. A threshold level of nanoparticles required for apoptosis was obtained through treatment of tomato leaf cells with different concentration of AuNP for 24 h (Fig. 8c). Low concentration, e.g., 5% v /v of AuNP stock suspension (15.56 mg/L) did not show significant apoptogenic effect on tomato cells even after 24 h of exposure. The nuclei after 10% AuNP treatment showed 6.52 ± 0.63 percentage of DNA in tail which was higher than untreated control nuclei (0.95 ± 0.66 percentage of DNA in tail) and those treated with 5% AuNP suspension (1.04 ± 0.89 percentage of DNA in tail). Treatment of tomato leaf cells with 15 and 20% AuNP resulted in a slight rise in DNA damage showing 7.15 ± 1.70 and 13.47 ± 2.16 percentage of DNA in tail, showing significant apoptogenic effect (Fig. 8d). Thus, in lower doses apoptogenic effect is negligible holding the possibility for these nanoparticles to be used as antimicrobial agent or drug/gene delivery vehicle in eukaryotic cells.
The AuNPs are protein-coated
A few previous studies have mentioned nanoparticles coated with protein of natural origin [15]. Lower magnification TEM showed that the AuNPs are surrounded by protein-like material (Fig. 9a, b). In order to confirm the nature of the material, the AuNPs were washed and run on SDS-PAGE along with cell-free extracts in other lanes (Fig. 9c). Boiling in SDS served to detach the surface bound proteins from the nanoparticles. The boiled nanoparticles (lane 4) showed the presence of a single intense band of 40 kDa which was similar to a protein band present in lane 2 (cell filtrate). However, in the sample that was not boiled (lane 3), a faint protein band bound to AuNP was seen at the 116 kDa level. Although another faint band did appear at the 40 kDa level due to dissociation of the capping proteins from the particles. Protein coats are known to promote stability of nanoparticles in solution and their catalytic activity [28, 38]. The naturally formed protein coat around the nanoparticles makes them functionally efficient for biomedical uses including easy adsorption and delivery of DNA or hydrophobic drugs [39]. Peptides and protein-aided delivery of AuNPs have been successfully used to overcome blood-brain barrier in treatment of central nervous system disorders [14]. Hence, these biocompatible AuNPs can be potentially suitable for several biomedical applications due to the small size, unique physico-chemical properties, and other advantages.
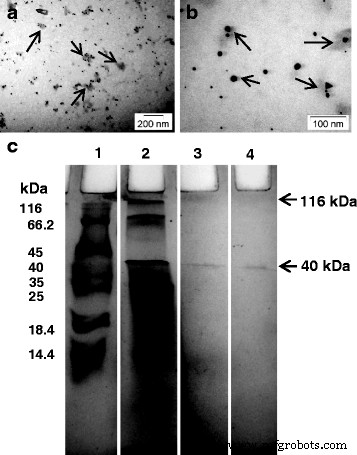
Protein cap analysis. 一 , b TEM images showing capping protein layer (arrows) around AuNPs. c SDS-PAGE of extracellular protein secreted from T. crassum and protein associated with nanoparticles. Lane 1, molecular size marker. Lane 2, total extracellular protein. Lane 3, nanoparticles loaded without boiling show faint protein band bound to the AuNPs at 116 kDa mark. There is also a band of detached protein at 40 kDa mark. Lane 4, nanoparticles after boiling with 1% SDS loading buffer showing disappearance of the 116 kDa band and a distinct 40 kDa band. Arrow indicates 40 kDa
图>The AuNPs could deliver green fluorescence protein (GFP) gene into Sarcoma 180 cancer cell lines
Plasmid DNA pCAMBIA1302 harboring gfp marker gene, complexed with AuNPs, was used to treat Sarcoma 180 cells. The cells produced green fluorescence indicating uptake of plasmid DNA/AuNPs complex and subsequent expression of the gene in the cancer cell, while the cells treated only with naked plasmid DNA did not show the fluorescence (Fig. 10a, b). This confirms the high potential of these particles to not only deliver genes into cancer cells, the genes were stably expressed and remained functional once delivered into the cells.
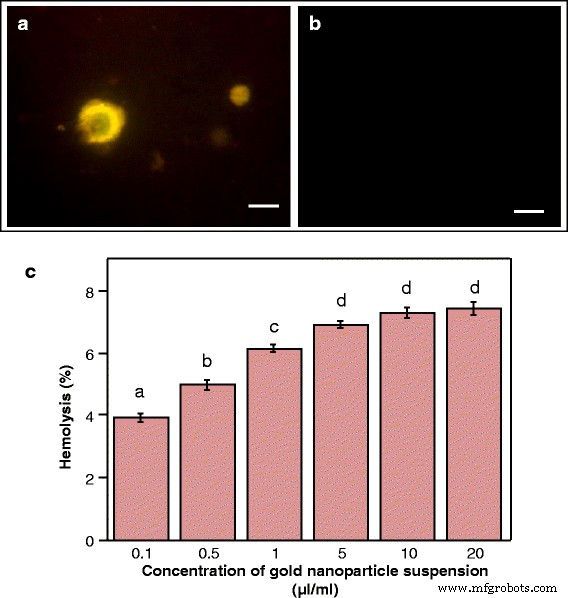
Gene delivery using AuNPs into Sarcoma 180 cancer cells and hemolysis assay with human erythrocytes; fluorescence microscopic image of a cancer cells expressing green fluorescence protein after uptake of DNA-AuNP complex and b control cells treated with free plasmid DNA (bars = 20 μm). c Percentage hemolysis with different dilutions of AuNPs. Data represents means ± SE of three replicates. Different letters indicate statistically significant differences among the samples (P < 0.05, Tukey’s HSD test)
图>Earlier metallic nanoparticles have been shown to exhibit immense therapeutic potential in treating variety of diseases like retinal neovascularization, HIV, Dalton’s lymphoma, and exhibited activity against hepatitis virus, respiratory syncytial virus, and herpes simplex virus [18]. Congruent to these reports, gold nanocarrier-based drug delivery in present study using AuNPs can be considered as a prospective mediator in numerous medical applications including diagnostics, drug delivery, and cancer therapeutics.
Compatibility of the AuNPs with human erythrocytes and toxicity assay
Erythrocytes are simple and convenient model of the cell membrane system and used for studying nanoparticle-membrane interactions [40]. The hemolytic assay elucidates membrane-lytic activity of the AuNPs at different concentrations. Figure 10c shows that membrane-lytic activity of the nanoparticles was negligible at low concentrations. The highest hemolytic activity found at higher concentrations of nanoparticle suspension (up to 20 μl/mL) was less than 8% which indicates very low blood toxicity. The gradually increasing hemolytic activity with increasing concentration of AuNPs is likely due to increased affinity and adhesion of larger number of particles with the erythrocytes. This affinity of the particles to cell membranes is expected to facilitate their cellular transport. Low hemolytic activity along with effective cellular uptake render nanoparticles highly suitable for the development of safe and efficient theranostic agents [41].
Conclusions
Use of edible mycorrhizal fungus to synthesize AuNPs of different geometric shapes in short reaction time with natural protein coat make this method simple and unique. The protein coat coming from the edible fungus did not have appreciable toxic effects and favor easy attachment of DNA onto the surface of the particles. Overall, these AuNPs show promise as antimicrobial, apoptotic agents for gene delivery into cancer cells.
Since filamentous fungi can withstand flow pressure or agitation [15], T. crassum can be cultured in fermentors to produce AuNPs on a large-scale using non-toxic agricultural wastes, allowing for easy withdrawal of product and system replenishing options [2]. Since the AuNPs are of different geometric shapes, there is the scope shape-based assortment with mechanical means such as centrifugation [42] and can be utilized according to their specific shape-based properties.
纳米材料
- 用于化学传感器的金纳米粒子
- 用于改进诊断和治疗应用的多功能金纳米粒子:综述
- 用于癌症治疗的纳米粒子:当前的进展和挑战
- 钴掺杂 FeMn2O4 尖晶石纳米粒子的制备和磁性
- 负载 ICA 的 mPEG-ICA 纳米颗粒的制备及其在治疗 LPS 诱导的 H9c2 细胞损伤中的应用
- Au 纳米颗粒对 HT29 和 SPEV 细胞系影响的体外研究
- 基于苯基三甲氧基硅烷改性氧化铝纳米颗粒的 Al2O3:SiOC 纳米复合材料的形成和发光特性
- 用 6-巯基嘌呤和神经元穿透肽修饰的金纳米颗粒促进 SH-SY5Y 细胞生长
- 桔梗皂苷(桔梗)用于金和银纳米颗粒的绿色合成
- 溶胶-凝胶法合成的 Pr 掺杂钙钛矿锰酸盐 La0.67Ca0.33MnO3 纳米颗粒的微观结构、磁性和光学性能
- 纳米颗粒的尺寸和聚集/团聚对聚合物纳米复合材料的界面/相间性质和拉伸强度的影响
- 从石榴皮提取物中合成的银纳米粒子的抗菌和细胞毒性作用