具有羟基依赖性的水溶性富勒烯醇,用于感染性微生物的有效双光子激发光动力灭活
摘要
我们成功制备了水溶性富勒烯醇 [C60(OH)46],其表现出高单线态氧量子产率并有效产生活性氧。此外,与具有较低此类基团组成的水溶性 C60(OH)46 相比,具有较高暴露羟基组成的水溶性 C60(OH)46 具有优异的双光子稳定性和特性。因此,制备的富勒烯醇可以作为一种有效的双光子光敏剂。水溶性 C60(OH)46 具有良好的双光子特性。在双光子光动力治疗期间,水溶性 C60(OH)46 对大肠杆菌 具有显着的抗菌活性 在 211.2 nJ pixel −1 的超低能级 800次扫描,光激发波长760 nm。
介绍
在过去的几十年里,已经合成了各种光敏剂 (PS) 分子 [1]。然而,现有PS的临床应用涉及几个问题。大多数 PS 分子是疏水的,很容易在水性介质中聚集,从而降低它们的量子产率 (QY) [2]。此外,聚合的 PSs 不能简单地静脉注射。还需要在死亡组织中选择性积累 PS 分子,以防止对健康细胞的损害。由于这些问题,开发有效的 PS 载体仍然是光动力疗法 (PDT) 的主要挑战。因此,使用纳米粒子作为 PS 载体的兴趣正在增加。
纳米生物技术的进步激发了人们对一类仅由碳原子组成的新型纳米结构 [3,4,5,6,7,8,9,10,11] 的生物医学应用的兴趣,即球状的富勒烯 C60分子(直径为 0.72 nm)无毒且具有独特的物理化学性质。亲脂性 C60 分子的小尺寸是它们与生物分子的空间相容性的原因,并促进它们整合到膜的疏水区域 [12, 13]。由于扩展的 π - 其分子轨道的共轭系统,富勒烯 C60 吸收紫外-可见光 (UV-vis) 光并能产生活性氧 (ROS),其单线态氧 QY (Φ) 接近 100% △)。此外,富勒烯 C60 的理化特性使其能够产生 ROS 并作为 PDT 的 PS。富勒烯也可能诱导促氧化作用,这可能取决于所使用的富勒烯、研究的细胞类型和实验设置 [14,15,16,17]。 C60在极性溶液中的溶解度极低,极大地限制了其在医学上的应用。然而,由于双键的存在,C60 可以很容易地使用化学基团进行改性,以增加其水溶性。因此,水溶性C60衍生物为医学应用增加了机会,包括神经保护、药物和基因递送、光敏化和生物传感。
多光子激光显微镜(也称为双光子激光显微镜)需要使用局部“非线性”激发来仅在薄光栅扫描平面内激发荧光。双光子激光显微镜已用于各种成像研究 [18]。它通常与近红外 (NIR) 激光激发相结合,以利用固有的最大组织传输进行生物成像;这是因为 NIR 具有轻微散射、低能量吸收、最佳辐射穿透和减少样品光漂白等优点。双光子激光显微镜与 NIR 激光激发的耦合已成为厚组织和更深生物标本中荧光显微镜的首选技术 [19, 20],并已广泛应用于其他光激发疗法 [21, 22]。此外,由于其超低能量和短光激发,双光子激光显微镜被认为是执行 PDT 的替代方法 [23]。虽然一些 PS 是有毒的 [24, 25],那些具有高 Φ Δ 优先进行 PDT。高Φ 当使用双光子技术评估光性质中的分子活性并有效地进行非线性显微研究时,Δ 值特别理想;这样的值是可取的,因为吸收的能量与输入到样品的能量通量之比很高,最大限度地减少了对样品的可能光损伤 [26]。然而,文献不包括考虑使用具有双光子特性的材料进行 PDT 的研究。为了填补这一研究空白,本研究应用了具有强给电子能力和大π的水溶性羟基化富勒烯醇。 -共轭系统提高电荷转移效率,从而增强双光子特性。具体而言,水溶性羟基化 C60(OH)46 被衍生并用作双光子 PS,使用超低能飞秒激光照射有效去除微生物,并且在双光子激发(TPE;激发波长,760 nm)下仅扫描 800 次.对于超低能量飞秒激光照射,能量为 211.2 nJ pixel −1 功率为2.112 mW(关于物镜后激光功率的计算,参见“材料与方法”部分和图1a,其中x –y 焦点和 z 激光系统的 - 轴分辨率分别约为 0.37538 和 0.90159 μm);此外,对于扫描过程,总有效曝光时间约为3.2621 s,扫描速率为4.0776 ms scan -1 , 扫描面积为 200 × 200 μm 2 (有关计算的详细信息,请参阅“材料和方法”部分)。水溶性羟基化 C60(OH)46 几乎 100% 消除大肠杆菌 (大肠杆菌 ,革兰氏阴性细菌菌株)。此外,在TPE下,与羟基组成较低的水溶性C60(OH)46相比,具有较高羟基组成的水溶性C60(OH)46表现出更好的双光子光性能;因此,衍生的水溶性C60(OH)46被认为具有相当大的潜力用于同步PDT以消除恶性微生物。
<图片>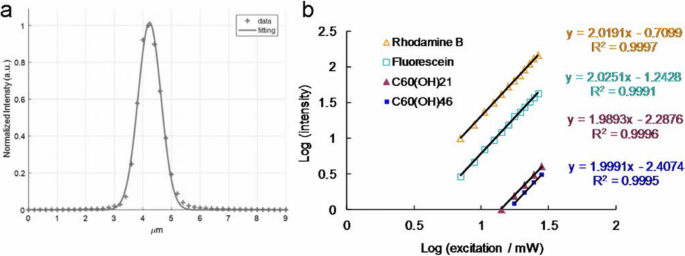
一 根据 z 用于测量不同位置二次谐波产生信号的金薄膜的轴扫描,z 激光系统的轴分辨率(半高全宽)约为 0.90159 μm(使用高斯函数拟合)。 b TPL 强度依赖于材料和荧光团的激发功率(对数); TPE 曝光从 704.0 到 2816.0 nJ pixel −1 对于罗丹明 B 和荧光素,从 1408.0 到 2816.0 nJ pixel −1 对于水溶性 C60(OH)21 富勒烯醇,从 1760.0 到 2816.0 nJ pixel -1 为水溶性 C60(OH)46 富勒烯醇。激发波长,760 nm。递送剂量:E的OD600 0.05。大肠杆菌 和 3 μg mL -1 材料。数据表示为平均值 ± SD (n =6)
图>材料和方法
水溶性富勒烯醇、C60(OH)21 和 C60(OH)46 [27]
的制备和表征粗富勒烯是从商业上获得的(Sigma-Aldrich,St. Louis,MO,USA),并且如前所述生产了 C60(OH)12 前体。首先,将30%的过氧化氢溶液(100 mL;Sigma-Aldrich, St. Louis, MO, USA)加入到起始原料中,0.5-1.0 g C60(OH)12,在60 °下剧烈搅拌该混合物C 在空气中。冷却后,将包含 2-丙醇、乙醚和己烷的溶剂混合物(每种 100–200 mL;Sigma-Aldrich, St. Louis, MO, USA)加入溶液中,随后离心并倾析。通过离心和倾析程序,用200 mL乙醚洗涤剩余的固体两次。最后,通过在室温下真空干燥残余物过夜,分别获得水溶性C60(OH)21和C60(OH)46的终产物。最终产品的重量通过热重分析校准。使用高分辨率透射电子显微镜(HR-TEM,JEOL 3010,Akishima,Tokyo,Japan)观察最终产品的形态,C60(OH)21 的分辨率约为 1.11 ± 0.03 nm 和 1.13 ± 0.04 nm和 C60(OH)46,分别。动态光散射(DLS,Malvern Nano-ZS90,Worcestershire,West Midlands,UK)也用于确定材料的尺寸。首先通过傅里叶变换红外(FTIR)光谱(RX1,PerkinElmer,Waltham,MA,USA)检查所制备材料的暴露官能团。使用光谱仪(U-4100,Hitachi,Chiyoda-ku,Tokyo,Japan)对材料进行紫外-可见光谱分析。通过X射线光电子能谱(XPS,PHI 5000光谱仪(VersaProbe,Chanhassen,MN,USA))检查富勒烯醇的表面化学。使用场解吸 (FD) 质谱仪 (AccuTOF, GCx-plus, JEOL, Akishima, Tokyo, Japan) 测定富勒烯醇的分子量,根据结果确认羟基数为 21 和 46,分别。
细菌培养 [28]
E。大肠杆菌 ,从我们自己的实验室获得,在 LB 营养琼脂中生长(每升:胰蛋白胨 10 g,酵母提取物 5 g,氯化钠 8 g,琼脂 15 g,pH 调至 7.5)(Sigma-Aldrich,圣路易斯, MO, USA) 并在 37 °C 下孵育。
菌落形成单位 (CFU) 计数法的生物相容性测定 [28]
E。大肠杆菌 (OD600 ~ 0.05) 加入材料 (0–9 μg mL -1 ),并在 37 °C 下孵育 3 小时(附加文件 1:图 S1)。温育后,将混合物离心并稀释细菌沉淀(OD600~ 0.05)。稀释系数为 10 −5 到 10 −8 然后在培养的细菌中进行,并铺在琼脂平板上。将板留在培养箱中(37 °C)过夜。测定存活菌数,以百分比(%)表示,对应单位CFU mL -1 孵化后。数据为平均值 ± SD (n =6).
ψ Δ 测量 [29, 30]
根据之前的研究,ψ Δ 可得。 ψ Δ 测量在 D2O 中进行,波长为 355 nm,使用 meso -四(4-磺基苯基)卟吩二盐酸盐(TSPP;Sigma-Aldrich, St. Louis, MO, USA)作为参考(ψ Δ =0.64).
荧光 QY 测量 [31, 32]
造影剂的相对光致发光 (PL) QY 通常是发射光子与吸收光子的比值,其公式如下:
$$ \mathrm{QY}={\mathrm{QY}}_{\mathrm{ref}}\ \left({\eta}^2/{\eta_{\mathrm{ref}}}^2\right) \left(I/A\right)\left({A}_{\mathrm{ref}}/{I}_{\mathrm{ref}}\right) $$ (1)其中 QYref =0.28 是溶解在二甲基亚砜(DMSO;Sigma-Aldrich,St. Louis,MO,USA)中作为参考的 Cy5.5 的 QY,η 是 ddH2O =1.33 (η DMSO 的参考值 =1.48), I 是积分荧光强度和A 是激发波长处的吸光度。单光子激发 (OPE) 或 TPE 产生相同的 QY。
用于测量双光子吸收 (TPA) 和双光子发光 (TPL) 的飞秒激光光学系统 [23, 28, 33,34,35 ,36,37,38]
根据之前的研究,使用自制的飞秒钛蓝宝石(ti-sa)激光光学系统(重复频率为80 MHz;Tsunami,Spectra-Physics,Santa Clara,CA,USA)。
TPA 衡量
振镜扫描仪速度为 2 m ms −1 ,激发光谱测量为 720-820 nm,激发功率为 2.8 mW [这是目标前的功率;目标后(或样本上)的功率为 0.9856 mW 或 98.56 nJ pixel −1 ]。因此,测量了作为富勒烯醇激发波长函数的相对 TPA 光谱。
TPL 光谱的测量
将材料暴露于来自飞秒激光的 TPE,激发波长为 760 nm,扫描面积为 200 × 200 μm 2 , 频率为 10 kHz, 曝光时间为 1.638 s/(scan, pixel) =100 μs, 128 × 128 pixels scan −1 , 像素面积为 1562.5 × 1562.5 nm 2 .焦斑面积计算为 πd 2 /4,其中 d =0.61 λ/数值孔径 (NA ) 是束腰的半高全宽。例如,在 x –y 轴焦斑具有 760 nm 激发和 × 40 油浸物镜,具有 NA 1.3,d =0.61 × 800 nm/1.3 =375.38 nm =0.37538 μm,z 测量的 - 轴分辨率为 0.90159 μm。对于 760 nm 激发,单个纳米材料每次扫描的曝光时间表示为(焦点面积/像素面积)×100 =4.0776 ms,总曝光时间 t =4.0776 ms × 扫描次数。 × 40 油浸物镜 (NA 1.3)采集信号,光谱光度计检测范围为300~695 nm。
此外,激光功率的计算(mW 或 nJ pixel −1 ) 用于样品如下。对于 × 40 油浸物镜 (NA 1.3),该光学系统在波长760 nm处的透射率约为88%,由于功率损失,激光功率从输出到物镜只有原始输出功率的40%。因此,目标(在样品上)后计算的能量为 P 输出 (mW)*40%*88% =0.352 × P 输出(毫瓦)。例如,P output =2.8 mW,目标后(在样品上)的计算能量为 3.0 mW*40%*88% =0.9856 mW。以 10 kHz 的扫描速率(每个脉冲保持 0.1 ms pixel −1 ),样本上的计算能量 (J pixel −1 ) 在 P 附近 输出 (mW)*40%*88%*0.1 ms =0.0352*P 输出 (J pixel −1 )。例如,P 输出 =2.8 mW,能量 (J pixel −1 ) 样本 =2.8 mW*40%*88%*0.1 ms =0.09856 μJ pixel −1 =98.56 nJ 像素 −1 .使用物镜(在样品上)后的功率并标记了本手稿的吞吐量。
TPE 绝对横截面的测量 [24, 36,37,38,39,40,41,42,43,44,45,46,47, 48]
TPE的绝对横截面是通过via的发光信号来测量的 根据以往的研究,飞秒激光光学系统。必须验证荧光素和罗丹明 B(Sigma-Aldrich,St. Louis,MO,USA)的 TPL。结果如图1b所示,是通过在704 nJ pixel -1 的激发功率范围内测量发射强度的依赖性而获得的 (7.04 mW) 到 2816 nJ pixel −1 (28.16 mW)。测量荧光素指数为 2.03 和罗丹明 B 指数为 2.02 的二次相关性,以增加激发功率以确定 TPE 的发光。根据之前的研究,TPE对荧光素和罗丹明B的作用截面分别为36.4和68.0 GM(1 GM =10 -50 厘米 4 s 光子 −1 ),分别用于 760 nm 激发。我们还参考了免费网站 http://www.drbio.cornell.edu/cross_sections.html,由 Chris Xu 教授(美国纽约州康奈尔大学)友情提供。经计算,荧光素和罗丹明 B 的 TPE 作用截面分别为 36.5 和 66.1 GM(表 1),与徐教授实验室的误差小于 5%。在本研究中,选择罗丹明 B 作为确定横截面的标准参考,计算出的水溶性 C60(OH)21 和 C60(OH)46 富勒烯醇的 TPE 绝对横截面约为 1230.51 GM 和 1037.21 GM , 分别。计算样品TPE绝对截面的实测参数如表3所示。材料的双光子性能和双光子光动力能力未观察到批次间变化。
图>飞秒激光光学系统(用于荧光寿命成像显微镜,FLIM)[39, 45]
根据之前的研究,使用自制的飞秒 ti-sa 激光光学系统(重复频率为 80 MHz;Tsunami,Spectra-Physics,Santa Clara,CA,USA)。使用三重指数方程拟合生成寿命数据和参数,同时监测 TPE (Ex, 760 nm) 下的发射。
计算辐射和非辐射衰减率 [46]
在研究不同环境中荧光染料的发射特性时,PL QY 和寿命都是主要参数。 QY (Q ) 可以表示为:
$$ Q=\frac{\varGamma }{\varGamma +k} $$ (2)其中 Γ 是辐射衰减率,k 是非辐射衰减率。荧光寿命通常定义为处于激发态的电子衰变为基态所需的平均时间。 TPL 生命周期 τ 也可以与衰减率相关,描述如下:
$$ \tau =\frac{1}{\varGamma +k} $$ (3)遵循方程。 (2)和(3)可以计算出辐射衰减率和非辐射衰减率。
在吸收光子后,荧光分子的弱结合电子之一——荧光团——被提升到更高的能级。然后荧光团处于激发态,A* .这种状态是亚稳态的;因此,荧光团将返回其稳定的基态,A .它可以通过发射荧光光子 hν
$$ A\ast ->A+ h\nu $$或通过将激发态能量以热的形式耗散:
$$ A\ast ->A+\mathrm{heat} $$激发态的减少取决于可用的去激发途径。荧光是第一个电子激发单线态 S 的最低振动能级的辐射失活 1 ,回到电子基态,S 0 .单线态是可以由弱束缚电子在没有自旋翻转的情况下填充的能级。吸收和发射过程由以 Aleksander Jablonski 命名的能级图说明。
荧光寿命,τ , 是荧光团保持在电子激发态 S 的平均时间 1 激发后。 τ 被定义为所有激发态人口减少过程的速率参数总和的倒数:等式。 (3),其中非辐射率常数k 是内部转换速率常数的总和 k ic 和系统间穿越到三重态 k 的速率常数 isc 使得 k =k ic + k 岛。荧光发射总是发生在 S 的最低振动能级 1,一条被称为 Kasha 规则的规则,表明荧光团对其激发途径没有记忆;例如,OPE和TPE产生相同的荧光光谱、QY和寿命。
激光照射后细菌存活率的测定 [28]
CFU 计数方法
将细菌 (OD600 ~ 0.05) 与材料 (3 或 6 μg mL -1 ) 在 37 °C 黑暗中孵育 3 小时。孵育后,将混合物离心并稀释细菌沉淀(OD600 ~ 0.05)并暴露于 211.2 nJ pixel -1 800 次扫描(大约 3.2621 s 的总有效曝光时间;例如,760 nm)。然后,稀释系数为 10 −5 到 10 −8 然后在培养的细菌中进行,并铺在琼脂平板上。将板留在培养箱中(37 °C)过夜。测定存活菌数,以百分比(%)表示,对应单位CFU mL -1 孵化后。数据为平均值 ± SD (n =6).
LIVE/DEAD Kit
将细菌 (OD600 ~ 0.05) 与材料 (3 或 6 μg mL -1 ) 在 37 °C 黑暗中孵育 3 小时。孵育后,将混合物离心并稀释细菌沉淀(OD600 ~ 0.05)并暴露于 211.2 nJ pixel -1 800 次扫描(大约 3.2621 s 的总有效曝光时间;例如,760 nm)。然后,使用 LIVE(SYTO 9,显示为绿色荧光)/DEAD(碘化丙啶, PI,显示为红色荧光)试剂盒(Thermo Fisher Scientific,Waltham,MA,USA)根据说明。细菌的生存能力通过抗菌测试进行量化,结果表明几乎所有经纳米材料处理的细菌在处理后都已死亡。通过 CFU 计数方法量化类似的活力,以确定材料在 PDT 中的有效抗菌作用。数据表示为平均值 ± SD (n =6).
ROS 检测 [23, 29, 34, 35, 49,50,51,52,53,54,55]
单线态氧 ( 1 O2)
(a) 材料(3 或 6 μg mL −1 ) 用细菌 (OD600 ~ 0.05) 处理,然后在 37 °C 黑暗中孵育 3 小时。随后,将混合物暴露于 TPE 光激发(211.2 nJ pixel -1 , 800 次扫描; Ex, 760 nm),最后与 Singlet Oxygen Sensor Green (SOSG) 试剂(1 μM;Thermo Fisher Scientific,Waltham,MA,USA)混合(Ex/Em:488/525 nm)。使用荧光光谱仪进行测量。为了中和 ROS,将混合物与 30 ppm 的抗氧化剂 α 混合 -生育酚/亚油酸甲酯(Sigma-Aldrich,St. Louis,MO,USA)在黑暗中并暴露于具有相同处理的 TPE 光激发。 (b) 材料(3 或 6 μg mL −1 ) 用细菌 (OD600 ~ 0.05) 处理,然后在 37 °C 黑暗中孵育 3 小时。随后,将混合物暴露于 TPE 光激发(211.2 nJ pixel -1 , 800 次扫描;例如,760 nm),最后与 10 μM 的反式-1-(2'-甲氧基乙烯基)芘(t -MVP, Thermo Fisher Scientific, Waltham, MA, USA)/0.10 M SDS(Sigma-Aldrich, St. Louis, MO, USA)(Ex/Em:352/465 nm)。为了中和 ROS,将混合物与 30 ppm 的抗氧化剂 α 混合 -生育酚/亚油酸甲酯(Sigma-Aldrich,St. Louis,MO,USA)在黑暗中。 t 的反应 -MVP with 1 O2 生成二氧杂环丁烷中间体,当它分解成 1-芘甲醛时会发出荧光。此外,这种高度选择性的荧光探针不会与其他活性氧物质(如羟基自由基、超氧化物或过氧化氢)发生反应。使用荧光光谱仪进行测量。 ROS中和的处理方法与前面描述的相同。
超氧化物自由基阴离子 (O2 .− )
(a) 材料(3 或 6 μg mL −1 ) 用细菌 (OD600 ~ 0.05) 处理,然后在 37 °C 黑暗中孵育 3 小时。随后,将混合物暴露于 TPE 光激发(211.2 nJ pixel -1 , 800 次扫描;例如,760 nm),最后与 2, 3-双(2-甲氧基-4-硝基-5-磺苯基)-2H-四唑鎓-5-甲酰苯胺(XTT,0.45 mM;Sigma-Aldrich,圣路易斯,密苏里州)混合, 美国)。该材料的目的是与 O2 相互作用。 - 并产生 XTT-甲臜,导致强吸收(波长为 470 nm)。紫外-可见光谱仪被用来监测这种吸收。为了中和 ROS,将混合物与 30 ppm 的抗氧化剂 α 混合 -生育酚/亚油酸甲酯(Sigma-Aldrich,St. Louis,MO,USA)在黑暗中并暴露于具有相同处理的 TPE 光激发。 (b) 材料(3 或 6 μg mL −1 ) 用细菌 (OD600 ~ 0.05) 处理,然后在 37 °C 黑暗中孵育 3 小时。随后,将混合物暴露于 TPE 光激发(211.2 nJ pixel -1 , 800 次扫描;例如,760 nm),最后与 50 mM 碳酸氢盐缓冲液(pH 8.60)和谷胱甘肽(γ -l-glutamyl-l-cysteinyl-glycine, GSH, Sigma-Aldrich, St. Louis, MO, USA)/0.80 mM 碳酸氢盐缓冲液(Ellman 检测 O2 。 - 检测)。随后,根据先前研究中的程序进行了以下实验。 GSH 的损失 (%) 计算为样品和阴性对照之间的吸光度差异除以阴性对照的吸光度。生成的O2信号 . - 如前面的计算中所述获得。数据为平均值 ± SD (n =6).
摄取测定 [35]
E。大肠杆菌 (OD600 ~ 0.05) 与 3 μg mL -1 材料。吸光度为3 μg mL -1 通过紫外-可见光谱(Abs,大约 203 nm)记录材料。材料与E混合。大肠杆菌 (OD600 ~ 0.05)从第1小时到第10小时,分别在37 °C下,离心(1200 rpm)去除多余物质并保留上清液并测量其吸光度。估计收集的上清液和原始材料之间的吸光度差异,从而得出每个时间点的吸收百分比。数据为平均值 ± SD (n =6).
统计分析 [56]
统计显着性是通过方差分析。 p 所有处理均认为该值具有统计学意义。
结果与讨论
水溶性富勒烯醇的表征
根据先前的研究 [27] 合成了水溶性 C60(OH)46(富勒烯醇),它被确定为圆形和单分散的。使用低放大倍数(图 2a)和 HR-TEM 图像(图 2b)测定的富勒烯醇的平均横向尺寸约为 1.13 ± 0.04 nm。此外,富勒烯醇表现出良好的结晶度和良好的晶格间距,这对应于 d - 富勒烯醇 {1\( \overline{1} \)00} 晶格条纹的间距。然而,这些颗粒在 pH 值为 7.0 的水溶液中可以通过氢键形成聚集体。 DLS 分析显示,形成的聚集体的平均尺寸约为 130 nm。此外,聚集体在不同的生理环境中保持高度稳定 3 个月,例如 pH 7.0 水溶液、1×磷酸盐缓冲盐水和培养基(附加文件 1:表 S1)。在富勒烯醇的紫外可见吸收光谱中,在大约 216 和 309 nm 处观察到吸收峰,这些峰归因于 π –π * 芳香族 C=C 键和 n 的转变 –π * C=O 肩部的过渡,分别。 π 富勒烯醇中的 - 电子跃迁包含氧(图 2c),正如通常在水分散体中观察到的那样,从而证实了富勒烯醇的存在。使用 FTIR、XPS 和质谱法进行了额外的表征,以确认所制备材料的特性。 FTIR 用于分析制备材料的暴露官能团。分析结果揭示了以下特征材料带: 在大约 1109 cm -1 处的 C-O 伸缩带 (波段 1),酚类 C-OH 伸缩带在大约 1271 cm -1 (波段 2),叔醇 C=O 伸缩带在大约 1422 cm −1 (波段 3),C=C 伸缩带在大约 1674 cm −1 (波段 4),C=O 伸缩带在大约 1721 cm −1 (带 5),以及 C-H 分子间氢键和羧酸盐 O-H 伸缩带,大约在 3318 cm -1 (波段 6)。此外,观察到 CO2 干扰带。这些带显示暴露的羟基和羰基以及芳香 C=C 键(图 2d)。进行 XPS 以检查富勒烯醇的表面化学,它通常主要包含碳原子。富勒烯醇的解卷积 C(1s) 光谱揭示了一个非氧化环 (C–C/C=C, 286.1 eV)、C-O 键 (286.9 eV) 和 C=O 键 (288.0 eV)。此外,O(1s)/C(1s) 比率约为 35.8%(图 2e)。还使用 FD 质谱法测定了富勒烯醇的分子量(附加文件 1:图 S2);羟基数(C-OH)为46个,与图2中富勒烯醇的原子比和键组成一致。这些表征结果证实了富勒烯醇的成功合成。
<图片>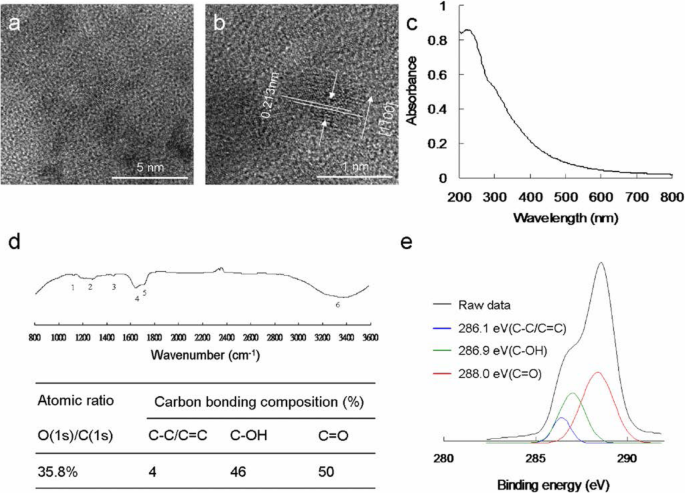
合成的水溶性 C60(OH)46 富勒烯醇的功能表征。 一 低倍 TEM 图像和 b 水溶性富勒烯醇的 HR-TEM 图像说明材料 {1\( \overline{1} \)00} 晶面和 1.11 ± 0.03 nm 的平均尺寸,d -间距为 0.213 nm。 c UV–vis and d FTIR spectra of nanomaterial. e Deconvoluted C(1s) XPS spectra and fitted peaks obtained using Gaussian function:nonoxygenated ring (C–C/C=C), C–O bond, and C=O bond, respectively. The atomic ratio and bonding composition of fullerenol are shown as summarized in the table. The O(1s)/C(1s) atomic ratio is 35.8%
图>ROS Generation of Water-Soluble Fullerenol Under TPE
A PS absorbs and transfers light energy to other nonabsorbing molecules to generate ROS, which kill targeted cells, damage tumor vasculature, and activate an antitumor immune response. PSs have a particular arrangement of electrons in their molecular orbitals. Similar to nearly all molecules, at ground (singlet) state, PSs have couples of electrons with opposite spins in low-energy molecular orbitals. The absorption of light at an appropriate wavelength lifts an electron to a high-energy orbital without changing its spin. This is a short-lived (nanoseconds) excited singlet (S1) state, and the PS can lose its energy and return to the ground state by emitting light (fluorescence) or heat. Alternatively, intersystem crossing, wherein the spin of the excited electron is inverted, can occur in the S1 state. This electron spin inversion is responsible for the relatively long life (lasting microseconds) of the excited triplet (T1) state. Radiative triplet-to-singlet transitions are inhibited because they require a change in electron spin, which is a slow process. From the T1 state, the PS can return to the ground state by emitting light (phosphorescence) or transferring energy to another molecule. It can also lose energy through internal conversion or radiationless transitions when colliding with other molecules. The longer the life of the PS in the T1 state is, the higher are its chances of colliding with another molecule, resulting in ROS production [57,58,59]. The photosensitization of water-soluble fullerenols results in their transition to a long-lived T1 state and subsequent energy or electron transfer to molecular oxygen, yielding ROS such as 1 O2 and O2 . - , which have major roles in PDT. Therefore, 1 O2 and O2 . - produced by water-soluble C60(OH)46 must be detected directly using laser irradiation. To detect 1 O2 and O2 . - formation during PDT, in this study, PDT was initiated by combining excited the triplet water-soluble C60(OH)46, oxygen, and light configured to a suitable wavelength and energy as well as by introducing SOSG, t -MVP, XTT, and GSH reagents [33, 34, 49,50,51]. To exploit the potential bactericidal capability of the materials, a wavelength of approximately 760 nm was determined to be the most efficient for deriving the relative maximum TPA ratio of the water-soluble C60(OH)46 under TPE (Fig. 3a); this is attributable to the interband transitions involved [52]. This wavelength was used in subsequent experiments in this study. The water-soluble C60(OH)46 was photoexcited through TPE at a power of 211.2 nJ pixel −1 with 800 scans (Ex, 760 nm; total effective exposure time, ~ 3.2621 s) and delivered dose of 3 or 6 μg mL −1 (Additional file 1:Table S2). Furthermore, to confirm the involvement of ROS in the PDT effects of the water-soluble C60(OH)46, α -tocopherol was used for ROS neutralization [49, 53]. The quantity of generated ROS was reduced after the addition of α -tocopherol, but the observed bacterial viability increased as expected. Additionally, the quantity of generated ROS depended on the delivered dose. To prevent 1 O2 and O2 . - production possibly engendered by inadvertent exposure of water-soluble C60(OH)46 to white light—which could have compromised the experiments in this study [60]—subsequent PDT experiments were conducted in the dark. This study focused on the quantities of generated 1 O2 and O2 . - . The water-soluble C60(OH)46 exhibited considerable antibacterial effects, demonstrating its potential for application in PDT. Notably, after the same experiment, the water-soluble C60(OH)21 (Additional file 1:Figs. S3, S4; Fig. 3a) was less effective in forming 1 O2 and O2 . - when compared with the water-soluble C60(OH)46 (Additional file 1:Table S2). The water-soluble C60(OH)46 generated more 1 O2 and O2 . - than did the water-soluble C60(OH)21; additionally, the water-soluble C60(OH)46 and water-soluble C60(OH)21 had Φ Δ values of approximately 0.93 and 0.85, respectively (for reference, Φ Δ =0.64 is the QY of TSPP dissolved in D2O [29, 30]).
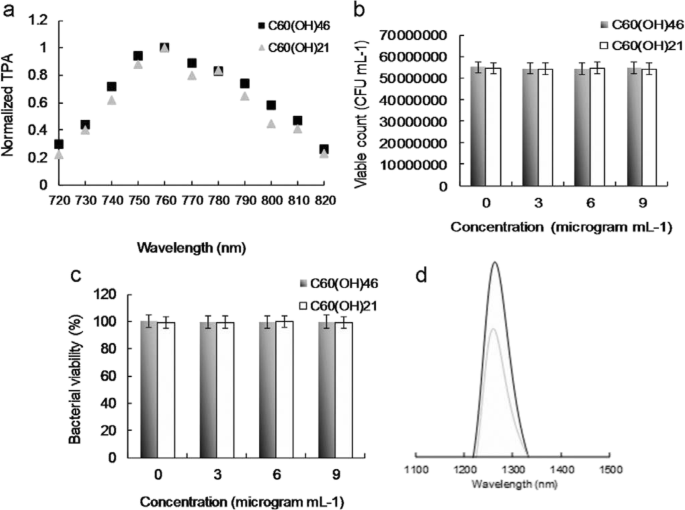
一 Relative TPA spectra of the material. TPE as a function of the wavelength (720–820 nm) at 98.56 nJ pixel −1 that was used to monitor the signals. Delivered dose, 3 μg mL −1 water-soluble C60(OH)46 or C60(OH)21 fullerenol. The number of surviving b material-treated bacteria was determined by CFU counting assay and is expressed as the percentage (%) for c bacteria that corresponds to the unit of CFU mL −1 . Delivered dose, OD600 ~ 0.05 of E.大肠杆菌 and 0–9 μg mL −1 water-soluble fullerenol. d Measurement of phosphorescence spectra at 1270 nm for material. Delivered dose, 3 μg mL −1 water-soluble fullerenol. Data are means ± SD (n =6)
图>Antimicrobial Ability Determination Using TPE
Before the execution of antimicrobial experiments, the toxicity of water-soluble fullerenols must be examined to exclude factors that could contribute to bacterial elimination and confound experimental results. In addition, to prevent possible ROS production engendered by the inadvertent exposure of experimental materials to white light, which could confound experimental results [35], PDT experiments must be conducted in the dark. This study applied Gram-negative E.大肠杆菌 as the experimental template. A CFU counting assay was conducted to determine the number of surviving bacteria (expressed herein as a percentage, corresponding to CFU mL −1 )。 The bacteria were treated with two types of the prepared water-soluble fullerenols (dose range, 0 to 9 μg mL −1 ) and incubated in the dark for 3 h at 37 °C to determine absorbance at 600 nm (OD600 ~ 0.05; Additional file 1:Fig. S1). The growth levels of the bacteria treated with the water-soluble fullerenols were first monitored by measuring absorbance at 600 nm. The initial absorbance was 0.05 OD600, and the absorbance associated with both materials reached approximately 0.37 over time. Accordingly, neither material inhibited bacterial proferation. Moreover, the materials engendered a nearly 0 log10 reduction in the number of surviving bacteria (Fig. 3b), corresponding to a viability of approximately 100% (Fig. 3c). Accordingly, the materials were determined to exhibit excellent biocompatibility with the bacteria. Consequently, the materials subjected to 3 h of incubation in the dark at 37 °C were used to conduct experiments. Although the water-soluble fullerenol could generate ROS, interactions between materials and reagents (i.e., SOSG, t -MVP, XTT, and GSH) may result in false-positive ROS signals, thereby confounding PDT results [52]. Therefore, to exclude this possibility, bacteria were introduced and treated with materials in the present study. The amount of ROS generated from the photoexcited material-treated E.大肠杆菌 was observed. Table 2 presents the observed amount of ROS, revealing a similar trend to that in Tables S2–S3 (Additional file 1:materials alone and material-treated-Gram-positive Bacillus subtilis (B. subtilis )); these results were consistent with the 1 O2 phosphorescence signal emitted from the materials at 1270 nm (Fig. 3d). PDT against E.大肠杆菌 was performed using irradiation with a low dose of energy (211.2 nJ pixel −1 with 800 scans, total effective exposure time ~ 3.2621 s; Ex, 760 nm). The effects PDT on the viability of E.大肠杆菌 treated with two-photon photoexcited materials were then determined (Fig. 4). No bactericidal effects were observed on bacteria alone (with or without laser exposure) or on the panel of material-treated bacteria without laser treatment (Fig. 4a). After TPE, bacterial viability was relatively low; specifically, the viability observed for the panel that was treated with the water-soluble C60(OH)21 was nearly 15%, corresponding to an approximately 0.823 log10 reduction (Fig. 4b). By contrast, the bacterial viability observed for the panel treated with the water-soluble C60(OH)46 was approximately 0 (100% elimination efficiency, corresponding to a ~ 7.736 log10 reduction). When the dose was increased, complete bactericidal effects were observed for both materials (Fig. 4c, d). However, antimicrobial effects did not differ by bacteria type (Gram-negative E. coli or Gram-positive B. subtilis ) after photoexcitation (Additional file 1:Fig. S5). In addition, regarding the fullerenols that eliminated bacteria, a higher composition of hydroxyl groups increased bactericidal capability when compared with a lower composition under identical treatment conditions.
图>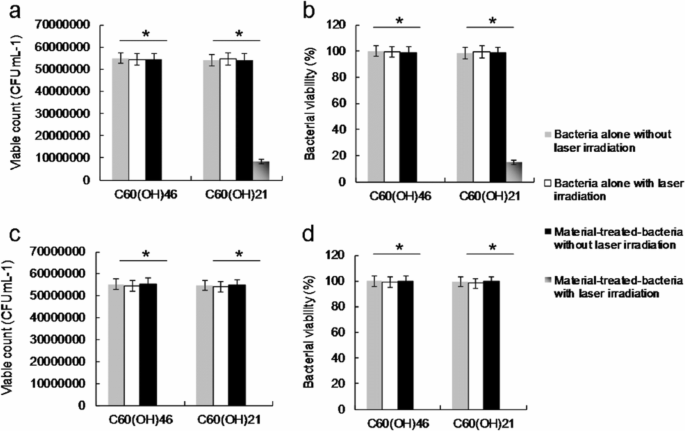
Viability (%) was quantified according to the determined viable count of material-treated bacteria through a CFU assay conducted using short excitation with a TPE power of 211.2 nJ pixel −1 with 800 scans (approximately 3.2621 s of total effective exposure time; Ex, 760 nm) to deliver a dose of a , b 3 or c , d 6 μg mL −1 . Delivered dose, OD600 0.05 of E.大肠杆菌 . Data are presented as means ± SD (n =6). For C60(OH)46- and C60(OH)21-treated E.大肠杆菌 with photoexcitation, a p <0.001 and p =0.662, b p <0.001 and p =0.658, c p <0.001 and p <0.001, and d p <0.001 and p <0.001. *p value obtained by Student’s t test
图>Observation of Water-Soluble Fullerenol-Treated E.大肠杆菌 Using TEM and Investigation of Two-Photon Properties
To observe the disruption of material-treated bacteria after photoexcitation, the water-soluble C60(OH)46 with high PDT efficiency was selected, and bacteria were imaged using TEM. Bare E.大肠杆菌 (Fig. 5a) were incubated with the water-soluble fullerenol for 3 h, resulting in the substantial adsorption of materials on the bacterial surfaces. Nevertheless, no unusual morphologies were observed, indicating normal live bacterial morphology (Fig. 5b). Uptake assay results revealed the adsorption of materials onto the bacterial surface, with the corresponding burst rate being approximately 85% within the first 3 h of incubation (Fig. 5c); the rate reached saturation from the 3rd to the 10th hour. Therefore, the materials were adsorbed and formed an external barrier on the bacterial surface. However, the E.大肠杆菌 exhibited a distorted appearance and severe morphological changes over 3 days of incubation (Fig. 5d), resulting in a 0.940 log10 reduction that corresponded to a nearly 18% viability (Fig.5f; Additional file 1:Fig. S6). Material absorption and coating on the bacterial surface suppressed the absorption of nutrients essential for microbial growth and engendered changes in membrane (wall) permeability, thereby inducing internal osmotic imbalances and inhibiting microbial growth. In other words, the water-soluble fullerenol had antibacterial (bacteriostatic or bactericidal) effects after 3 days of incubation. Furthermore, the photoexcited material-treated bacteria, particularly E.大肠杆菌 , exhibited unique morphologies with severe damage after 3 h of incubation (Fig. 5e, f; Additional file 1:Fig. S6). No heat-generated bubbles formed on the bacterial surface incurred damage, indicating that the water-soluble fullerenol did not have photothermal-mediated heat properties after photoexcitation (Additional file 1:Fig. S7). The viability of E.大肠杆菌 was also determined through fluorescence and quantification (Fig. 6). The green fluorescence indicative of living bacteria in Fig. 6a reveals that the bacteria exposed to laser treatment alone were largely undamaged, which is consistent with the results presented in Fig. 5a. Dead bacteria were detectable after treatment with the materials and laser exposure (red fluorescence in Fig. 6b), a finding that is also consistent with that in Fig. 5e. Bacterial viability was quantified for further antimicrobial testing. Nearly complete elimination of the material-treated bacteria (Fig. 6c) was observed. Viability was also quantified using a CFU assay (Figs. 4a, b and 5f, and Additional file 1:Fig. S6) to demonstrate the antibacterial efficiency of the water-soluble C60(OH)46 in PDT. According to the results in Figs. 4, 5, and 6; Table 2; and Table S2 (Additional file 1), E.大肠杆菌 treated with the water-soluble C60(OH)46 was susceptible to photoexcitation, leading to a higher death rate, increased ROS generation, and more severe morphological collapse compared with E.大肠杆菌 treated with the water-soluble C60(OH)21. In general, the absolute cross section for TPE makes fluorophores efficient for nonlinear microscopic studies because the ratio of the energy absorbed to the input energy flux to a specimen is high, thereby minimizing possible photodamage to specimens [39, 40]. When two-photon techniques are used to image molecular activities in living biological preparations and turbid tissues, a favorable cross section is desirable [61]. In the present study, the absolute cross section for TPE calculated for the water-soluble C60(OH)46 was approximately 1037 GM (Goeppert-Mayer unit, with 1 GM =10 −50 cm 4 s photon −1 ) at a 760-nm excitation wavelength (fluorescein was the standard reference for the cross section [39, 40]; Fig. 1b and Tables 1 and 3); the absolute cross section calculated for the water-soluble C60(OH)21 was approximately 1230 GM, which is similar to values obtained in relevant studies [62, 63]. These absolute cross sections could facilitate the two-photon process. Moreover, the fluorescence of the water-soluble C60(OH)46 was illuminated through a two-photon process (Fig. 1b). The relative fluorescence QY was approximately 0.02 (the QY of Cy5.5 in dimethyl sulfoxide [31] served as a reference:QYref =0.28); similarly, the absolute QY [64] was approximately 0.01, and the same QYs were derived for one-photon excitation and TPE [31]. By contrast, the water-soluble C60(OH)21 had lower relative and absolute QYs (0.06 and 0.05, respectively). In addition, this study investigated the lifetime of the fullerenols. The effects of radiative and nonradiative decay rates on QY and lifetime were calculated. The average lifetime of the water-soluble C60(OH)46 was approximately 7.797 ns, as calculated from observed lifetimes of 0.149, 1.775, and 19.679 ns; the average lifetime of the water-soluble C60(OH)21 was approximately 5.251 ns (Fig. 7 and Table 4). Therefore, the ratio of radiative to nonradiative decay rates of the water-soluble C60(OH)46 was approximately0.020 (derived from rates of approximately 2.565 × 10 6 s −1 to 1.257 × 10 8 s −1 ), whereas that of the water-soluble C60(OH)21 was approximately 0.064 (approximately 1.143 × 10 7 s −1 and 1.790 × 10 8 s −1 ; Additional file 1:Table S4). This finding is attributable to the existence of a hydroxyl group on the surface of the water-soluble fullerenol, which induced the nonradiative recombination of electron–hole pairs, leading to the inhibition of intrinsic state emission. However, hydroxylgroups at the edge of the water-soluble fullerenol may have a high occupied molecular orbital. This can be attributed to the strong orbital interaction between hydroxyl groups, which could thus increase the efficiency of intersystem crossing (rather than fluorescence generation) and generate numerous nanomaterial triplets with a high composition of hydroxyl groups; therefore, this would result in a high Φ Δ value for the water-soluble fullerenol and induce the fullerenol to react with oxygen according to the Jablonski diagram [65]. Consequently, two-photon PDT can be effectively performed using ultralow energy in an extremely short time, thereby providing an alternative approach to killing malignant species.
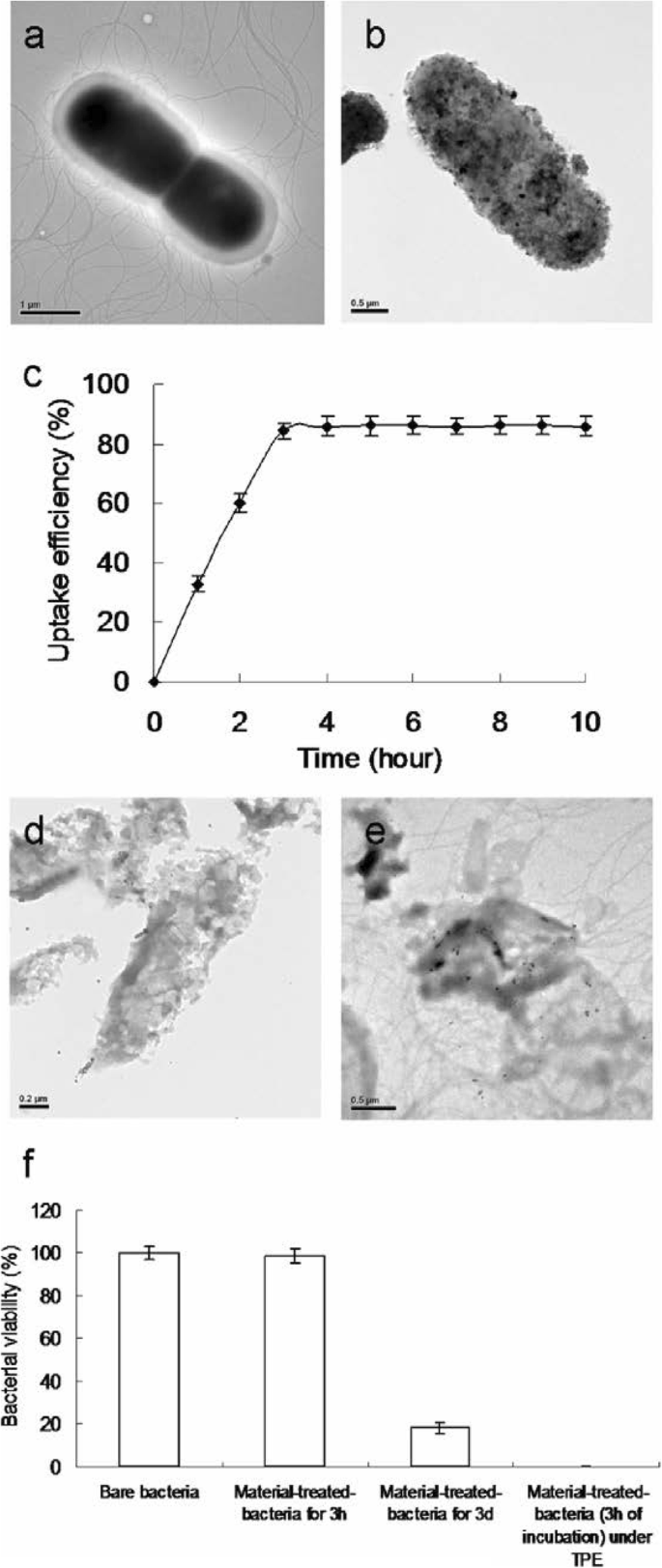
TEM images. 一 Showing bare bacteria without any treatment. Bacteria treated with material for b 3 h and d 3 days of incubation. e The photoexcited material-treated bacteria (3 h of incubation) with a TPE power of 211.2 nJ pixel −1 with 800 scans (approximately 3.2621 s of total effective exposure time; Ex, 760 nm). c Uptake assay of bacteria and material at 37 °C. f Viability (%) was quantified following the determined viable count of material-treated bacteria via CFU assay by short excitation with the same treatment. Delivered dose OD600 ~ 0.05 of E.大肠杆菌 and 3 μg mL −1 water-soluble fullerenol C60(OH)46. Data are means ± SD (n =6)
图>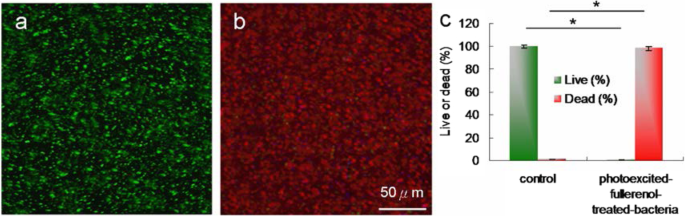
Images obtained after laser photoexcitation exposure (211.2 nJ pixel −1 ) with 800 scans (approximately 3.2621 s of total effective exposure time; Ex, 760 nm) of a , b material-treated bacteria. The Live/Dead kit was used to stain bacteria before images were obtained. Scale bar, 50 μm. c Viability (%) determination results. Delivered dose, OD600 ~ 0.05 of E.大肠杆菌 and 3 μg mL −1 water-soluble fullerenol C60(OH)46. For the percentages alive and dead, p <0.001. *p value obtained using Student’s t test. Data are presented as mean ± SD (n =6)
图> 图>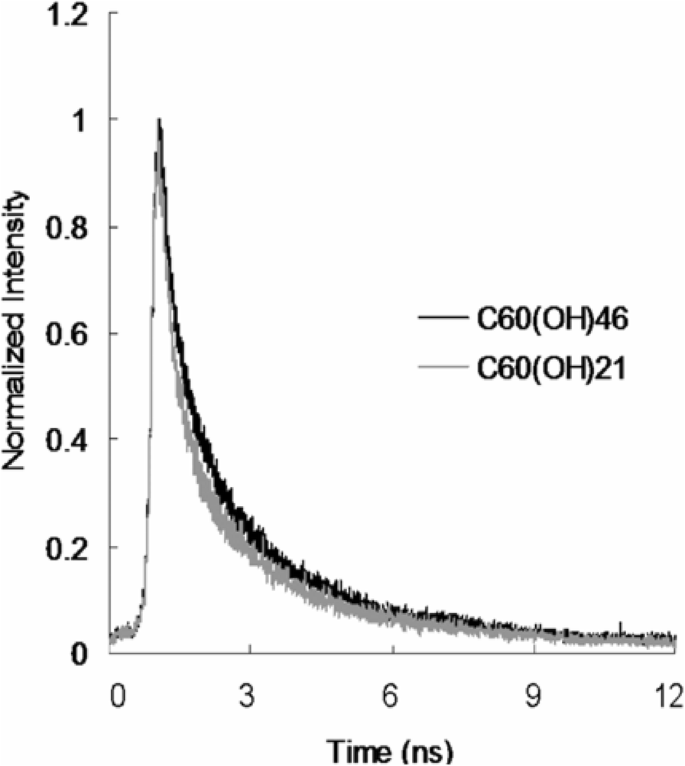
Time-resolved room-temperature PL decay profiles of material (98.56 nJ pixel −1 )。 Excitation wavelength, 760 nm. Delivered dose, OD600~ 0.05 of E.大肠杆菌 and 3 μg mL −1 材料。 Data are presented as means ± SD (n =6)
图> 图>Conclusions
This study revealed that a water-soluble fullerenol material with a higher composition of hydroxyl groups had superior photoproperties to those of a fullerenol material with a lower composition of hydroxyl groups; the superior photoproperties can be attributed to the reduced laser exposure and materials used for treatment. Furthermore, the water-soluble fullerenol with a higher composition of hydroxyl groups exhibited high TPA, a favorable absolute cross section for TPE, and high two-photon stability. Therefore, this fullerenol has potential as a two-photon PS in two-photon PDT coupled with TPE. This property is probably due to the presence of a hydroxyl group on the surface of the water-soluble fullerenol, which caused the nonradiative recombination of electron–hole pairs, leading to the inhibition of intrinsic state emission. Moreover, hydroxyl groups at the edge of the water-soluble fullerenol may have a high occupied molecular orbital; this may be ascribed to the strong orbital interaction between the hydroxyl groups, thereby increasing intersystem crossing (rather than fluorescence generation) efficiency and generating numerous material triplets with a high composition of hydroxyl groups. Therefore, the water-soluble fullerenol would have a high Φ Δ value and react with oxygen according to the Jablonski diagram. Consequently, two-photon PDT can be effectively performed using ultralow energy in an extremely short time. Accordingly, this efficient alternative approach to managing malignant species presents possibilities for future clinical applications.
数据和材料的可用性
All datasets are presented in the main paper.
缩写
- PS:
-
Photosensitizers
- QY:
-
Quantum yield
- PDT:
-
Photodynamic therapy
- UV–vis:
-
Ultraviolet–visible
- ROS:
-
Reactive oxygen species
- ψ Δ :
-
Singlet oxygen QY
- 近红外:
-
近红外
- TPE:
-
Two-photon excitation
- E.大肠杆菌 :
-
大肠杆菌
- HR-TEM:
-
High-resolution transmission electron microscopy
- DLS:
-
动态光散射
- FTIR:
-
Fourier-transform infrared
- XPS:
-
X射线光电子能谱
- FD:
-
Field desorption
- CFU:
-
Colony forming unit
- TSPP:
-
Meso -tetra(4-sulfonatophenyl)porphine dihydrochloride
- PL:
-
光致发光
- DMSO:
-
二甲亚砜
- OPE:
-
One-photon excitation
- TPA:
-
Two-photon absorption
- TPL:
-
Two-photon luminescence
- ti-sa:
-
Titanium-sapphire
- NA :
-
Numerical aperture
- FLIM:
-
Fluorescence lifetime imaging microscopy
- 1 O2:
-
Singlet oxygen
- SOSG:
-
Singlet Oxygen Sensor Green
- t -MVP:
-
Trans-1-(2′-methoxyvinyl)pyrene
- O2 . - :
-
Superoxide radical anion
- XTT:
-
2, 3-Bis (2-methoxy-4-nitro-5-sulfophenyl)-2H-tetrazolium-5-carboxanilide
- GSH:
-
Glutathione, γ -l-glutamyl-l-cysteinyl-glycine
- S1:
-
Singlet
- T1:
-
Triplet
- B. subtilis :
-
Bacillus subtilis
- GM:
-
Goeppert-Mayer
纳米材料
- 具有可控厚度的二硫化钼用于电催化析氢
- S、N 共掺杂石墨烯量子点/TiO2 复合材料用于高效光催化制氢
- 用贵金属纳米粒子装饰的电纺聚合物纳米纤维用于化学传感
- 具有分层多孔结构的单分散碳纳米球作为超级电容器的电极材料
- 探索 Zr-金属-有机框架作为制氢的高效光催化剂
- 用于高效光催化制氢的 ZnO@TiO2 空心球的分级异质结构
- 用于最大太阳能收集的高效且有效的 InP 纳米线设计
- 掺杂 Sb2O3 纳米晶体:一种用于有机降解的高效可见光催化剂
- 具有倒金字塔微结构的 20.19% 效率单晶硅太阳能电池的制造
- 制备具有可见光催化活性的 Cu2O-TiO2 NTA 异质结的简便方法
- Fox Chase 与 Rodon Group 合作开发用于 Temple Health 的 COVID 检测拭子
- 沃尔沃集团与英伟达合作开发自动驾驶卡车的人工智能平台